- More patients than ever before are being cured of their cancer or are having ongoing treatment to allow them to live with their cancer with good quality of life.
- Major advances have been made in the delivery of radiotherapy to minimize the damage to normal tissues.
- The increased understanding of tumor biology has resulted in many biologically targeted agents being available in the clinic.
- For a number of the common tumor types, genetic testing of the tumors of patients is now standard practice to define which targeted therapies they should receive.
- Consideration regarding the future cost of cancer therapy needs to be made to ensure that the complex therapies developed are not prohibitively expensive and therefore not financially viable.
Introduction
Chemotherapeutic agents exert their effect by killing cells that are rapidly dividing. The agents are therefore not tumor cell specific and cause their toxic effect by killing dividing normal cells (e.g. hair follicle cells and gastrointestinal (GI) mucosa). In recent years, our understanding of the molecular pathways controlling the growth of both normal and tumor cells has improved significantly. By exploiting the differences between normal and malignant cells, we can target pathways and receptors unique to the cancer cells, thus avoiding the indiscriminate universal killing of dividing cells of conventional cytotoxics. We are therefore entering the era of biologically targeted therapies in cancer treatment (Chapter 16).
Radiotherapy is the application of ionizing radiation to treat disease. Ionizing radiation is electro-magnetic radiation and elementary particles, which deposit energy in materials through the processes of excitation and ionization events. The forms of ionizing radiation in common use are photon beams (X-rays and gamma rays) and electrons (β particles). Radiotherapy has been in use for over 100 years, and currently over 60% of patients diagnosed with cancer will receive it at some time during their illness. There have been major technical advances in delivery of radiotherapy in recent years.
Hormones are thought to be etiological agents in at least half of the cancers diagnosed in developed countries. Common cancers, such as breast and prostate, often express receptors for sex hormones (e.g. female hormones such as estradiol or male hormones such as dihydrotestosterone). The growth of such cancers is at least in part dependent on hormonal stimulation. The aim of hormonal therapy is to withdraw the growth stimulus, either by reducing hormone production or by interfering with the receptor–ligand interaction or signaling associated with agonist activity. Subclasses of estrogen and progesterone receptors are of particular interest with regard to breast cancer, and androgen receptors are relevant to the treatment of prostate cancer.
Radiotherapy Physics
The biological effects of radiation are a result of absorption of energy from the radiation. Since absorbed dose is energy divided by mass, radiotherapy is prescribed in units of Grays, where one Gray = 1 joule of energy absorbed per kilogram of mass.
When photons pass through matter, they interact with that matter through a number of different physical processes. Low-energy photons (e.g. those used in diagnostic X-rays) interact with electrons which are bound within atoms, resulting in the photo-electric effect. This effect is highly dependent on atomic number, and therefore differentiates tissues such as bone very well from other tissues such as water. At higher photon energies, the photoelectric effect becomes less important, and photons tend to interact with unbound electrons within the matter. This physical process is called “Compton scatter.” For photon energy produced by linear accelerators (≥4 MeV), the effect of photoelectric absorption is negligible and other physical processes predominate.
External beam radiotherapy can be delivered using a number of different modalities:
In addition to external beam therapy, other modalities place the source of radiation internally:
Radiobiology
Ionizing radiation causes extensive cellular damage, principally via the formation of free radicals. Cells have a considerable capacity for repair, and cell survival depends on a number of factors (see Chapters 8 and 10). In an average cell, one Gray (Gy) causes damage to over 1000 bases in DNA, approximately 1000 single-strand breaks (SSBs) in DNA, and approximately 40 double-strand breaks (DSBs). DSBs in DNA are considered the most significant types of molecular damage, and correlate with the probability of cell survival. Typically, survival curves are continuously bending, with a slope that steepens as the dose increases (see Fig. 18.1). Mathematically, a continuously bending curve is most simply described by a linear quadratic equation of the form: surviving fraction = exponential (−α D – β D2), where D is the given single dose and α and β are parameters characteristic of the cells concerned. Since the ratio of α : β gives the relative importance of the linear dose term and the quadratic dose term for those cells, this ratio is used to characterize the radiosensitivity of a particular tissue type.
Figure 18.1 Survival curves for cells with different α : β ratios subjected to irradiation. The more steeply curving survival curve has a lower α : β ratio when fitted to the linear quadratic equation. Note log scale. SF = surviving fraction following irradiation.
