Chapter 1 The structure and function of skin
Skin is a double-layered membrane covering the exterior of the body and consists of a stratified cellular epidermis and an underlying dermis of connective tissue. In adults, the skin weighs over 5 kg and covers a surface area approaching 2 m2. The epidermis is mainly composed of keratinocytes and is typically 0.05–0.1 mm in thickness. The dermis contains collagen, elastic tissue and ground substance and is of variable thickness, from 0.5 mm on the eyelid or scrotum to more than 5 mm on the back (Fig. 1.1).
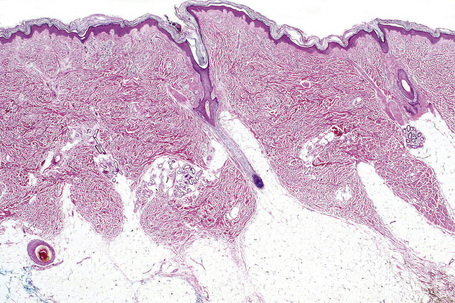
Fig. 1.1 Skin from forearm: there is a fairly thin epidermis. Compare the thickness of the dermis with that from the back (see Fig. 1.5).
Normal epidermal histology
An eosinophilic acellular layer known as the stratum lucidum is sometimes seen in skin from the palms and soles (Fig. 1.2).
Basal cells are cuboidal or columnar with a large nucleus typically containing a conspicuous nucleolus. Small numbers of mitoses may be evident. Clear cells are also present in the basal layer of the epidermis; these represent melanocytes. Cells with clear cytoplasm seen in the stratum spinosum represent Langerhans cells. Very occasional Merkel cells may also be present but these are not easily identified in hematoxylin and eosin stained sections. Histologically, prickle cells are polygonal in outline, have abundant eosinophilic cytoplasm and oval vesicular nuclei, often with conspicuous nucleoli. Keratohyalin granules typify the granular cell layer (Fig. 1.3). Further maturation leads to loss of nuclei and flattening of the keratinocytes to form the plates of the keratin layer (stratum corneum). Adjacent cells are united at their free borders by intercellular bridges (prickles), which are most clearly identifiable in the prickle cell layer and in disease states of the skin where there is marked intercellular edema (spongiosis) (Fig. 1.4).
Toker cells represent an additional clear cell population, which may be found in nipple epidermis of both sexes in up to 10% of the population.1 The cells are large, polygonal or oval and have abundant pale staining or clear cytoplasm with vesicular nuclei often containing prominent, albeit small, nucleoli. The cytoplasm is mucicarmine and PAS negative.1 The cells may be distributed singly but more often they are found as small clusters, not uncommonly forming single layered ductules.1 They are located along the basal layer of the epidermis or suprabasally and are also sometimes seen within the epithelium of the terminal lactiferous duct.
Toker cells are of particular importance as they may be mistaken by the unwary as Paget cells. They are thought to be the source of mammary Paget’s disease in those exceptional cases where an underlying ductal carcinoma is absent.2 Toker cells express CK7, AE1, CAM 5.2, epithelial membrane antigen (EMA), cerbB2, estrogen and progesterone receptors.3,4 They do not express p53 or CD138. Carcinoembryonic antigen (CEA) may also be present albeit weakly.4 Paget’s cells by way of contrast are often negative for estrogen and progesterone receptors and are p53 and CD138 positive.4
Regional variations in skin anatomy
Regional variation in skin structure is illustrated in Figures 1.5–1.20.
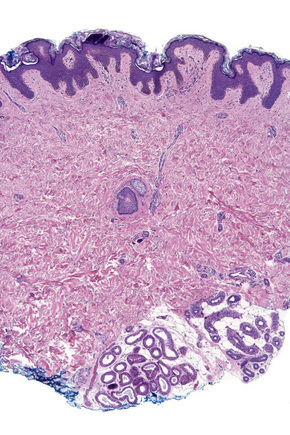
Fig. 1.9 Skin from axilla: apocrine glands as seen at the bottom of the field are typical for this site.
