Trends in the Public Sector Adoption of Translational Research Approaches
HumanFirst Therapeutics LLC, Silver Spring, MD, USA
The past decade has witnessed strong growth in the adoption and funding of translational research strategies by the public sector [1]. This has been driven by an increasing awareness within the scientific community of the need to engage in the high-risk and consortium-driven steps required to move basic science discoveries toward the clinic. Early drug discovery activities, such as target discovery and pharmacological validation, are increasingly understood to be precompetitive, community-based efforts in which information sharing can accelerate the formation of collaborative alliances between the complementary capabilities of biology, chemistry, and informatics. Chemical biology approaches, in particular, have attained new prominence as a vital enabler of novel pharmacological investigation. These project-based team efforts allow, for example, preclinical testing and validation of a new therapeutic approach. This leverages investment in investigator-initiated research, and motivates directed preclinical development activities that aim to progress a therapy into the clinic. A model for the path of these activities, leading from lab bench to clinic, is shown in Figure 8.1. A more elaborate academic view of translational research process is described by Trochim et al. [2]. Both the National Institutes of Health (NIH) and nonprofit disease foundations are stakeholders in this process and have made efforts to support engagement of the scientific community in translational research.

Particular note will be made of best practices learned from translational research initiatives launched by the NIH. The NIH Molecular Libraries Initiative has been an early driver of work to ascribe function to new genomic information by building a compendium of molecular probes (pharmacological tools) that could be used by the scientific community to better understand and validate possible disease targets, pathways, and phenotypes. The molecular probes resulting from the chemical biology effort are beginning to drive preclinical proof-of-principle (POP) work aimed at validating strategies to therapeutically alter processes that lead to disease initiation or progression [3]. They can also be employed to identify similarities in the molecular pathology of diseases for which there was not previously a direct association. At the other end of the spectrum lies the NIH Clinical Translational Science Awards (CTSA) initiative,* a collaborative consortium of 60 medical research institutes with efforts centered on early investigative clinical trials, both in terms of clinical methods development and training, comparative effectiveness research, and human proof-of-concept (POC) testing of new therapeutic approaches [4–6].
The difficulty in connecting research success to clinical testing is often voiced as a prominent concern of the medical research community [7, 8]. Whereas a number of medical research institutes have robust and innovative research activity (supported primarily by NIH funding) and large clinical trials enterprises, it is rare for research discoveries to progress from bench to bedside within the walls of an institution. Key factors include funding, mentorship, and training, and the need to partner with external organizations (e.g., pharmaceutical companies, disease foundations), which have competencies that are generally missing from the academic environment. This chapter will examine several public efforts aimed at facilitating the migration of research innovation from bench-to-bedside (and POP-2-POC). This path typically requires a handoff of investigator-initiated activities at the lab bench to large-scale consortium efforts (e.g., high-throughput screening [HTS]), before returning to activities such as molecular probe optimization and preclinical experimentation that are executed by individual investigator teams or comparatively small collaborating teams.
The discussion will center on the first step in many translational efforts: the adaptation by investigators of lab-based assays for novel biological targets, pathways, and cellular phenotypes as a means of initiating a subsequent chemical biology effort to design molecular probes for use in investigative POP research. Initiatives to perform large-scale HTS of small molecule compound libraries using these assays, by the NIH Molecular Libraries Centers and by other Centers, are discussed elsewhere [9, 10]. A model NIH program to facilitate investigator-driven optimization of chemical leads coming from HTS will also be covered to illustrate unique issues encountered in assembling small multidisciplinary teams in an effective manner. Finally, mention will be made of a CTSA Public–Private Partnership efforts to identify, aggregate, and partner tools and know-how comprising a POP-2-POC toolkit for therapy development projects, via the open-access web resource CTSA•IP.*
Steps to Successful Assay Development for HTS
The NIH Molecular Libraries Assay Development for HTS Initiative began with the purpose of funding independent investigators to miniaturize and characterize biological assays that could be used in small molecule screening campaigns by the pilot phase Molecular Libraries Screening Center Network (MLSCN). The data would then be posted publically in PubChem.† The Initiative quickly expanded to encompass a broader scope and mandate of assembling complete assay projects that could be used for HTS and probe development in the Molecular Libraries Probe Production Centers Network (MLPCN).‡ In this section of the review, a Best Practices in Assay Development is discussed that has been developed and used to guide investigators seeking to prepare assay development proposals and forge working relationships with Molecular Libraries Centers. Many of these details are embedded in prior NIH Program Announcements for the Assay Development for HTS program,§ and in the Fast Track program guidance for the entry of completed HTS projects into the MLPCN.¶
The Assay Development for HTS program worked to adapt assays from the research programs of investigators for use in HTS and molecular probe design. Resources were provided to configure and characterize assays with the goal of their entering screening in the MLSCN (and MLPCN) within 12–18 months. A particular emphasis was given to the development of novel assays and assay technologies. The effort successfully assembled a portfolio of diverse small molecule screening projects well suited to HTS.
