CONTENTS
9.1.1 Anatomy and Physiology of the Skin: Implications for Transdermal Drug Delivery
9.1.2 Advantages of the Transdermal Route for Drug Delivery
9.1.3 Limiting Factors for Transdermal Drug Delivery
9.2 Mechanical and Biological Properties of the Principal Skin Layers
9.2.1 Stratum Corneum: The Key Mechanical Barrier
9.2.2 Viable Epidermis and Dermis
9.3 Approaches to Transdermal Drug Delivery
9.3.1 Passive Approaches Relying on Diffusion
9.3.1.1 Transdermal Therapeutic Systems
9.3.2 Active Approaches Requiring Application of Energy
9.3.3 Physical Approaches That Breach the Stratum Corneum
9.3.3.1 Intradermal Injections
9.3.3.4 Microneedles, Microprojections, and Arrays Thereof
9.3.3.5 Nanopatch™: A Case Study
Transdermal drug delivery is an emerging field with key applications in local delivery of molecules to local tissue sites, through to systemic delivery applications under sustained-release conditions. Historically, transdermal delivery has been limited to molecules that fit a narrow physicochemical profile (low molecular weight, adequate solubility in both oil and water, high partition coefficient); however, the range of deliverable drugs is rapidly expanding on all fronts, thanks to advances primarily in (1) enhancing the permeability of the outermost skin layer (stratum corneum [SC]), (2) increasing the driving force for drug transport across the SC, (3) physical approaches that bypass the SC altogether, and (4) novel combinations of these methods. With ≈20 small molecule drug delivery systems (DDS) currently FDA-approved involving transdermal approaches, based on current trials in small animals and humans, it is likely that delivery of genetic and cellular therapies, vaccines, therapeutic proteins, and nanoparticle-encapsulated systems will be available in the near future.
9.1.1 ANATOMY AND PHYSIOLOGY OF THE SKIN: IMPLICATIONS FOR TRANSDERMAL DRUG DELIVERY
The skin is the largest organ of the human body (1.5–2.5 m2 surface area); its structure is described in Chapter 4 (Section 4.5.1 and Figure 4.6). It is composed of three principal skin layers: the SC, 10–20 μm; the viable epidermis (VE), 50–100 μm; and the dermis, 1–2 mm. The dermis is highly vascularized, while the SC/VE is not, yet capillary loops in the reticular dermis provide a route for nutrient supply and waste removal from the live cells in the VE. Traveling deeper into the dermis, hypodermis, and subcutaneous tissue, the blood vessels become larger and less dense, draining into the superficial venous plexus and the deep venous plexus. Stem and progenitor cells, located at the dermoepidermal junction, are responsible for skin renewal, continually pushing dead and dying cells into the SC and regenerating the underlying viable tissue.
The SC is the outermost layer, composed of corneocytes arranged in a “bricks and mortar” structure; it comprises the major physical barrier function of the skin (Figure 9.1). The corneocytes are terminally differentiated and flattened keratinocytes, rich in keratin filaments and embedded in a dense matrix of proteins to restrict transport of large molecules into the epithelial tissues beneath. The physicochemical properties of this layer are primarily responsible for limiting the free diffusion of molecules into the skin to those species <500 Da (approximately), which are moderately soluble in both water and oil phases.
The VE, i.e., the layers of the epidermis deep to the SC, is primarily composed of a densely packed layer of keratinocytes (≈95%) interspersed with small numbers of highly specialized cell types, including Langerhans cells (3%–5%; dendritic cells) and melanocytes (≈8%; melanin production to prevent UVB-associated damage) (Figure 9.1). Langerhans cells are potent antigen-presenting cells (APCs) and are thought to be key mediators of the improved immune responses observed in some transdermal vaccination techniques (see Section 9.3.3).
The dermis is a highly hydrated tissue composed largely of a collagenous extracellular matrix, containing a capillary network and a relatively lower density of cells, primarily fibroblasts and mast cells. Dermal dendritic cells (dDCs) have relatively recently been identified as a distinct APC population native to this layer, and their principal functions are still being determined, along with their interactions with other cells, tissue layers, and other components of the immune system. The vasculature of the dermis provides routes for systemic drug delivery, via circulating blood or lymphatic fluid.
FIGURE 9.1 Epidermal cell layers. (Designua/Shutterstock.com.)
9.1.2 ADVANTAGES OF THE TRANSDERMAL ROUTE FOR DRUG DEliVERY
In general terms, transdermal delivery methods are considered to be more acceptable to patients in comparison to more invasive delivery routes, including needle/syringe methods. This is because transdermal methods are relatively noninvasive, do not necessarily require trained health staff for administration, and “access” to viable delivery sites is rarely a concern due to the high number of available skin sites. Importantly, a number of transdermal techniques also allow the user or medical practitioner to control release rates into the tissue, from rapid delivery applications (e.g., local anesthetics) through to sustained-release periods (up to 1 week, e.g., pain medications).