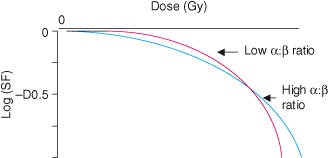
To improve the therapeutic ratio (the differential effect on malignant tissue compared to normal tissue), the total dose of radiotherapy can be divided into small daily amounts – “fractionation.” Fractionated radiotherapy inflicts more damage on tumor cells than on normal cells for a given dose of radiation. Moreover, cell and tissue kinetics usually favor recovery and repopulation of damaged normal tissue over tumor tissue (see Box 18.1). Although tumors are very diverse, the radiobiological properties of most tumors are similar to those of acute responding tissues, that is, a high α : β ratio results in moderate sensitivity to changes in fraction size and some dependence on the total treatment times. It has been shown that extending total treatment time or allowing significant gaps during treatment can decrease the efficacy of the radiotherapy.
The timing and severity of injury to normal tissue depend on the rate of turnover of the mature cells in the tissue. Epithelial and hematopoietic tissues have rapid turnover and are therefore susceptible to acute effects over days or weeks (Box 18.2). Tissues with slower turnover are susceptible to late effects, with a timescale of months or years. In fact, risks of major late effects to spinal cord, lung, liver, and kidney are often the dose-limiting factors in radiotherapy. Since stem cells of late-responding tissues have more a curved survival curve (i.e. a lower α : β ratio) than cells of acute-responding tissues, the late-responding tissues are particularly sensitive to changes in fraction size. The probability of “long-term toxicity” can therefore be diminished significantly by using small fractions of radiotherapy rather than larger fractions, but this consideration must be balanced against the effect of the overall treatment time on the tumor tissue.
Treatment Planning
Management plans are highly individualized and based on the nature of the cancer, the general health of the patient, and the goal of the therapy. Treatment planning for external-beam radiotherapy involves a number of steps, as shown in Fig. 18.2. The specification of a radiotherapy treatment will include the dose, the number of treatment fractions, and the volume that is to receive the dose. The gross tumor volume consists of all known macroscopic disease, demonstrated by either physical examination or imaging. The clinical target volume encompasses the gross tumor volume plus regions considered to harbor potential microscopic disease. The planning target volume provides a margin around the clinical target volume to allow for internal target motion (e.g. respiratory motion) and variations in treatment setup. Any nearby structures which may suffer from radiation damage are also defined as organs at risk. The challenge in defining the radiation beams which will be used for the treatment lies in maintaining dose coverage to the planning target volume, whilst ensuring doses to organs at risk are acceptable. These target volumes are shown in Fig. 18.3. Patient immobilization is used when highly sensitive structures are close to the target, for example spinal cord or brain. A thermoplastic shell is often used to immobilize the head and keep the neck position constant, with the advantages that the treatment fields can be drawn on the shell rather than the patient and the shell can be fixed to the radiation couch.
Figure 18.3 Schematic representation of “volumes” in radiotherapy. Gross tumor volume (GTV): demonstrable tumor; clinical target volume (CTV): GTV + suspected microscopic disease; planning target volume (PTV): CTV + margin for positional variation; OAR: organ at risk.
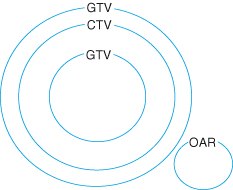
Recent Advances
Intensity-Modulated Radiotherapy (IMRT)
Better methods for delivering radiation allow the area of high dose to more closely conform to the planning target volume. In IMRT, the pattern of radiation from each beam is manipulated to give greater control over the dose in the patient. These patterns would be too complex to design manually, and these treatments are created by a computer process called “inverse planning.” With this technique, the defined volumes and specified acceptable dose levels are critical. IMRT has found widespread uptake in the treatment of prostate and head and neck cancers (Fig. 18.4).
Figure 18.4 Dose distribution using intensity-modulated radiotherapy (IMRT). This dose distribution is for a modern IMRT treatment of a head and neck tumor showing the concurrent delivery of a high tumorcidal dose to the primary tumor and a lower prophylactic dose to the at-risk lymph nodes. The dose to the spinal cord and the contralateral parotid gland are kept below the tolerance dose for late effects.
