22 Transmission electron microscopy
Tissue preparation for transmission electron microscopy
The basic preparation methods for routine TEM are provided in this chapter. More detailed discussions of these, plus alternative and specialized procedures, can be found elsewhere (Glauert 1972; Robards & Wilson 1993; Allen & Lawrence 1994; Glauert & Lewis 1998; Hayat 2000). A flow chart summarizing the steps required for preparing the basic range of diagnostic TEM specimens is given in Figure 22.1.
Fixation
The fixatives used in TEM generally comprise a fixing agent in buffer (to maintain pH) and, if necessary, with various additives to control osmolarity and ionic composition. Other factors that affect fixation include fixative concentration and temperature, and the duration of fixation. The standard protocol involves primary fixation with an aldehyde (usually glutaraldehyde) to stabilize proteins, followed by secondary fixation in osmium tetroxide to retain lipids (Hayat 1981).
Duration of fixation
The time required for optimal fixation depends on a range of factors, including the type of tissue, the size of the sample, and the type of fixative and buffer system used. In most circumstances immersion of 0.5–1.0 mm3 blocks of tissue in 2.5% glutaraldehyde fixative for 2–6 hours is sufficient. It is recommended that punch biopsies of skin taken for cerebral autosomal dominant arteriopathy with subcortical infarcts and leukoencephalopathy (CADASIL) screening should be fixed overnight (to ensure adequate preservation), particularly if they are left whole (i.e., not dissected) and sent to a distant laboratory for processing and screening. Secondary fixation in 1% osmium tetroxide for 60–90 minutes is usually effective; much longer times are required if osmium tetroxide is the primary fixative. The use of microwave irradiation can accelerate fixation times in aldehyde fixative to as little as 5–10 seconds (Leong 1994), after which the sample may be stored in buffer or processed immediately.
Buffers
Fixatives are normally buffered within the range of pH 7.2–7.6 (Robinson & Gray 1996). Ideally the osmolarity and ionic composition of the buffer should mimic that of the tissue being fixed. In general practice this is not a major requirement but, if required, 300–330 mOsm (the osmolarity equivalent to that of plasma or slightly hypertonic) is suitable for most circumstances. Non-ionic molecules such as glucose, sucrose or dextran are used to adjust tonicity as these will not influence the ionic constitution of the buffer. The addition of various salts, particularly calcium and magnesium, is thought to improve tissue preservation, possibly by stabilizing membranes (Hayat 1981). This is unlikely to have a major effect in routine diagnostic applications.
Phosphate buffers
Phosphate buffers (Gomori 1955) have the disadvantage of being good growth media for molds and other microorganisms. Additionally, most metal ions form insoluble phosphates, which restricts the use of this buffer (the phosphates of sodium, potassium and ammonium are soluble). Nevertheless, phosphate buffers are the buffer of choice as they are non-toxic and work well with most tissues.
Alternative buffers
Other buffers that have been recommended for use in TEM include cacodylate (Plumel 1948; Sabatini et al. 1963), HEPES (N-2-hydroxyethylpiperazine-N′-2-ethanesulfonic acid), MOPS (3-(N-morpholino) propanesulfonic acid) and PIPES (piperazine-N,N′– bis2-ethanesulfonic acid) (Good et al. 1966; Good & Izawa 1972; Massie et al. 1972; Salema & Brandão 1973; Ferguson et al. 1980).
Aldehyde fixatives
Glutaraldehyde
Although glutaraldehyde is the most widely used primary fixative in TEM, its fixation reactions are not well understood. The most important reaction of glutaraldehyde, that of stabilizing proteins, is thought to occur via a cross-linking mechanism involving the amino groups of lysine and other amino acids through the formation of pyridine intermediaries. Lipids and most phospholipids (those that do not contain free amino groups) are not fixed and will be extracted during subsequent processing without secondary fixation (Hayat 2000).
Aldehyde combinations
The use of an aldehyde mixture has been proposed as a way of offsetting the disadvantages of glutaraldehyde (a slow penetration rate) and formaldehyde (less stable fixation) when applied individually (Karnovsky 1965).