Principal technical challenges in the preparation of a biological assay for screening against a small molecule compound library such as the NIH Molecular Libraries Small Molecule Repository (MLSMR)* include the requirements for miniaturization, the availability of consistent and stable reagent preparations (e.g., cells, vectors, biochemical substrates), a low number of handling steps to accommodate full automation, and an assay readout with robust signal and low background. These are default minimum requirements for an HTS assay but are not sufficient to drive a screening project to successful completion.
Academic assay projects intending to advance to screening can often be open-ended, be performed with the intent of adapting the approach to the results of screening, and may lack a desired outcome, specified at the outset to guide the project. Played out in the context of a multidisciplinary screening center environment, which employs a team approach encompassing a number of skill sets and coordinated activities, open-ended projects can experience long delays as different methodologies are adopted, or new assays inserted to accommodate a change in goals. In addition, at the handoff of a project from the Investigator to the Center, it is necessary to establish the criteria guiding the selection and progression of screening positives (hits). Equally important is agreement on when a project should be stopped, with the understanding that HTS resources are typically constrained, and that early termination of a project not meeting its goals would allow centers to expedite new project efforts.
To resolve project start-up and decision-making issues, the Molecular Libraries Initiative adopted guidelines for interrogating the HTS-readiness of a project prior to its acceptance into the network. These Fast Track program guidelines were originally designed to allow NIH staff to evaluate Assay Development for HTS projects that had neared completion, and were later broadly applied to assay projects originating in diverse NIH-funded activities. The assay project guidelines “start at the end” by first asking the Investigator to visualize a successful outcome, and then describe the “critical path” of assays and compound selection criteria to be used at each step in the path. A simple model of a project’s critical path is illustrated in Figure 8.2.

Investigators are prompted to answer the following questions in a project proposal:
- What experiments (e.g., mechanism-of-action, POP) would be enabled by having a molecular probe?
- What features would this probe need to have in order for it to be useful (e.g., affinity, selectivity, cell penetrance, stability to metabolism)?
- What assays would be used to measure these probe attributes?
- In what order would you use these assays? What would the assay cutoff values need to be to select compounds in the test?
An evaluation using technical screening criteria is then employed to judge the robustness of the proposal and allow determination of the NIH institute-specific interest (basic science or disease relevance) in a project. A project ranking is accomplished using the following scorecard as guide:
- Feasibility of the proposed screening assays
- Completeness of the screening plan
- Availability of key reagents required for execution of the HTS project
- Completion of a pilot screen.*
- Current availability of molecular probes (pharmacological tools) to the proposed target or phenotype
- Intended experimental use of the probe to be developed, to include a description of values for the attributes that are required in a useful probe (e.g., affinity value as measured in the primary assay, fold-selectivity versus an undesired target interaction).
A proposal for an HTS assay needs to demonstrate that the assay can be further miniaturized and implemented in an automated screening center environment. The following detailed information is needed to describe the primary HTS assay:
- A description of reagents and readouts that can be translated to an automated HTS environment. Common assay readouts are fluorescence, luminescence, fluorescence resonance energy transfer (FRET), and bioluminescence resonance energy transfer (BRET). Absorbance, scintillation proximity assay (SPA) or biophysical readouts, and whole cell imaging are also used. Assays requiring only addition of reagents (e.g., “mix and measure”) are preferable, and steps such as centrifugation, filtration, and extraction are to be avoided in an automation environment.