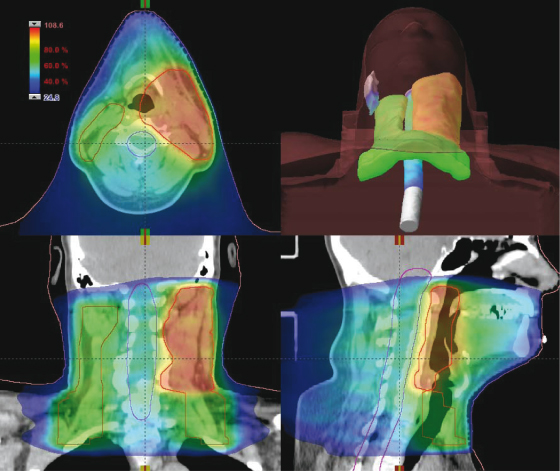
Rotational IMRT has demonstrated an even greater improvement in control over the dose distribution, where the pattern of radiation is varied as the beam rotates around the patient in a continuous sweep. Alternatively, tomotherapy machines use a narrow slit of radiation, which rotates around the patient as they pass though the center of the machine, analogous to a spiral CT scanner.
Image-Guided Radiotherapy (IGRT)
Imaging systems integrated into the treatment device allow the position of the target to be monitored and corrected on a daily basis. As well as ensuring the treatment is accurately delivered as specified, this should also allow for a reduction in the planning target volume margin and hence reduce the volume of tissue irradiated. A whole range of techniques are available, but the challenge remains the visualization of the actual tumor rather than surrounding bony anatomy. Several techniques involve the implantation of markers into the tumor.
Stereotactic Radiosurgery
Highly accurate immobilization and positioning devices, coupled with the precise delivery of multiple small radiation fields, allow tightly conformed doses of radiotherapy to be delivered as a single dose or as fractionated doses. Stereotactic radiosurgery is a well-established technique for the treatment of small malignant lesions in the brain and benign arterio-venous malformations, although lesions must meet stringent size criteria. Advances in extracranial stereotactic radiosurgery now allow similar high-dose treatments for lung and hepatobiliary tumors as well as metastases in lung, liver, and spine.
Proton Radiotherapy
The use of protons for radiotherapy can offer exquisite control over the dose distribution inside the patient. They are especially useful where the target is very close to critical structures and have an established role for neurological, pediatric, and ocular tumors. The immense devices that are used to produce proton beams, cyclotrons, are hugely expensive and can be funded at only a national level. In 2010 nearly 7000 patients received proton radiotherapy at one of around 30 centers worldwide. These low numbers often limit the application of protons to those cases with the greatest need. A number of research programs offer the promise of proton radiotherapy at greatly reduced cost, which would provide a huge advance for clinical radiotherapy.
Chemoradiation
The concomitant use of chemotherapy and radiotherapy is a curative modality in the treatment of anal, head and neck (H&N), and cervical cancer, and may be considered as an alternative to surgery. Although the concomitant use of chemotherapy with radiotherapy increases acute and late damage to normal tissues, it is an established standard of care for carcinoma of the cervix and anus. In squamous H&N cancer, meta-analyses have suggested a small but consistent survival benefit from the addition of chemotherapy to radical radiotherapy. The expanding use of chemoradiation is currently an exciting area of research, particularly the combination of newer chemotherapy drugs and biological therapies with radiotherapy in the treatment of GI cancers.
Rationale of Chemotherapy
Much has already been discussed in this book about drug development in cancer, in Chapters 16 and 17 in particular. To understand the rationale of cytotoxic chemotherapy, it is important to recognize the features of tumor growth (see Chapter 5). The factors responsible for determining the growth of a tumor include the cell-cycle time and growth fraction (i.e. the number of cells that are undergoing cell division and the number of cells). Also, the more cancer cells there are, the more likely that some will become resistant to chemotherapy and prevent cure. The asymmetric sigmoidal growth curve (“Gompertzian” growth curve) describes the natural history of tumor growth. By the time they are clinically apparent (around 109 cells or more), most tumors are in the relatively slow phase of growth (near the plateau of the Gompertzian growth curve). Unfortunately, this is the time that chemotherapeutic agents are least likely to prove effective. However, if the tumor can first be resected, then subsequent chemotherapy should be more effective as remaining cancer cells are now likely to be in logarithmic growth.
