Trace Elements1
Curtis D. Eckhert
1Abbreviations: AI, adequate intake; As, arsenic; AsO2–, arsenite; ATPase, adenosine triphosphatase; B, boron; Ca2+, calcium; cADPR, cyclic adenosine diphosphate ribose; CO2, carbon dioxide; Cr, chromium; DARP, dissimilatory arsenate-reducing prokaryote; DRI, dietary reference intake; FAD, flavin adenine dinucleotide; FDA, Food and Drug Administration; GTF, glucose tolerance factor; Mo, molybdenum; NAD, nicotinamide adenine dinucleotide; Ni, nickel; PO3–, phosphite; Si, silicon; TPN, total parenteral nutrition; UL, tolerable upper intake level; V, vanadium.
Elements ingested in milligrams or less per day are referred to as trace elements (1). Chemists originally used the term trace to indicate that concentrations were lower than the detectable limits of the analytic procedure in some of their samples. Statistical analysis cannot use words, so the practice was changed by replacing “trace” with an estimated number, often the midpoint been the lowest detectable limit and zero.
Trace elements enter the food chain from soil, water, and atmospheric particles derived from weathered geologic formations and volcanic eruptions. Two concepts are important when considering the essentiality of a trace element metals. First, evolving biologic systems were based on geochemistry and aquatic chemistry, which used metals and metalloids to perform catalytic, structural, and signaling functions. In metal-poor environments such as the ocean, organisms survive because many functions can be maintained using different metals with similar ionic radii and electronic structures. The second concept is chemical reactivity. In living systems, reactive metal atoms are not “free,” but rather are stabilized by coordinate bonds to functional groups of amino acids in energetically strained (entatic) catalytic sites of proteins or are bound to ligands such as nucleotides and tetrapyrroles (2). In metalloenzymes, the strained state of metal coordination geometry stores most of the energy required to reach the critical high-energy transition state of the enzymesubstrate complex, so only small geometric changes are required to produce the activation energy needed to initiate enzymatic catalysis. One of the coordination sites on the metal atom is open for substrate binding and, in the relaxed state, binds an easily replaced ligand such as water (H2O). The reactivity of a metal confined in this manner performs an essential biologic function, but at concentrations that exceed the capacity to coordinate or bind metal atoms, the same reactivity can damage neighboring molecules. Thus, at low levels of intake, the benefit of a trace element metal may be relative to the availability of other elements, but at high intakes, the probability of toxicity approaches certainty.
This chapter addresses several of the trace elements: arsenic (As), boron (B), chromium (Cr), molybdenum (Mo), nickel (Ni), silicon (Si), and vanadium (V). These elements are present in tissues at concentrations in the range of micrograms per kilogram and have been reported to alter some biologic process in some species. The elements Cr, Mo, Ni, and V are metals, whereas As, B, and Si are metalloids with properties of both metals and nonmetals. The term essential has two parts: essentiality of the biologic function and the requirement of a specific element to achieve that function. B and Mo are the only trace elements covered in this chapter that are essential for plants and whose concentration in plant foods is determined by local soil and water concentrations, as well as homeostatic mechanisms of the plant. Mo is essential for human health, and B is essential for lower vertebrates and beneficial for humans. The elements As and B are unique in that both have been proposed to have important roles in the origin of life. Evidence suggests that the other trace elements are beneficial to health under specific conditions. The benefits of these other trace elements to humans may not be unique but can be achieved using other elements or molecules.
ARSENIC
Historical Overview
As has been used as a poison for thousands of years. The ancient Syrians used inorganic salts of As as agricultural pesticides (3), and today organic arsenics such as roxarsone (4-hydroxy-3-nitrophenyl arsenic acid) are used to prevent coccidiosis in swine and improve the growth of poultry. As trioxide was such an effective human poison during the Middle Ages; it was referred to as “inheritance powder” (4). One of the curious events of European history involved the metabolism of As. William Morris (1834 to 1896) was a member of the family that owned the largest As mine in Europe. The mine polluted the ecology of Devon, England and caused pocked skin and lung disease in the local population (5). To divorce himself from the mine, William sold his share and used the proceeds to manufacture expensive wallpaper colored with the dye Scheele’s green, which contained copper arsenite. Fungi in the wallpaper paste methylated the As salt into toxic trimethyl arsine. This volatile substance rose to high concentrations in the poorly ventilated rooms of the time. This is presumed to be the source of the high As levels in the hair of exiled Napoleon Bonaparte (6).