Skin development
Two major embryological elements juxtapose to form skin. These comprise the prospective epidermis that originates from a surface area of the early gastrula, and the prospective mesoderm that comes into contact with the inner surface of the epidermis during gastrulation. The mesoderm generates the dermis and is involved in the differentiation of epidermal structures such as hair follicles.1 Melanocytes are derived from the neural crest. After gastrulation, there is a single layer of neuroectoderm on the embryo surface: this layer will go on to form the nervous system or the skin epithelium, depending on the molecular signals (e.g., fibroblast growth factors or bone morphogenic proteins) it receives.2 The embryonic epidermis consists of a single layer of multipotent epithelial cells which is covered by a special layer known as periderm that is unique to mammals. Periderm provides some protection to the newly forming skin as well as exchange of material with the amniotic fluid. The embryonic dermis is at first very cellular and at 6–14 weeks three types of cell are present: stellate cells, phagocytic macrophages and granule-secretory cells, either melanoblasts or mast cells (Fig. 1.21). From weeks14 to 21, fibroblasts are numerous and active, and perineural cells, pericytes, melanoblasts, Merkel cells and mast cells can be individually identified. Hair follicles and nails are evident at 9 weeks. Sweat glands are also noted at 9 weeks on the palms and the soles.3 Sweat glands at other sites and sebaceous glands appear at 15 weeks. Touch pads become recognizable on the fingers and toes by the sixth week and development is maximal by the 15th week. The earliest development of hair occurs at about 9 weeks in the regions of the eyebrow, upper lip and chin. Sebaceous glands first appear as hemispherical protuberances on the posterior surfaces of the hair pegs and become differentiated at 13–15 weeks. Langerhans cells are derived from the monocyte–macrophage–histiocyte lineage and enter the epidermis at about 12 weeks. Merkel cells appear in the glabrous skin of the fingertips, lip, gingiva and nail bed, and in several other regions, around 16 weeks. Although some cells of the dermis may migrate from the dermatome (venterolateral part of the somite) and take part in the formation of the skin, most of the dermis is formed by mesenchymal cells that migrate from other mesodermal areas.4 These mesenchymal cells give rise to the whole range of blood and connective tissue cells, including the fibroblasts and mast cells of the dermis and the fat cells of the subcutis. In the second month, the dermis and subcutis are not discernible as distinct skin layers but collagen fibers are evident in the dermis by the end of the third month. Later, the papillary and reticular layers become established and, at the fifth month, the connective tissue sheaths are formed around the hair follicles. Elastic fibers are first detectable at 22 weeks.
Keratinocyte biology
The cytoskeleton of all mammalian cells, including epidermal keratinocytes, comprises actin containing microfilaments ≈︀7 nm in diameter, tubulin containing microtubules 20–25 nm in diameter, and filaments of intermediate size, 7–10 nm in diameter, known as intermediate filaments. There are six types of intermediate filaments of which keratins are the filaments in keratinocytes (Figs 1.22, 1.23). The human genome possesses 54 functional keratin genes located in two compact gene clusters, as well as many nonfunctional pseudogenes, scattered around the genome.1 Keratin genes are very specific in their expression patterns. Each one of the many highly specialized epithelial tissues has its own profile of keratins. Hair and nails express modified keratins containing large amounts of cysteine which forms numerous chemical cross-links to further strengthen the cytoskeleton. The genes encoding the keratins fall into two gene families: type I (basic) and type II (acidic) and there is coexpression of particular acidic–basic pairs in a cell- and tissue-specific manner. Keratin heterodimers are assembled into protofibrils and protofilaments by an antiparallel stagger of some complexity. Simple epithelia are characterized by the keratin pair K8/K18, and the stratified squamous epithelia by K5/K14. Suprabasally, keratins K1/K10 are characteristic of epidermal differentiation (Fig. 1.24). K15 is expressed in some interfollicular basal keratinocytes as well as keratinocytes within the hair-follicle bulge region at the site of pluripotential stem cells. K9 and K2e expression is site restricted in skin: K9 to palmoplantar epidermis and K2e to superficial interfollicular epidermis.
Apart from their structural properties, keratins may also have direct roles in cell signaling, the stress response and apoptosis.2 In epidermal hyperproliferation, as in wound healing and psoriasis, expression of suprabasal keratins K6/K16/K17 is rapidly induced.