Paraformaldehyde (2%) and glutaraldehyde (2.5%) fixative (buffered) (based on Glauert 1972; Karnovsky 1965)
Stock reagents
0.2 M buffer, pH 7.4 (phosphate, cacodylate) | 50 ml |
Paraformaldehyde | 2.0 g |
25% aqueous glutaraldehyde | 10 ml |
Distilled/deionized water to | 100 ml |
Method
1. Completely dissolve paraformaldehyde in buffer using heat and with continuous stirring. It may be necessary to add a few drops of 1.0 M sodium hydroxide to clarify the solution.
2. Cool the solution rapidly under running water.
3. Add aqueous glutaraldehyde. Check the pH of the mixture and adjust if necessary to pH 7.4.
Osmium tetroxide
The use of osmium tetroxide fixation to preserve lipids is fundamental to electron microscopy (Palade 1952; Millonig & Marinozzi 1968). While primary fixation in osmium tetroxide is effective, its extremely slow penetration rate can give rise to autolytic changes. For this reason osmium tetroxide is almost always used as a secondary fixative (termed ‘post-fixation’) after primary fixation in aldehyde. The penetration rate of osmium tetroxide is also higher in stabilized tissue, such that immersion for 60–90 minutes is usually sufficient for most specimens.
Osmium tetroxide fixative (2% aqueous)
Stock reagent
Osmium tetroxide | 1.0 g |
Distilled/deionized water | 50 ml |
Method
1. Clean, then score the glass ampoule with a diamond pencil and place in a dark-glass storage bottle.
2. Break the ampoule with a glass rod and add water. It may take 24 hours or longer for the osmium tetroxide to dissolve completely.
3. Prepared solutions may be stored for short periods at room temperature in the dark in a well-sealed bottle (double wrap the bottle in aluminum foil); for long periods store at 4°C. All osmium solutions should be stored inside a second closed container to prevent the leakage of osmium fumes. Aqueous osmium solutions that are prepared and stored in a clean container should last for ~1 year; solutions in buffer may only last a few days before they deteriorate.
4. For a 1% working solution combine 1:1 with water or buffer.
Epoxy resins
Epoxy resins have been the embedding medium of choice in TEM since their introduction in the mid-1950s (Glauert et al. 1956). These resins contain a characteristic chemical group in which an oxygen and two carbon atoms bond to form a three-membered ring (‘epoxide’). Cross-linking between these groups creates a three-dimensional polymer of great mechanical strength. The polymerization process generates very little shrinkage (usually less than 2%) and, once complete, is permanent. As well as their properties of uniform polymerization and low shrinkage, epoxy resins also preserve tissue ultrastructure, are stable in the electron beam, section easily and are readily available.
Examples of widely used epoxy resin composites include Araldite (Glauert & Glauert 1958), Epon (Luft 1961) and Spurr’s resin (Spurr 1969). Although the original product names Araldite and Epon refer to epoxy resins developed by the CIBA Chemical Company and Shell Chemical Company, respectively, these terms are now in general use. Araldite polymers are preferred as these react with a higher degree of cross-linking and are the most stable.
Occupational exposure to epoxy resins is a common cause of allergic contact dermatitis (Kanerva et al. 1989; Jolanki et al. 1990). These agents are also probable carcinogens, primary irritants and systemically toxic (Causton 1981). Spurr’s resin in particular is toxic and should be handled with great care (Ringo et al. 1982).
Acrylic resins
The main commercial acrylic resins are LR White and LR Gold and the Lowicryl series (K4M, K11M, HM20 and HM23). Each of these can be used for low-temperature dehydration and embedding to reduce the heat damage from exothermic polymerization and extraction by solvents and resin components (Acetarin et al. 1986; Newman and Hobot 1987, 1993). These characteristics make several forms of acrylic resin ideally suited to electron immunogold labeling (Stirling 1994) and enzyme cytochemical studies.
Tissue processing schedules
Manual tissue processing is best performed by keeping the tissue sample in the same vial throughout, and using a fine pipette to change solutions. When processing multiple samples, take care not to cross-contaminate specimens – use separate pipettes. All vials must be clearly labeled and labels must be ‘solvent-proof’. It is advantageous to agitate tissue specimens throughout the processing cycle to enhance reagent permeation. A protocol for the routine processing of solid tissue samples is given in Table 22.1.