Terminology, Chemistry, Metabolic Roles, Interactions with Other Compounds, and Basic Importance in Normal Functions
As is widely distributed in nature in association with ores of metals such as copper, lead, and gold (3, 7). It is a metalloid existing in four oxidation states: As(−III), As(0),
As(III), and As(V). The predominant form of inorganic As in aqueous and aerobic environments is arsenate (As[V] as H2AsO4– and HAsO42-), whereas in anoxic environments, arsenite (As[III] as H3AsO30 and H2AsO3–) predominates. Adsorption of arsenate on the surface of minerals such as ferrihydrite and alumina constrains its hydrologic mobility. The most common form of arsenite (AsO2–) is less strongly adsorbed to minerals, so its oxyanion is more mobile in environmental water (8). As has not been shown to be required for any physiologic functions in animals or humans, but deficiencies have been reported to result in myocardial damage. Its principal importance in the diet is as a toxin that can induce damage to the nervous and cardiovascular systems and increase the risk of cancer of the skin, lung, and bladder.
As(III), and As(V). The predominant form of inorganic As in aqueous and aerobic environments is arsenate (As[V] as H2AsO4– and HAsO42-), whereas in anoxic environments, arsenite (As[III] as H3AsO30 and H2AsO3–) predominates. Adsorption of arsenate on the surface of minerals such as ferrihydrite and alumina constrains its hydrologic mobility. The most common form of arsenite (AsO2–) is less strongly adsorbed to minerals, so its oxyanion is more mobile in environmental water (8). As has not been shown to be required for any physiologic functions in animals or humans, but deficiencies have been reported to result in myocardial damage. Its principal importance in the diet is as a toxin that can induce damage to the nervous and cardiovascular systems and increase the risk of cancer of the skin, lung, and bladder.
Dietary Sources
The total dietary intake of As from food is approximately 50 µg of As/day, of which 10 µg is inorganic. Less than 4 µg/day is derived from drinking water (9). Salt water fish contain the highest concentration of As (1662 ng/g) as arsenobetaine, a nontoxic organic form. Cereals and bakery products provide approximately 23.5 ng/g, and fats and oils contain 19 ng/g (10). The major contributors of inorganic As are rice, flour, spinach, and grape juice (11). As intakes in North America range from 0.5 to 0.81 µg/kg/day, with a median intake of 2.0 to 2.9 and 1.7 to 2.1 µg/day for men and women, respectively (12). The concentration in human milk ranges from 0.2 to 6 µg/kg wet weight. Drinking water is the primary source of inorganic As(III) and As(V).
Recommended Dietary Allowances
The Food and Nutrition Board of the Institute of Medicine has not established a dietary reference intake (DRI) or a tolerable upper intake level (UL) for dietary As (Table 16.1).
Sites of Intestinal Absorption, Blood Transport, and Intracellular Forms
Soluble forms of ingested As are readily absorbed from water (90%) and food (60% to 70%) by the human gastrointestinal tract (13, 14). Less soluble arsenosugars occur in plant products such as seaweed and are poorly absorbed (15). The proportion of inhaled As absorbed ranges from 30% to 34% (16). As bound to the skin is slowly released into the circulation (17). As is cleared from the blood in humans, although some remains bound to the cysteine residues of hemoglobin (18). It is methylated in the liver using S-adenosylmethionine as the methyl donor to methylarsonic acid and dimethylarsinic acid (19). Arsenics containing As(III) are the preferred substrates for enzymatically catalyzed methylation. Inorganic and organic As(V) is first reduced to As(III) by glutathione or other thiols and then is methylated and cycled between the As(V) and As(III) oxidation states to form dimethylated products,
TABLE 16.1 RECOMMENDED DIETARY ALLOWANCES AND UPPER LIMITS | |||||||||||||||||||||||||||||||||||||||||||||||||||||||||||||||||||||||||||||||||||||||||||||||||||||||||||||||||||||||||||||||||||||||||||||||||||||||||||||||||||||
---|---|---|---|---|---|---|---|---|---|---|---|---|---|---|---|---|---|---|---|---|---|---|---|---|---|---|---|---|---|---|---|---|---|---|---|---|---|---|---|---|---|---|---|---|---|---|---|---|---|---|---|---|---|---|---|---|---|---|---|---|---|---|---|---|---|---|---|---|---|---|---|---|---|---|---|---|---|---|---|---|---|---|---|---|---|---|---|---|---|---|---|---|---|---|---|---|---|---|---|---|---|---|---|---|---|---|---|---|---|---|---|---|---|---|---|---|---|---|---|---|---|---|---|---|---|---|---|---|---|---|---|---|---|---|---|---|---|---|---|---|---|---|---|---|---|---|---|---|---|---|---|---|---|---|---|---|---|---|---|---|---|---|---|---|---|
|
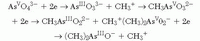
The mammalian enzyme responsible for catalyzing the transfer of the methyl group from S-adenosyl-L-methionine to trivalent and dimethylated arsenics is S-adenosyl-L-methionine: As(III) methyltransferase (19). Methylation efficiency in humans decreases when concentrations are high (20), and when the liver’s methylating capacity is exceeded, inorganic As accumulates in soft tissues. Pretreatment of cells with small amounts of As over prolonged periods increases the methylating efficiency and thereby decreases the risk of toxicity.