- A detailed description of methods demonstrating highly robust and reproducible behavior in a 96-well or higher density format (e.g., 384- or 1536-well plates), or adaptability to a flow cytometry approach.
- Performance data for the primary assay, to include the following:
- A signal of sufficient intensity that can be easily measured (using, e.g., a microtiter plate of low volume).
- A signal-to-background ratio of at least 5 and a coefficient of variation (CV) below 10% (determined from measurements across an entire plate). These factors can be expressed individually and as the statistical parameter Z, which has an acceptable lower limit of 0.5 [11].
- Reproducible, dose-dependent responses in the primary assay to a small collection of pharmacologically active standards, reaction substrates, or inhibitors. Alternatively, data from pilot screening of a structurally diverse collection of small molecules could be used. The intent of this characterization is to demonstrate that the assay can detect interaction between a small molecule and the target.
- Control parameters, including time, temperature, and protein concentration dependencies. Additional information that should also be provided in a proposal includes tolerance to the effect of 0.1–1% dimethyl sulfoxide (DMSO), between-plate and day-to-day variations, and a determination of reagent stability to storage and assay conditions.
- A signal of sufficient intensity that can be easily measured (using, e.g., a microtiter plate of low volume).
The critical path of activities constituting a complete screening project plan includes the following: a primary HTS assay to identify compounds having activity toward the target, phenotype, or pathway that is the subject of molecular probe design; a secondary assay used to confirm the activity of the chemical hits and additionally provide detail about their activity; and counterscreening assays to test for the activity of hits at other targets. These assays can also be used to characterize additional hit attributes such as cellular penetrance or toxicity.
Information provided to describe the critical path assays should include the following:
- A description of secondary screens that can be used to confirm that the activity of compounds in the primary assay is reproducible and rule out artifacts (e.g., compound impurity or fluorescence interference of the test compound). This test can be performed using the primary assay to establish dose-dependent activity, or an assay to the target that employs a different measurement of activity. The secondary assay can also be used to rank order the potency or affinity of hits, and assess agonism or antagonism.
- A description of counterscreening assays allowing the prioritization of hits for further testing (e.g., on the basis of selectivity or potential toxicity). It is likely that several hundred or more active compounds may be identified in an HTS effort that employs a library of >105 compounds.
- Recommendations of appropriate test concentrations, together with concentration cutoffs to be used in selecting active compounds.
- Information describing reagent availability, equipment required for performing the assays, and estimated cost per well of an assay.
- Description of the throughput rate (compounds per day in the format provided), the current plate format (384-well, 96-well, cuvette), and the average assay cycle time (hours or days to complete an assay).
Last, it is worth noting that phenotypic assay projects represent an exception to the standard formatting of a critical path, as followed for molecular target assay projects. The goal of developing a probe in this instance often supports target discovery rather than target characterization efforts. Thus, successful hit deconvolution presents a challenge. Phenotypic assays entering the MLPCN for screening have, in general, used one of two approaches to verifying and sorting hits from the primary (phenotypic) HTS assay:
- The secondary and counterscreen assays used are alternative phenotypes. For instance, the goal may be to target cancer cells but not isogenic cells lacking oncogene [12].
- The characterization assays represent cellular signaling pathways in a cell that can be used to narrow the potential activity of a compound to a specific pathway or member of that pathway [13, 14].