Surgery
Historically, surgery was the only treatment option for cancer, and in the modern era it often still offers the best chance of a cure. There are six main areas where surgery plays a fundamental role in the treatment of cancer (Box 18.3).
More recently, laparoscopic or “keyhole” surgery has become an attractive proposition in cancer patients. There is good evidence that, with appropriate training, complete clearance of tumors can be achieved using this approach with the added benefit of reduced morbidity and hospital stay for patients.
Combination Chemotherapy
In modern oncological practice, chemotherapeutic agents are used in combination rather than as sequential single therapies. For a particular tumor type, only drugs which are known to be effective as single agents should be incorporated, and ideally these drugs should be used at their optimal dose and schedule. It is imperative that the combination of drugs chosen should have a minimal overlap in toxicity to achieve this goal. The treatment should be delivered on an intermittent basis, with the shortest possible time between treatments that allows the recovery of the most sensitive normal tissue (e.g. the bone marrow or gut). This intermittent scheduling takes advantage of the observation that tumor cells recover slower from cytotoxic damage than do their normal counterparts. Thus, with each sequential cycle, the tumor population should be increasingly depleted, leaving the normal population relatively unaffected. Wherever possible, it is preferable to use drugs with known synergistic killing effects, for example the combination of oxaliplatin and 5-fluorouracil (5-FU). Another possibility is to use a combination of drugs which can kill cancer cells at different stages of the cell cycle (i.e. they are cell-cycle dependent).
Some regimens use alternating cycles of different drug combinations. One of the main principles behind this practice is the Goldie–Coldman hypothesis. The model predicted that the mutation of tumor cells to drug resistance occurred at a rate proportional to the genetic instability of a particular tumor. Therefore, the probability that a tumor at diagnosis would contain resistant clones would be proportional to both the tumor size and the inherent mutation rate. Theoretically, tumors should initially respond to treatment but recur as resistant clones expand. Therefore, the Goldie–Coldman hypothesis predicted that drug resistance could be present even with small tumors and the maximum chance of cure would occur if all available effective drugs were used simultaneously. This was the basis behind the development of using combinations of effective, non-cross-resistant drugs in alternating cycles.
In the 1980s, Norton and Day reviewed the Goldie–Coldman hypothesis. In their overview, they predicted that if two agents were used and if the less effective one was given first, the overall outcome would be superior to initial administration of the more effective agent. This has been the rationale for using sequential therapies in the treatment of some tumor types, for example breast cancer.
Indications for Chemotherapy
Chemotherapy is a systemic treatment most commonly used to treat advanced cancer. In a limited number of situations, it may be used as the primary or sole modality of treatment (e.g. chemosensitive cancers such as leukemia or teratoma). For the majority of the common solid tumors, chemotherapy is frequently used to reduce the volume of disease and palliate symptoms caused by the cancer. A further indication for chemotherapy is to use it as an adjuvant after the primary tumor has been controlled by either surgery or radiotherapy. Here the rationale is to eradicate subclinical micrometastatic disease and reduce the risk of recurrence. An example where adjuvant chemotherapy is extremely useful is in patients with breast cancer who have nodal involvement.
Neoadjuvant chemotherapy is being used increasingly to de-bulk or downstage primary tumors prior to the definitive treatment, which could be either surgery or radiotherapy. An example of this would be to use neoadjuvant chemotherapy to downstage a large breast primary tumor, so that conservative breast surgery could be offered rather than radical mastectomy.
In the majority of cases, chemotherapy is used systemically either intravenously or orally. It may, however, be administered regionally, for example intrathecally in the treatment of hematological malignancies with a high likelihood of central nervous system involvement. It can also be infused directly into the blood supply of liver metastases from colorectal cancer, or into the peritoneum in the treatment of advanced ovarian cancer.
Classification of Cytotoxic Drugs
Historically, anticancer agents have been classified according to the phase of the cell cycle during which they work. With the increasing number and complexity of chemotherapeutic agents, classification according to their mode of action is far more relevant.