Currently, 21 of the 54 known keratin genes have been linked to monogenic genetic disorders, and some have been implicated in more complex traits, such as idiopathic liver disease or inflammatory bowel disease.3,4 The first genetic disorder of keratin to be described was epidermolysis bullosa simplex, which involves mutations in the genes encoding K5 or K14 (Fig. 1.25). About half of the keratin genes are expressed in the hair follicle, and mutations in these genes may underlie cases of monilethrix as well as hair and nail ectodermal dysplasias.5
Epidermal stem cells
To maintain, repair and regenerate itself, the skin contains stem cells which reside in the bulge area of hair follicles, the basal layer of interfollicular epidermis and the base of sebaceous glands (Fig. 1.26).1 Stem cells are able to self-renew as well as give rise to differentiating cells.2 It is not clear, however, whether every basal keratinocyte or only a proportion of cells is a stem cell.3 Two possible hypotheses have emerged. One theory divides basal keratinocytes into epidermal proliferation units, which comprise one self-renewing stem cell and about 10 tightly packed transient amplifying cells, each of which is capable of dividing several times and then exiting the basal layer to undergo terminal differentiation.4 This unit gives rise to a column of larger and flatter cells that culminates in a single hexagonal surface. The process of division of basal cells in this model is viewed as a symmetrical process in which equal daughter cells are generated with the basal cells progressively reducing their adhesiveness to the underlying epidermal basement membrane, delaminating and committing to terminal differentiation. The alternative theory is that some basal cells (perhaps up to 70% of cells) can undergo asymmetrical cell division, shifting their spindle orientation from lateral to perpendicular.5 Asymmetrical cell divisions provide a means of maintaining one proliferative daughter while the other daughter cell is committed to terminal differentiation. Asymmetrical cell divisions, therefore, can bypass the need for transient amplifying cells.
Hair follicle stem cells are found in the bulge regions below the sebaceous glands. These stem cells are slow cycling and express the cell surface molecules CD34 and VdR as well as the transcription factors TCF3, Sox9, Lhx2 and NFATc1 (Fig. 1.27). The bulge area stem cells generate cells of the outer root sheath, which drive the highly proliferative matrix cells next to the mesenchymal papillae. After proliferating, matrix cells differentiate to form the hair channel, the inner root sheath and the hair shaft. Hair follicle stem cells can also differentiate into sebocytes and interfollicular epidermis. Despite this multipotency, however, the follicle stem cells only function in pilosebaceous unit homeostasis and do not contribute to interfollicular epidermis unless the skin is wounded.6
Stem cells are also found in the base of sebaceous glands: the progeny of these cells differentiate into lipid-filled sebocytes. Apart from stem cells in the hair follicles, sebaceous glands and interfollicular epidermis, other cells in the dermis and subcutis may have stem cells properties. These include cells that have been termed skin-derived precursors (SKPs), which can differentiate into both neural and mesodermal progeny.7 In addition, a subset of dermal fibroblasts can have adipogenic, osteogenic, chondrogenic, neurogenic and hepatogenic differentiation potential.8
Skin barrier
A major function of the epidermis is to form a barrier against the external environment. To achieve this, terminal differentiation of keratinocytes results in formation of the cornified cell envelope. This physical barrier is rendered highly insoluble by the formation of glutamyl-lysyl isodipeptide bonds between envelope proteins, catalyzed by transglutaminases.1 Several different proteins contribute to construction of the cornified cell envelope, including involucrin, and the family of small proline-rich proteins (SPR1) including cornifin or SPR1 and pancornulins. Other envelope proteins include SKALP/elafin and keratolinin/cystatin. Some precursors of the cornified envelope are delivered by granules: small, smooth, sulfur-rich L granules contain the cysteine-rich protein loricrin, and accumulate in the stratum granulosum.2 Loricrin is the major component of the cornified envelope. Profilaggrin in F granules may make a minor contribution to the envelope. Membrane-associated proteins that contribute to the cornified envelope include the plakin family members, periplakin, envoplakin, epiplakin, desmoplakin as well as plectin. Formation of the cornified cell envelope is triggered by a rise in intracellular calcium levels.3 This leads to cross-link formation between plakins and involucrin catalyzed by transglutaminases. Other desmosomal proteins are then also cross-linked, forming a scaffold along the entire inner surface of the plasma membrane. Ceramides from the secreted contents of lamellar bodies are then esterified onto glutamine residues of the scaffold proteins. The cornified cell envelope is reinforced by the addition of a variable amount of SPRs, repetin, trichohyalin, cystostatin α, elafin and LEP/XP-5 (skin-specific protein). Although most desmosomal components are degraded, keratin intermediate filaments (mostly K1, K10 and K2e) may be cross-linked to desmoplakin and envoplakin remnants.