Tissue accumulation is influenced by methyltransferases, and polymorphisms of these enzymes may explain the variability in individual risk for As toxicity (19). As accumulates in the liver, kidney, muscles, heart, spleen, pancreas, lungs, and brain (21). Following low-level exposure to inorganic As, its methylated metabolites are
rapidly excreted in urine with small amounts of inorganic As eliminated in feces, sweat, skin desquamation, hair, and nails (22). The relative proportions of urinary As metabolites are 40% to 60% dimethylarsinic acid, 20% to 25% inorganic As, and 15% to 25% methylarsinic acid (23). One study, in which a single intravenous injection of radiolabeled trivalent inorganic As(III) was administered to human volunteers, showed that most of the As(III) was removed by urinary excretion within 2 days, and a small amount of excretion continued during the subsequent 2 weeks. The biologic half-life of As from fish is estimated to be less than 20 hours, with total clearance occurring over 48 hours. Blood concentrations may appear normal while levels in the urine remain elevated.
rapidly excreted in urine with small amounts of inorganic As eliminated in feces, sweat, skin desquamation, hair, and nails (22). The relative proportions of urinary As metabolites are 40% to 60% dimethylarsinic acid, 20% to 25% inorganic As, and 15% to 25% methylarsinic acid (23). One study, in which a single intravenous injection of radiolabeled trivalent inorganic As(III) was administered to human volunteers, showed that most of the As(III) was removed by urinary excretion within 2 days, and a small amount of excretion continued during the subsequent 2 weeks. The biologic half-life of As from fish is estimated to be less than 20 hours, with total clearance occurring over 48 hours. Blood concentrations may appear normal while levels in the urine remain elevated.
Functions in Metabolism and Biology
Bacteria isolated from mud taken from Mono Lake, California were able to grow using AsO3– in place of phosphite (PO3–). This finding suggests that life using AsO3– in place of PO3– was important in the origin of life on Earth and may exist on other planets (24).
A group of bacteria called dissimilatory arsenatereducing prokaryotes (DARPs) uses As(V) as a nutrient (7). DARPs occur in anoxic environments, in the gastrointestinal tracts of animals, and in the subsurface aquifer sediments of Bangladesh (7, 25). DARPs use As in respiration by linking the oxidation of lactate to the reduction of As(V) to As(III). No unique biologic functions have been discovered for As in vertebrates.
Assessment of Nutrient Status
Methods are not available to assess nutritional status, but blood levels of As are affected by the status of folate. Methylation of ingested inorganic As to monomethylarsonic and dimethylarsinic acids requires folate-dependent one-carbon metabolism and facilitates urinary As elimination. Gamble et al (26) used folic acid supplementation (400 µg/day) to reduce total blood As concentrations from a preintervention mean ± SE of 9.86 ± 0.62 µg/L to 8.20 ± 0.50 µg/L after the intervention with folic acid (p < .0001). A nonsignificant decline from 9.59 ± 0.63 µg/L to 9.14 ± 0.61 µg/L occurred in individuals receiving a placebo (p = .10) (26).