Table 22.1 Standard processing schedule for solid tissue cut into 1 mm3 blocks (each step is performed at room temperature unless stated otherwise)
Primary fixation | 2.5% glutaraldehyde in 0.1 M phosphate buffer | 2–24 hours (room temperature or 4°C) |
Wash | 0.1 M phosphate buffer | 2 × 10 minutes on rotator |
Wash | Distilled water | 2 × 10 minutes |
En bloc staining (optional) | 2% aqueous uranyl acetate | 20 minutes |
Dehydration | 70% ethanol | 10 minutes on rotator* |
90% ethanol | 10 minutes on rotator | |
95% ethanol | 10 minutes on rotator | |
100% ethanol | 15 minutes on rotator | |
Dry absolute ethanol | 2 × 20 minutes on rotator | |
Transition solvent (clearing) | 1,2-epoxypropane | 2 × 15 minutes on rotator |
Infiltration | 50 : 50, clearant : resin# | 1 hour |
25 : 75, clearant : resin | 1 hour | |
Resin only | 1–24 hours (with vacuum to remove bubbles) | |
Embedding | Fresh resin in embedding capsules | 12–24 hours at 60–70°C |
* Tissues may be stored at this stage.
# As batches may vary, resin should be prepared in accordance with manufacturer’s instructions.
Procedures for other tissue samples
Cell suspensions or particulate matter
Cell suspensions (such as fine needle biopsy aspirates, bone marrow specimens or cytology samples) or particulate materials (including fluid aspirates, tissue fragments or products and specimens for the assessment of ciliary structures) are best embedded in a protein support medium before processing. Plasma, agar or bovine serum albumen (BSA) can be used. The addition of tannic acid (Hayat 1993) during the preparation of ciliary specimens gives improved visualization of axonemal components (Sturgess & Turner 1984; Glauert & Lewis 1998). The tannic acid is thought to act as a fixative and also a mordant, facilitating the binding of heavy metal stains (Hayat 2000). Double en bloc staining with uranyl acetate and lead aspartate may also improve the visibility of dynein arms (Rippstein et al. 1987).
Preparing particulate specimens
Method
1. Centrifuge the material in buffer in a plastic centrifuge tube to form a loose pellet.
2. Discard supernatant and resuspend the material in glutaraldehyde fixative at room temperature for a minimum of 1 hour.
3. Centrifuge the material and carefully discard the supernatant.
4. Wash the specimen by resuspending it in buffer for 10–15 minutes.
5. Centrifuge the material to form a loose pellet.
6. Discard supernatant and introduce 0.5 ml of 15% aqueous BSA. Resuspend the specimen and allow it to infiltrate for a minimum of 1 hour.
7. Centrifuge the material and discard most of the supernatant, leaving sufficient to cover the pellet to a depth of approximately 1 mm.
8. Introduce an equal volume of glutaraldehyde fixative to form a layer above the BSA. Allow material to solidify for 2–24 hours.
9. Remove the material (this is most easily achieved by cutting away the plastic centrifuge tube) and divide into small portions.
10. Wash in four changes of buffer, each for 5 minutes (for ciliary biopsies only, incubate for 15 minutes in buffered tannic acid solution, then wash in four changes of buffer, each for 5 minutes, before proceeding to step 11).
11. Postfix in 1% aqueous osmium tetroxide and process as normal.
Material embedded in paraffin/cell smears
Reprocessing paraffin-embedded material
Method
1. Remove the area of interest from the block, taking care not to damage the tissue.
2. Dewax the specimen by passing through several changes of xylene. The time required depends on the size of the sample but should be at least 1 hour. A minimum of three changes is recommended.
3. Rehydrate the material in a graded ethanol series.
4. Wash in water, postfix in osmium tetroxide and process as routine specimen (see above).
Pop-off technique for slide-mounted sections (after Bretschneider et al.1981)
If additional fixation is required
2. Rehydrate the tissue in a graded ethanol series.
3. Wash in buffer and fix the tissue in glutaraldehyde fixative for 15–20 minutes.
4. Wash in buffer and postfix in 1% osmium tetroxide for 20–30 minutes.
5. Wash in buffer or distilled water, and then cover with 2% uranyl acetate for 15 minutes.
6. Dehydrate the tissue by passing the slide through 70%, 90%, 95%, 100% and super dry ethanol for 5 minutes in each stage.
7. Dip the slide into propylene oxide for 5 minutes. The tissue should not be allowed to dry.
8. Cover the tissue with a 2 : 1 mixture of propylene oxide and epoxy resin for 5–15 minutes. The tissue should not be allowed to dry.