In the upper stratum spinosum and stratum granulosum lipid is synthesized and packaged into lamellated membrane-bound organelles known as membrane-coating granules, lamellar granules or Odland bodies (Fig. 1.28).4 They are found adjacent to the cell membrane with alternating thick and thin dense lines separated by lighter lamellae of equal width, consistent with packing of flattened discs within a membrane boundary. These granules contain phospholipids, glycolipids and free sterols and move towards the plasma membrane as the cells move through the granular layer where they cluster at the cell membrane. They fuse with the plasma membrane, dispersing their contents into the intercellular space. Polar lipids from the lamellar granules are remodeled into neutral lipids in the intercellular space between corneocytes, thereby contributing to the barrier.
Within the granular layer of the epidermis, the main keratinocyte proteins are keratin and filaggrin, which together contribute approximately 80–90% of the mass of the epidermis and are ultrastructurally represented by the keratohyalin granules (Fig. 1.29). Filaggrin is initially synthesized as profilaggrin, a ≈︀500-kDa highly phosphorylated, histidine-rich polypeptide. During the post-translational processing of profilaggrin, the individual filaggrin polypeptides, each ≈︀35 kD, are proteolytically released. These are then dephosphorylated, a process that assists keratin filament aggregation and explains the origin of the name ‘filaggrin’ (filament aggregating protein) (Fig. 1.30). Typically, there are 10 highly homologous filaggrin units, although the number of filaggrin repeat units is variable and genetically determined, with duplications of filaggrin repeat units 8 and/or 10 in some individuals. Fewer filaggrin repeats leads to dryer skin. Loss-of-function mutations in filaggrin are very common, occurring in up to 10% of the European population. These mutations lead to reduced or absent keratohyalin granules, and are the cause of ichthyosis vulgaris as well as constituting a major risk factor for atopic dermatitis (Fig. 1.31).5
Skin immunity
Skin possesses both innate and adaptive immune responses to defend against microbial pathogens and thereby prevent infection. One of the primary mechanisms is the synthesis, expression and release of antimicrobial peptides (Fig. 1.32).1 There are more than 20 antimicrobial peptides in the skin, including cathelicidins, β-defensins, substance P, RANTES, RNase 2, 3, and 7, and S100A7. Many of these peptides have antimicrobial action against bacteria, viruses, and fungi. In the stratum corneum there is an effective chemical barrier maintained by the expression of S100A7 (psoriasin).2 This antimicrobial substance is very effective at killing Escherichia coli. Subjacent to this in the skin there is another class of antimicrobial peptides, such as RNASE7, which is effective against a broad spectrum of microorganisms, especially enterococci.3 Below this in the living layers of the skin are other antimicrobial peptides including the β-defensins.4 The antimicrobial activity of most peptides occurs as a result of unique structural characteristics that enable them to disrupt the microbial cell membrane while leaving human cell membranes intact. The antimicrobial peptides can have immunostimulatory and immunomodulatory capacities as well as being chemotactic for distinct subpopulations of leukocytes and other inflammatory cells.5 Some peptides have additional roles in signaling host responses through chemotactic, angiogenic, growth factor and immunosuppressive activity. These peptides are known as alarmins.6 Alarmins may also stimulate parts of the host defense system, such as barrier repair and recruitment of inflammatory cells.