Specific Causes and Manifestations of Deficiency and Excess
Dabeka (10) reported symptoms associated with low dietary As intake in goats, miniature pigs, and rats. Myocardial damage was observed in lactating goats with evidence of mitochondrial membrane damage. Other manifestations include reduced growth, impaired fertility, and increased perinatal mortality. Deficiency symptoms depend on available methylating capacity (25).
The toxicity of As is based on the ability of As(III) to react with the sulfhydryl groups in proteins, thus leading to inactivation of enzymes (27). Mitochondria are the primary cellular targets of As(III), and they are where it accumulates, uncouples oxidative phosphorylation, and reduces the synthesis of adenosine triphosphate (ATP). As is also a cocarcinogen with ultraviolet radiation (20). The underlying mechanism may be inhibition of DNA repair by AsO2– following ultraviolet damage (28). Methylation of As competes for S-adenosylmethionine and leads to hypomethylation of DNA and potential damage (29). Acute As poisoning causes an acute paralytic syndrome characterized by cardiovascular collapse and loss of brain function resulting from necrosis of white and gray matter secondary to vasodilation (30, 31). The symptoms of As toxicity are dose dependent and include encephalopathy, gastrointestinal symptoms, skin pigmentation and dermatitis, peripheral vascular disease and neuropathy, genotoxicity, and cancer. Acute ingestion of 1 mg/kg/day of inorganic As causes anemia and hepatotoxicity. Ingestion of 10 mg/kg/day or more can result in encephalopathy and gastrointestinal disturbances. Long-term ingestion of 10 µg/kg/day in drinking water can produce arsenicism. This is an occlusive peripheral vascular disease, commonly referred to as black foot disease, in which, in extreme cases, feet turn black and develop gangrene. The US Environmental Protection Agency’s maximum contaminant level (MCL) for drinking water As is 10 µg/L (16).
Long-term ingestion of low levels of inorganic As occurs over an extensive region of Southern Asia and increases the risk of cancers of the skin, bladder, and lung (32). As poisoning is a problem in Bangladesh and West Bengal, India on a scale never before encountered for a natural or synthetic toxic substance (33, 34). The problem is a consequence of attempts to meet the water demand for the large population. In the 1970s, The United Nations Children’s Fund (UNICEF) and other relief international agencies drilled 6 to 10 million shallow drinking water wells to bypass sewage-tainted surface waters contaminated with cholera. The sediments in the area contained As adsorbed on the surface of iron oxides. By 1998, 61% of the shallow wells were found to be contaminated with As, thus exposing millions of people to high levels, with 200,000 reported cases of arsenicosis. The release of As from the minerals may have been initiated by the reduction of iron oxide coating on sand grains in the sediment by DARPs, inorganic carbon from peat, and methane (34).
BORON
Historical Overview
B was identified as an essential plant nutrient in 1923 (35), but it took 73 years to discover that borate esters are required to hold the cell wall scaffolding together under the enormous pressures required for cell elongation (36). B is also required for flowering and seed formation and is added to fertilizers, but deficiencies remain a major cause
of crop failure throughout the world. Fungi synthesize antibiotics that contain a single B atom in their structure, and bacteria synthesize and release autoinducer AI-2, a quorum-sensing molecule with a single B atom (37).
of crop failure throughout the world. Fungi synthesize antibiotics that contain a single B atom in their structure, and bacteria synthesize and release autoinducer AI-2, a quorum-sensing molecule with a single B atom (37).
In the search for B function, Hunt and Nielsen (38) employed a nutrient stress model. These investigators showed that B was beneficial for bone development in birds and mammals stressed with a combined deficiency of B along with calcium (Ca2+), vitamin D, or magnesium. Penland (39) determined that the major change in humans subjected to 62 days of B deprivation was a deficit in executive brain function. Eckhert (40) determined that B was required even in the absence of stress by showing that the growth of embryonic rainbow trout embryos (Oncorhynchus mykiss) increased in a dose-dependent manner with increasing boric acid. Further evidence for its essentiality in vertebrates was obtained in a study showing that B deficiency disrupted cleavage of zebrafish zygotes. B-deficient zygotes failed to cleave properly into two cells, and from two cells to the four-cell stage, and this was reversible by repletion with boric acid (41). These observations were reinforced by studies showing B was also required for the morphogenesis of frog (Xenopus) embryos (42). The major symptom of B deficiency in adult zebrafish was retinal degeneration, an observation that reinforced Penland’s observation that B was important for the nervous system (43).