Alkylating Agents
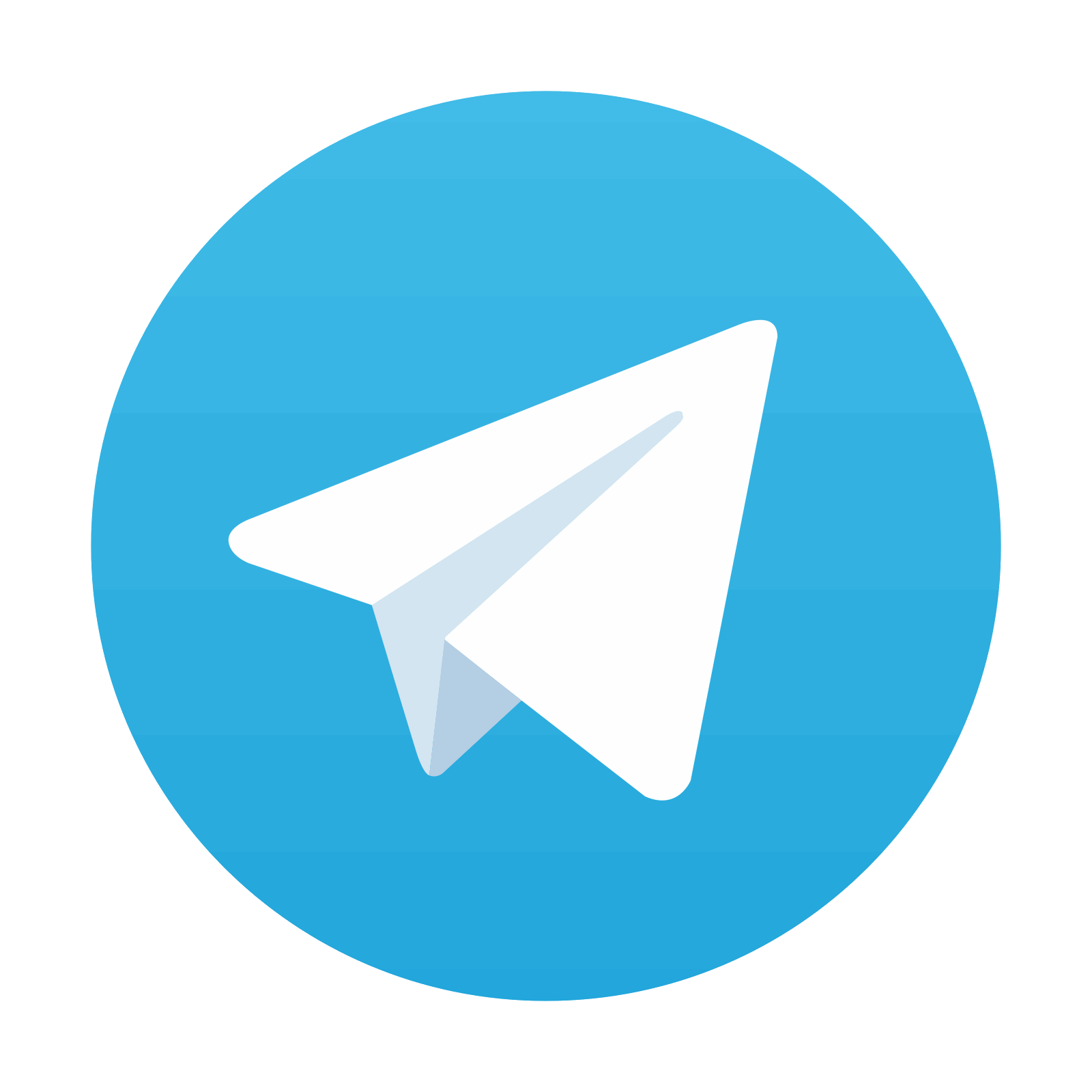
Stay updated, free articles. Join our Telegram channel

Full access? Get Clinical Tree
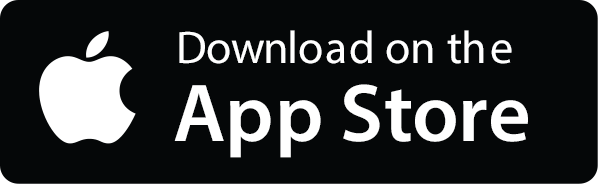
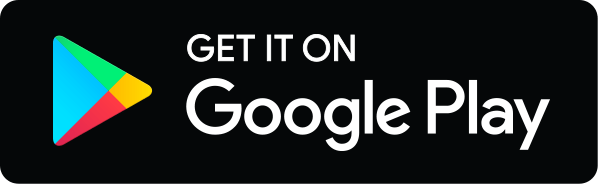