Skin immunity is also provided by a distinct population of antigen presenting cells in the epidermis known as Langerhans cells (Fig. 1.33). These are dendritic cells that were first described by Langerhans, who demonstrated their existence in human epidermis by staining with gold chloride. Without stimulation, Langerhans cells exhibit a unique motion termed ‘Dendrite Surveillance Extension And Retraction Cycling Habitude (dSEARCH)’.7 This is characterized by rhythmic extension and retraction of dendritic processes between intercellular spaces. When exposed to antigen, there is greater dSEARCH motion and also direct cell-to-cell contact between adjacent Langerhans cells which function as intraepidermal macrophages, phagocytosing antigens among keratinocytes. Langerhans cells then leave the epidermis and migrate via lymphatics to regional lymph nodes. In the paracortical region of lymph nodes the Langerhans cell expresses protein on its surface to present to a T lymphocyte that can then undergo clonal proliferation. Langerhans cells, in combination with macrophages and dermal dendrocytes, represent the skin’s mononuclear phagocyte system.8 By electron microscopy, Langerhans cells have a lobulated nucleus, a relatively clear cytoplasm and well-developed endoplasmic reticulum, Golgi complex and lysosomes. They also possess characteristic granules which are rod or racquet-shaped (Fig. 1.34). These ‘Birbeck’ granules represent subdomains of the endosomal recycling compartment and form at sites where the protein Langerin accumulates.
Besides antigen detection and the processing role by epidermal Langerhans cells, cutaneous immune surveillance is also carried out in the dermis by an array of macrophages, T cells and dendritic cells. These immune sentinel and effector cells can provide rapid and efficient immunologic back-up to restore tissue homeostasis if the epidermis is breached. The dermis contains a very large number of resident T cells. Indeed, there are approximately 2 × 1010 resident T cells, which is twice the number of T cells in the circulating blood. Dermal dendritic cells may also have potent antigen-presenting capacities or the potential to develop into CD1a-positive and Langerin-positive cells. Dermal immune sentinels are capable of acquiring an antigen-presenting mode, a migratory mode or a tissue resident phagocytic mode.9
Melanocytes
Melanocytes are pigment-producing cells and are found in the skin, inner ear, choroid and iris of the eye. In skin, melanocytes are located in the basal keratinocyte layer. The ratio of melanocytes to basal cells ranges from approximately 1:4 on the cheek to 1:10 on the limbs. They appear as vacuolated cells in hematoxylin and eosin stained sections (Fig. 1.35). Ultrastructurally, melanocytes have pale cytoplasm and are devoid of tonofilaments and desmosomes (Fig. 1.36). They are easily recognized by their specific cytoplasmic organelles (melanosomes) which are derived from the smooth endoplasmic reticulum. Melanosomes are believed to represent a specialized variant of lysosome (Fig. 1.37). The function of melanocytes is the production of melanin, a pigment that varies in color from yellow to brown or black and accounts for the various skin colors within and among races. Melanin protects the mitotically active basal epidermal cells from the injurious effects of ultraviolet light, which accounts for individuals with less pigmentation (fair-haired and light-skinned) having a much greater risk of sunburn and developing cutaneous malignancies (squamous cell and basal cell carcinomas, and melanoma). The mechanism involves absorbing or scattering ultraviolet radiation and/or its photoproducts. Other functions of melanin include control of vitamin D3 synthesis and local thermoregulation.