Terminology, Chemistry, Metabolic Roles, Interactions with Other Compounds, and Basic Importance in Normal Functions
B was formed along with hydrogen, carbon, nitrogen, and oxygen during the nucleosynthesis of low-weight elements following the Big Bang (44). Borates from meteorites could stabilize glyceraldehyde, thus allowing it to combine with enediolate and stabilize ribose in the interstellar environment (45). This postulated role, in the transition between interstellar chemistry and the RNA world, has placed B center stage at the origin of life.
B, atomic number 5, has an atomic weight of 10.81 and exists as a mixture of stable isotopes 10B and 11B with respective abundances of 19.8% and 80.2% in the natural environment. The principal geologic forms of borate include the following: tincal (borax), Na2B4O7.10H2O; kernite (borax pentahydrate), Na2[B4O5(OH)4].2H2O; colemanite, Ca[B3O4(OH)3].H2O; and ulexite, NaCa[B5O6(OH)6].5H2O (44). B is a metalloid with an electronic structure of 1s22s2p and oxidation state of +3. The chemistry of B in nature is dominated by its affinity for oxygen (46). Three (trigonal) covalent bonds with oxygen form boric acid and four (tetrahedral) borates. B has a strong tendency to form a fourth bond to complete the octet of valence electrons in molecules such as halides. Soluble forms of B include boric acid B(OH)3 and the monovalent anion B(OH)4–, with the predominate form dependent on the pH of the solvent. Boric acid is a weak Lewis acid with a negative logarithm of the constant for the ionization equilibrium (pKa) of 9.2. The structures of borate minerals contain trigonal BO3 or tetrahedral BO4 units forming large B-oxygen anions. Boric acid is the major form of B in physiologic fluids at concentrations with reported ranges from 2 to 100 µM B, but usually between 2 and 10 µM B. Boric acid and borate form complexes with cis-diol groups on the five-carbon sugars apiose in plants and ribose in animals. Physiologic concentrations of boric acid have been shown to modulate the release of endoplasmic reticular Ca2+ stores, one of the major processes by which cells control intracellular events in response to changes in the environment. At millimolar concentrations, boric acid inhibits serine proteases including prostate serum antigen (8).
Dietary Sources
All foods made from plants and their byproducts contain B as an essential structural component of cell walls; seeds, nuts, and vegetables contain a higher concentration than fruits and grains. Furthermore, the B content of foods reflects local soil and water conditions in which they were grown (46). The major contributors of B to the diet are those associated with the Mediterranean diet and include apples, avocados, legumes, dates, prunes, nuts, wine, whole grain breads, tomato sauces, and potatoes (47, 48).
Because diets in industrialized countries contain various plant products, the major contributors to the diet often represent less than 10% of total B intake. In developing countries, however, it is skewed to one food. For example, the top contributor in Germany is wine (15%); in Kenya, it is maize (35%); in South Korea, it is rice (6%); in Mexico, it is tortillas (56%); and in the United States, it is coffee (6%) (48, 49).
Recommended Dietary Allowances
The Food and Nutrition Board has not established a DRI for B. The ULs for different age groups are as follows: 3 mg B/day, 1 to 3 years of age; 6 mg B/day, 4 to 8 years; 11 mg B/day, 9 to 13 years, 17 mg B/day, 14 to 18 years; and 20 mg/day for pregnant and lactating women more than 19 years old and all adults (11) (see Table 16.1).
Sites of Intestinal Absorption, Blood Transport, and Intracellular Forms
Boric acid and borates are rapidly absorbed from the gastrointestinal tract with more than 90% efficiency (11). Borates are not absorbed through skin, but small amounts can be absorbed by inhalation of dust from occupational and consumer product exposures. The major form in blood and other body fluids is boric acid, which is distributed to all tissues. Human semen concentrations from physiologically normal men are four times higher than
in blood in low-B regions, but the ratio is lower in men living in higher-B regions (50). This finding suggests that borate/boric acid export transporters are present in the prostate or seminal glands. More than 90% of ingested B is eliminated as boric acid in the urine of humans and rats following first-order kinetics. The half-life of renal clearance is approximately 21 hours in humans, renal reabsorption occurs when the ratio of B to creatinine is less than 1 (51). Locksley and Sweet (52) conducted a dose-response mouse toxicity study using intraperitoneal injections of borax. Tissue B concentrations increased proportionally over a range of 1.8 to 71 mg B/kg. Ku et al (53) evaluated the tissue concentrations of male rats fed a diet containing 1575 mg B/kg for 7 days. After bone, the seminal vesicles accumulated the next highest concentration and are a known target of toxic exposure in rats.