Impact of the NIH Molecular Libraries Assay Development for HTS Initiative
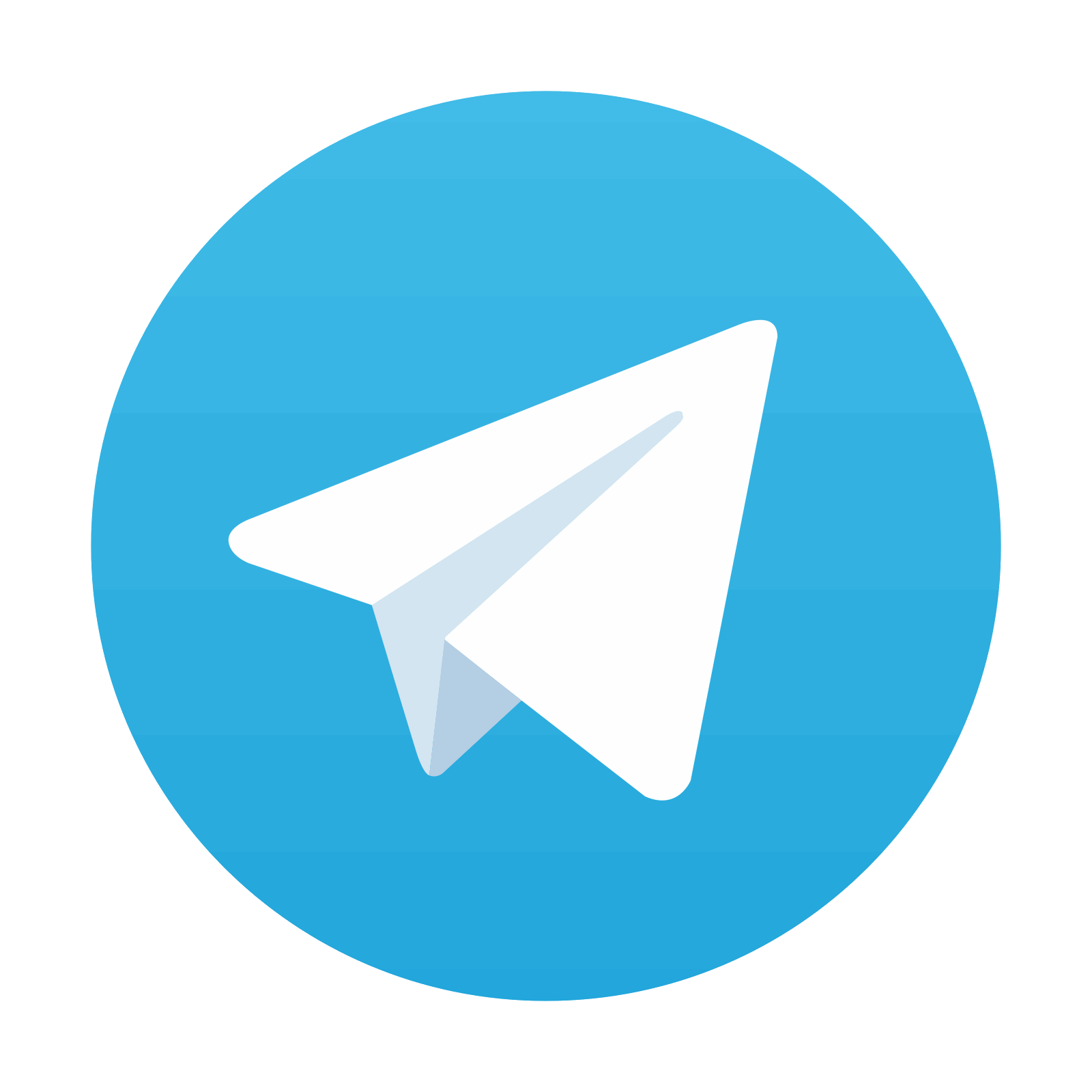
Stay updated, free articles. Join our Telegram channel

Full access? Get Clinical Tree
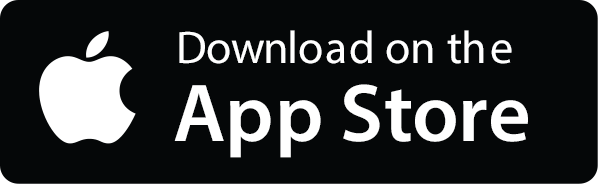
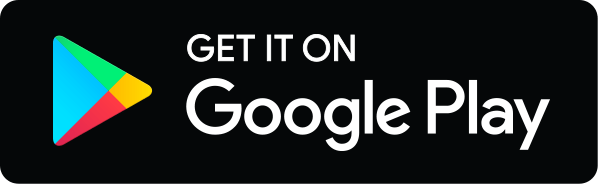