For the delivery of drugs to local skin epithelial tissues, transdermal methods can clearly target the tissue of interest without off-target systemic effects. Transdermal delivery also has advantages for systemic delivery applications due to direct and/or indirect uptake of drugs from skin tissue fluid into the blood or lymphatic circulation, respectively. This potentially avoids first-pass liver metabolism, thereby requiring lower initial concentrations for therapeutic effects. Controlled-release strategies can also be used to control the drug delivery rate and pharmacodynamic profile. Furthermore, in the last decade or so, a clear narrative is emerging that transdermal methods provide unique access to a unique population of immunologically sensitive cells, which can be exploited for immunotherapeutic and vaccine applications.
9.1.3 LIMITING FACTORS FOR TRANSDERMAL DRUG DELIVERY
The key limitation in transdermal drug delivery, particularly for diffusion-based mechanisms, is that efficient delivery is limited to those compounds that possess the physicochemical properties that favor transepithelial transport. These properties are described in detail in Chapter 4 (Section 4.3.4); to summarize here for the transdermal route, this refers to low-molecular-weight (<500 Da approximately) compounds, with high partition coefficients and moderate solubility in both oil and water phases. This is due primarily to the physicochemical properties of the SC, which are crucial to maintain the skin’s barrier function. To overcome this limitation, a range of methods have been developed to enhance diffusion through the SC, or breach this outer layer completely, to gain direct access to the epithelial tissues underneath.
The other limitation, again particularly related to diffusion-based methods, is that attempts to increase delivery rates through the SC (direct breaching, enhanced permeability, etc.) are generally accompanied by local tissue irritation and/or inflammation. This has been problematic for the health-care providers, FDA, and patients, although it is becoming apparent that a minor degree of local irritation may enhance some therapeutic strategies, especially those that target the immune system.
9.2 MECHANICAL AND BIOLOGICAL PROPERTIES OF THE PRINCIPAL SKIN LAYERS
9.2.1 STRATUM CORNEUM: THE KEY MECHANICAL BARRIER
The SC is a semipermeable barrier that, owing to its variable mechanical properties, is challenging to breach in a minimally invasive manner, to target the underlying viable skin strata (whether it is the VE, dermis, or a combination of both). Mechanically, the SC is classified as a bioviscoelastic solid and shows highly variable properties. Obvious differences include the huge variation in thickness and composition with the skin site and the age of an individual. However, there are more subtle and equally important variations in SC properties to consider when configuring targeting methods.
For example, the SC mechanical breaking stress is strongly influenced by the ambient humidity/moisture content—the relative humidity range from 0% to 100% results in a decrease in excised human SC breaking stress from 22.5 to 3.2 MPa. Similarly, an increase in ambient temperature also results in an SC breaking stress decrease by an order of magnitude. More recently, with indentation studies using small probes (diameters of 2 and 5 μm) fitted to a nanoindenter (Kendall et al. 2007), we have found more complexity and variation in key SC, and underlying VE, mechanical properties. This work was then extended, first by moving the probes into the dermis (Crichton et al. 2011) and then also with more comprehensive measurements including a larger range of probe sizes (0.5–40 μm; Crichton et al. 2013).
Specifically, we found:
• As the probe radius is decreased, the elastic modulus increases (Figure 9.2a). Indeed, taking into consideration the work of others (e.g., Boyer et al. 2009), we found that decreasing the probe radius by three orders of magnitude (i.e., from 1000 to 1 μm, approximately), the corresponding elastic modulus also increased by three orders of magnitude (from 104 to 107 MPa).
• Significant differences in instantaneous elastic modulus between skin strata, also influenced by probe size (Figure 9.2b). In general, for a given probe size, the elastic modulus decreased from the SC to the VE, before increasing to the highest level in the dermis.
These and other sources of variability in the SC mechanical properties present challenges and fresh opportunities in configuring approaches to breach the SC in a minimally invasive manner and effectively deliver drugs/vaccines to the underlying layers. The other interesting point to note about the SC is that 0.1% of its area is comprised of appendages (hair follicle, sebaceous glands, and sweat ducts) that can provide “shunt” pathways for ions or polar molecules that cannot otherwise passively diffuse through the barrier. Indeed, since these units protrude into the dermis, they are served by capillary loops, which can serve to transport delivered cargoes into the systemic circulation. However, due to the very low surface area available at the skin surface, this route may only be applicable to drugs that require only minute quantities to generate the desired therapeutic response (e.g., gene therapies, vaccines).
9.2.2 VIABLE EPIDERMIS AND DERMIS
In the VE, the skin has evolved a highly competent immunological function, with an abundance of Langerhans cells (500–1000 cells mm−2), often serving as the first line of defense against many pathogens. As one example, Langerhans cells (Figure 9.1) are extremely effective APCs, responsible for the uptake and processing of foreign materials in order to generate an effective immune response. Such cells are reported to be up to 1000-fold more effective than keratinocytes, fibroblasts, and myoblasts at eliciting a variety of immune responses (Banchereau and Steinman 1998). Similarly, there are many types of APCs within the dermis that also serve important roles in immu-nological function.