in blood in low-B regions, but the ratio is lower in men living in higher-B regions (50). This finding suggests that borate/boric acid export transporters are present in the prostate or seminal glands. More than 90% of ingested B is eliminated as boric acid in the urine of humans and rats following first-order kinetics. The half-life of renal clearance is approximately 21 hours in humans, renal reabsorption occurs when the ratio of B to creatinine is less than 1 (51). Locksley and Sweet (52) conducted a dose-response mouse toxicity study using intraperitoneal injections of borax. Tissue B concentrations increased proportionally over a range of 1.8 to 71 mg B/kg. Ku et al (53) evaluated the tissue concentrations of male rats fed a diet containing 1575 mg B/kg for 7 days. After bone, the seminal vesicles accumulated the next highest concentration and are a known target of toxic exposure in rats.
Functions in Metabolism and Biology
Unique roles have been identified for B in three different biologic processes. In vascular plants, polysaccharide chains of the most complex carbohydrate known, rhamnogalacturonan II, provide scaffolding to maintain the architecture of cells as they expand to hundreds of times their length during growth. As tremendous turgid pressure elongates the cell, borate esters link dimers of rhamnogalacturonan II together to prevent rupture (22). Several transporters have been identified in plants that move borate anions from the roots to the shoots and export B when levels become excessive (54). One transporter has been identified in animal cells, but this has not been confirmed by other laboratories (55). Myxobacteria synthesize antibiotics that contain a single B atom (56, 57, 58). Gram-positive and Gram-negative bacteria synthesize an autoinducer that contains a single B atom and coordinates gene expression among different species (59).
Hunt (60, 61, 62, 63) proposed that B acts in animals by altering energy substrate utilization, mineral metabolism, vitamin metabolism, and enzyme activities, as well as by perturbing the immune system and more. Cui, Barranco, and Eckhert et al (64, 65, 66) used epidemiology as a tool to screen for B-responsive health effects and found that intake from food and regional groundwater was inversely associated with prostate cancer. The plausibility of this hypothesis has been confirmed in both cell culture and animal models (67). These investigators proposed that these effects were secondary to B’s ability to modulate the nicotinamide adenine dinucleotide (NAD+)/CD38/cyclic adenosine diphosphate ribose (cADPR) intercellular signaling pathway (68). Extracellular NAD+ derived from active secretion by cells or their necrosis binds to CD38 on the plasma membrane of neighboring cells (69). CD38 is a multifunctional enzyme that converts NAD+ to cADPR, an intracellular messenger. cADPR is released into the cytoplasm, where it binds to the ryanodine receptor, a Ca2+ channel that controls the release of Ca2+ stores in the endoplasmic reticulum into the cytoplasm. Mass spectrometry showed boric acid bound to NAD+ and cADPR (70, 71). Confocal Ca2+ imaging identified boric acid acts as a reversible competitive inhibitor of cADPR-stimulated Ca2+ release (72). The ability to modulate cADPR-stimulated Ca2+ release was within the dietary controlled blood range of healthy humans and was dose dependent. The effect occurred within seconds and led to a 30% drop in endoplasmic reticular Ca2+ levels. Ca2+ signaling and phosphorylation represent the major ways cells adapt to changes in their environment, and the relationship between Ca2+ and cell proliferation is well established (73).