9. Cover the tissue with a 1 : 2 mixture of propylene oxide and epoxy resin for 5–15 minutes. The tissue should not be allowed to dry.
10. Cover the tissue with neat epoxy resin for 5–15 minutes.
11. Drain off surplus resin mixture. Invert a freshly filled (to overflowing) embedding capsule over the section and press onto the slide.
12. Incubate the slide and capsule at 60°C for 24 hours for polymerization to occur.
13. Remove the slide and, while still warm, separate the capsule and the newly embedded tissue from the glass slide.
If additional fixation is not required
2. Dip the slide in equal parts of propylene oxide and xylene, then into propylene oxide for 5–10 minutes. The tissue should not be allowed to dry.
3. Cover the tissue with a 2 : 1 mixture of propylene oxide and epoxy resin for 5–15 minutes. The tissue should not be allowed to dry.
4. Cover the tissue with a 1 : 2 mixture of propylene oxide and epoxy resin for 5–15 minutes. The tissue should not be allowed to dry.
5. Cover the tissue with neat epoxy resin for 5–15 minutes.
6. Drain off surplus resin mixture. Invert a freshly filled (to overflowing) embedding capsule over the section and press onto the slide.
7. Incubate the slide and capsule at 60°C for 24 hours for polymerization to occur.
8. Remove the slide and, while still warm, separate the capsule and the newly embedded tissue from the glass slide.
Ultramicrotomy
Glass knives
Knives should always be inspected before use. If the knife edge is correctly formed, when it is observed face-on it should be straight and even but with a small glass spur on the top right-hand end (Fig. 22.2).
In ultramicrotomy, thin sections are floated out for collection as they are cut. This requires a small trough to be attached directly to the knife. Pre-formed plastic or metal troughs that can be fitted to the back of the knife are commercially available. These must be sealed with molten dental wax or nail varnish after attachment but they are expedient and simple to use. An alternative approach is to prepare a trough using self-adhesive PVC insulating tape. The lower edge of the trough so formed is then sealed with molten dental wax (Fig. 22.3).
Block trimming
Once polymerized, blocks must be cleared of excess resin to expose the tissue for sectioning. At the completion of this process the trimmed area should resemble a flat-topped pyramid with a square or trapezium-shaped face (Fig. 22.4).
Semi-thin sections
Commonly, semi-thin sections are cut at between 0.5 and 1.0 µm from trimmed or partly trimmed blocks using the ultramicrotome and a glass knife. Sections can be cut dry (using a slow cutting speed) and picked up with forceps or directly into the flotation bath attached to the knife. Sections are transferred to a drop of water on a glass microscope slide and dried on a hot plate at 70–80°C. Semi-thin sections can be examined using phase contrast or be stained and viewed by bright-field microscopy. Various cationic dyes, including methylene blue, azure B (Richardson et al. 1960) and crystal violet, can be used for this purpose, although the most common is toluidine blue (with borax). All are applied at high alkaline pH and with heat to facilitate penetration of the resin.
Collection of sections
Ultra-thin sections are mounted onto specimen grids for viewing. Grids measure 3.05 mm in diameter and are made of conductive material, commonly copper, nickel or gold, although silver, palladium, molybdenum, aluminum, titanium, stainless steel, nylon-carbon and combination varieties are available. A large range of patterns and mesh sizes are available (Fig. 22.5), with 200 square mesh being commonly used, although slotted, parallel bar and hexagonal patterns are also standard. As electrons cannot pass through the metal grid bars, the choice of grid becomes a compromise between support for the sections (better with grids of smaller mesh size) and the relative proportion of exposed section (better with grids of larger mesh size). The latter provides a large area of section for viewing but with less stability.
Support films
Electron-transparent plastic films prepared from collodion, Formvar or Butvar are commonly used. There are many methods for applying plastic films, with one of the simpler being illustrated in Figure 22.6. The major problem with using plastic films is that the conductive properties of the grid become compromised. Re-instating the thermal and electrical properties is usually achieved by adding a 5–10 nm layer of carbon in a sputter coater or vacuum-evaporating unit.