The “target tissue” for most transdermal delivery approaches is the highly vascularized and immunologically rich dermal layer. In humans, the blood vessel density varies from 20 to 40 cm-2
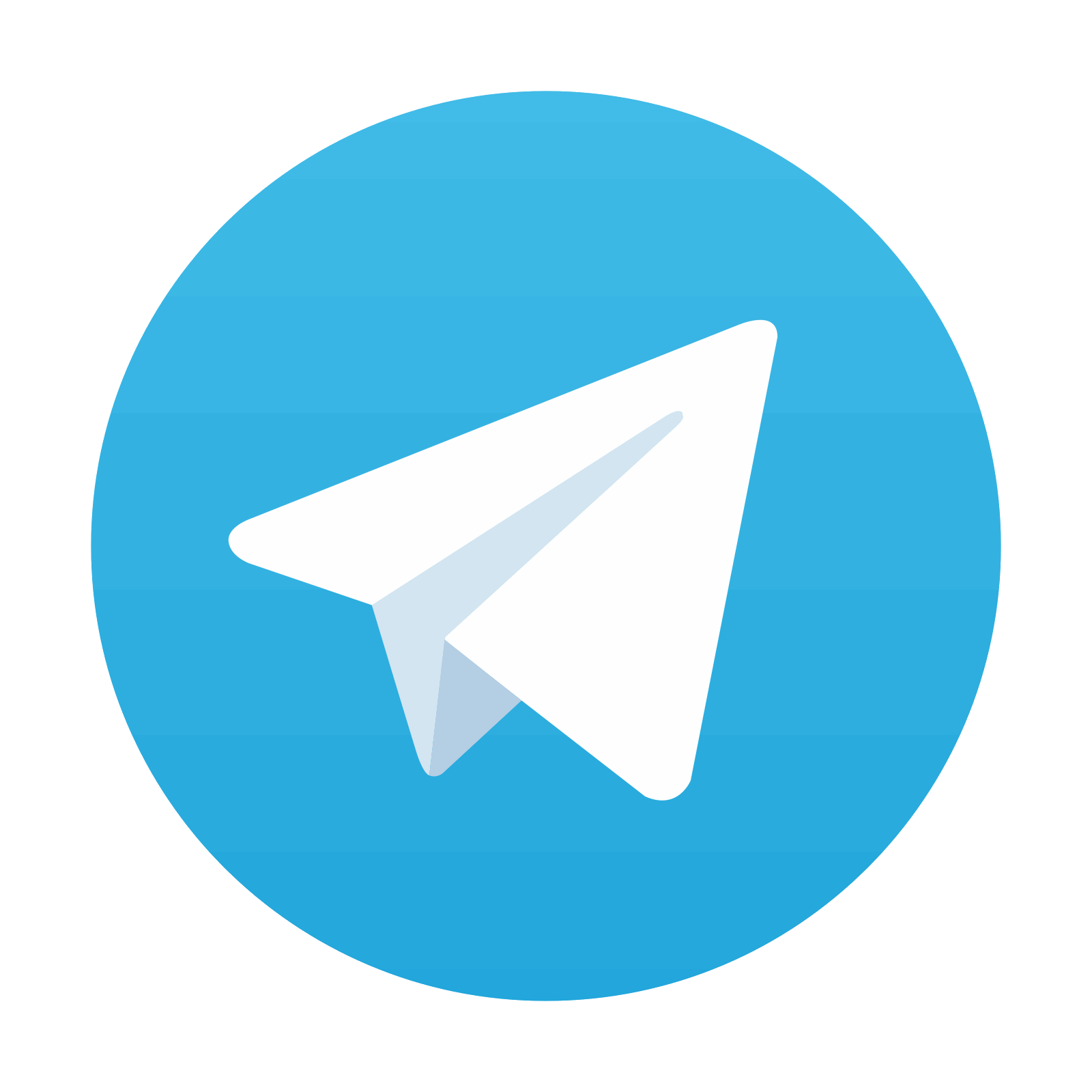
Stay updated, free articles. Join our Telegram channel

Full access? Get Clinical Tree
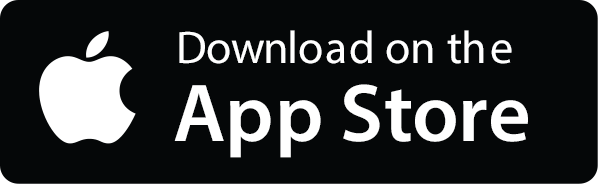
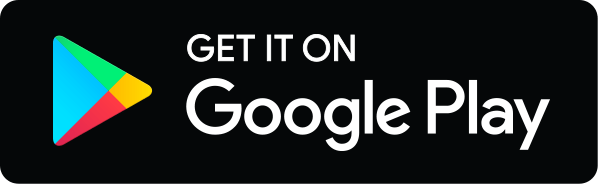