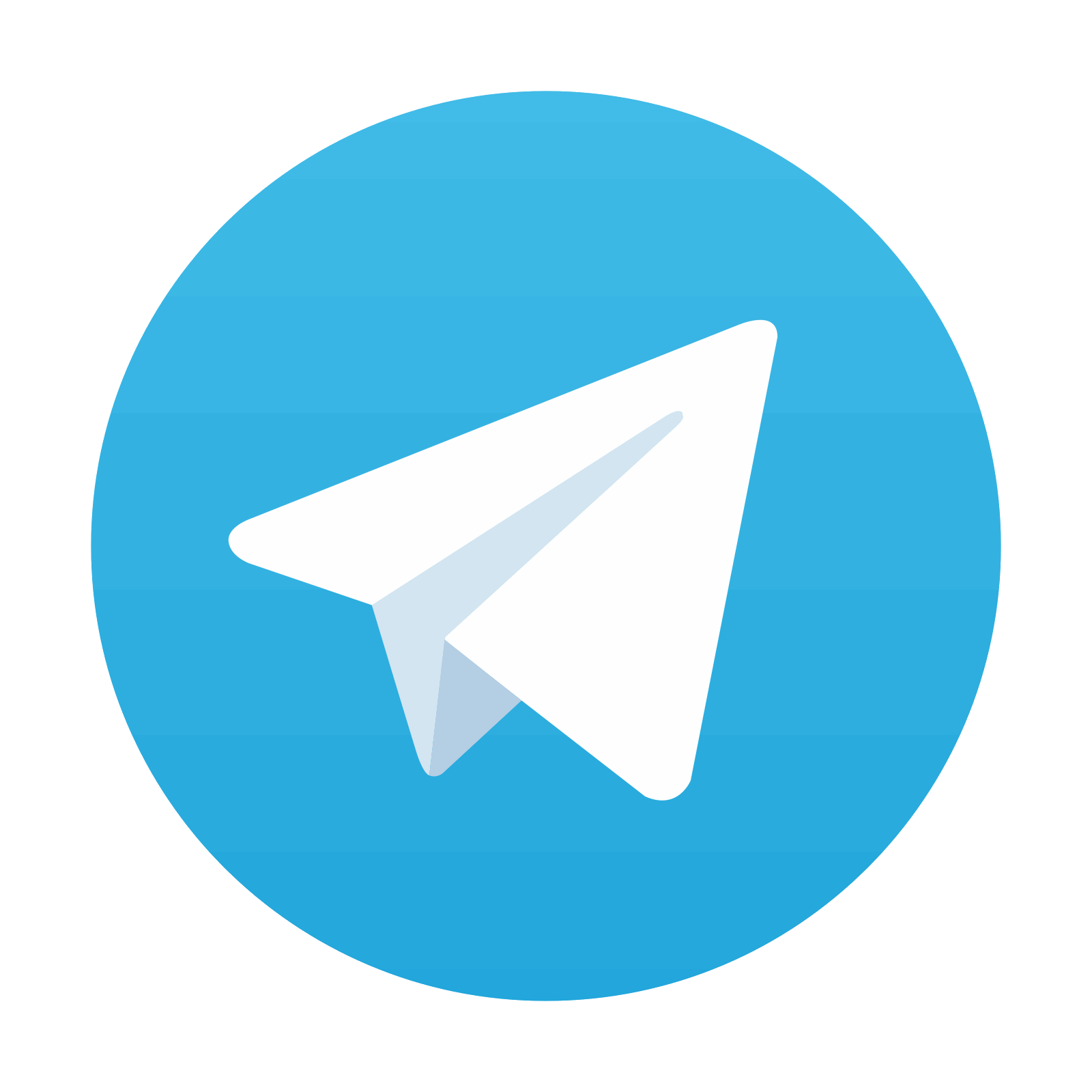
Stay updated, free articles. Join our Telegram channel

Full access? Get Clinical Tree
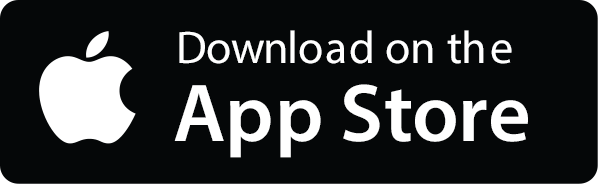
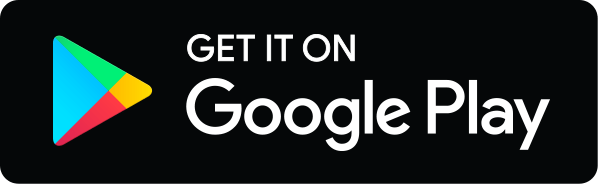