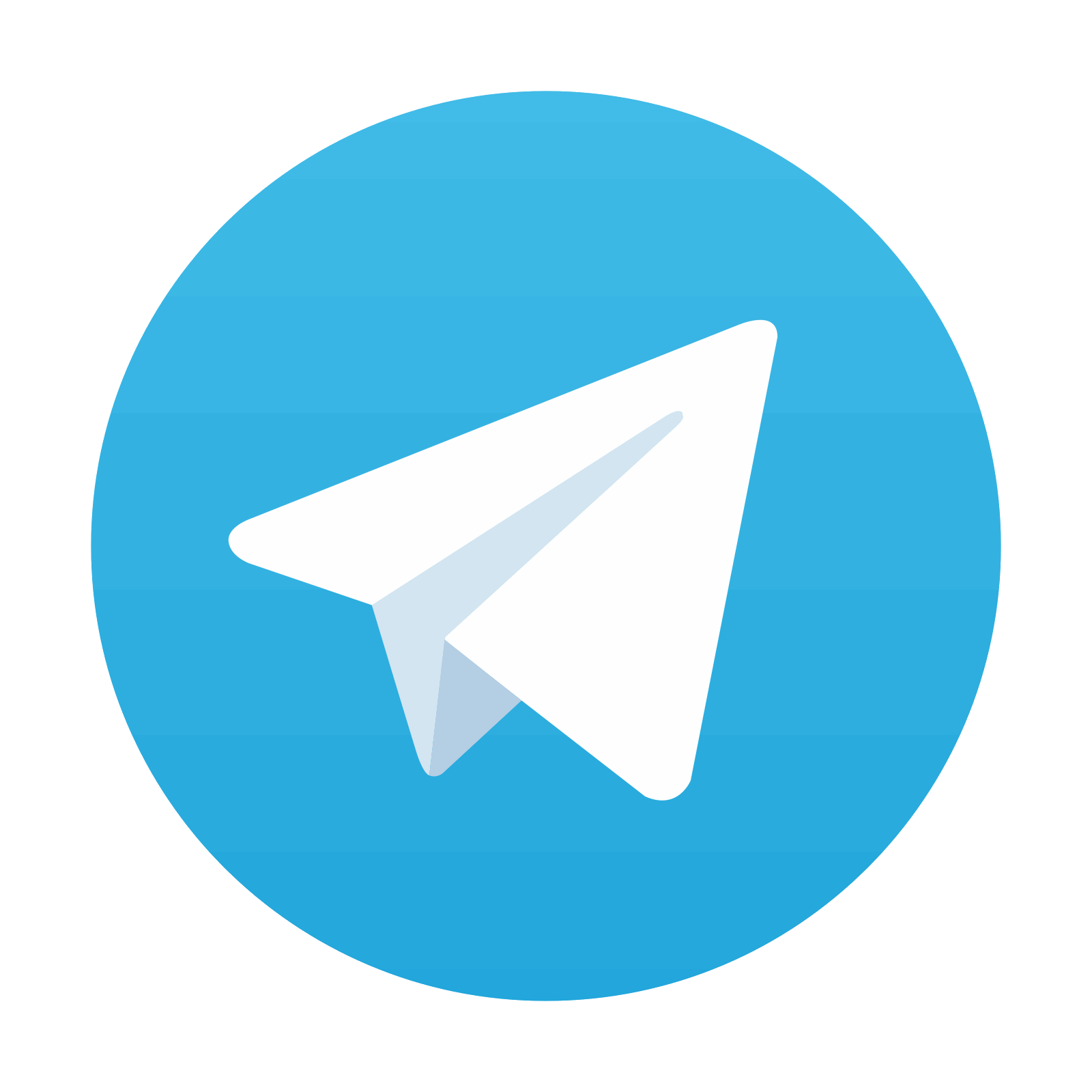
Stay updated, free articles. Join our Telegram channel

Full access? Get Clinical Tree
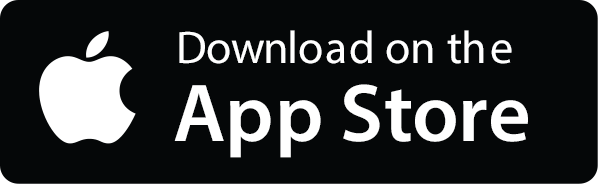
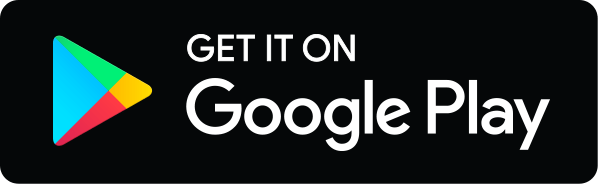