Melanocytes also possess melanocyte-specific receptors including melanocortin-1 (MC1R) and melatonin receptors.1 The activation or the inhibition of melanocyte-specific receptors can augment normal melanocyte function, skin color, and photoprotection. Moreover, receptor polymorphisms are known to underlie red hair phenotypes.2 Hair graying reflects abnormalities in melanocyte signaling. Notably, Notch transcription factor signaling in melanocytes is essential for the maintenance of proper hair pigmentation, including regeneration of the melanocyte population during hair follicle cycling.3
Melanin is transferred from melanocytes in melanosomes to neighboring keratinocytes in the epidermis and into the growing shaft in hair follicles and can be identified by silver techniques such as the Masson-Fontana reaction (Fig. 1.38). Transport occurs along the dendritic processes of the melanocytes and the melanosomes are engulfed as membrane-bound (lysosomal) single or compound melanosomes by a group of adjacent largely basally located keratinocytes (epidermal melanin unit) where they are typically seen in an umbrella-like distribution over the outer aspect of the nucleus (Fig. 1.39). A compound melanosome typically contains from three to six single melanosomes. In heavily pigmented skin and dark hair, melanosomes remain solitary and are longer than those seen in melanogenesis in paler races. Other cells that may contain compound melanosomes include macrophages (melanophages), melanoma cells and, occasionally, Langerhans cells, the other type of epidermal dendritic cell. Macromelanosomes (giant melanosomes) measure several microns in diameter and therefore are readily visible in hematoxylin and eosin stained sections (Fig. 1.40). They may be encountered in normal skin, in lentigines, dysplastic nevi, Spitz nevi, in the café-au-lait macules of neurofibromatosis and in albinism. A key protein involved in melanosome assembly is NCKX5, encoded by the gene SLC24A5.4 Loss of expression of this gene in mice results in marked changes in skin color with loss of pigment. Mature melanosomes of eumelanin are ellipsoidal in shape, while pheomelanin-producing melanosomes are spherical.
Merkel cells
Merkel cells are postmitotic cells scattered throughout the epidermis of vertebrates and constitute 0.2–0.5% of epidermal cells.1 Merkel cells represent part of the affector limb in cutaneous slowly adapting type-1 (SA1) mechanoreceptors and are therefore particularly concerned with touch sensation. They are located amongst basal keratinocytes and are mainly found in hairy skin, tactile areas of glabrous skin, taste buds, the anal canal, labial epithelium and eccrine sweat glands. In glabrous skin, the density of Merkel cells is ≈︀50 per mm2. Sun-exposed skin may contain twice as many Merkel cells as non-sun-exposed skin.2 Numerous Merkel cells can be found in actinic keratoses.3 Merkel cells cannot be recognized in conventional hematoxylin and eosin stained sections. Rather, immunocytochemistry, particularly using antikeratin antibodies, or electron microscopy, is necessary for their identification (Figs 1.41 and 1.42).
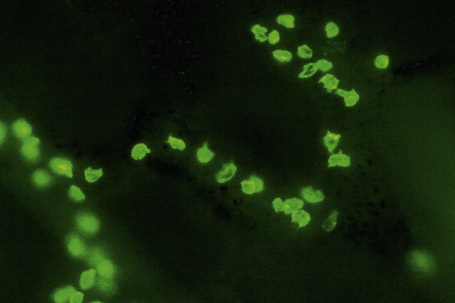
Fig. 1.41 Merkel cells: separated human epidermis showing a striking linear arrangement (troma-1 antibody).
By courtesy of J.P. Lacour, MD, and J.P. Ortonne, MD, University of Nice, France.
Ultrastructurally, Merkel cells appear oval with a long axis of ≈︀15 μm orientated parallel to the basement membrane (Fig. 1.43). They also have a large bilobed nucleus and clear cytoplasm which reflects a relative scarcity of intracellular organelles. Merkel cells contain numerous neurosecretory granules, each 50 nm to 160 nm across; these are found opposing the junctions with the sensory nerve ending (Fig. 1.44). Merkel cells contain keratin filaments, particularly keratin filament types 8, 18, 19, and 20, which are characteristic of simple epithelium and fetal epidermis. Immunocytochemically, Merkel cells also express neuropeptides including synaptophysin, vasoactive intestinal peptide (VIP) and calcitonin gene-related polypeptide (CGRP).4,5 They contain neuron-specific proteins including neuron-specific enolase (NSE) and protein gene product (PGP) 9.5.6 In addition, Merkel cells express desmosomal proteins, membranous neural cell adhesion molecule and nerve growth factor receptor.7–9 Merkel cells show a positive uranaffin reaction.10 Merkel cells form close connections with sensory nerve endings and secrete or express a number of these peptides.11 The close contact between Merkel cells and nerve fibers represents a Merkel cell–neurite complex, but there is no clear evidence of synaptic transmission, although numerous vesicles can be identified in neurons apposed to Merkel cells.12
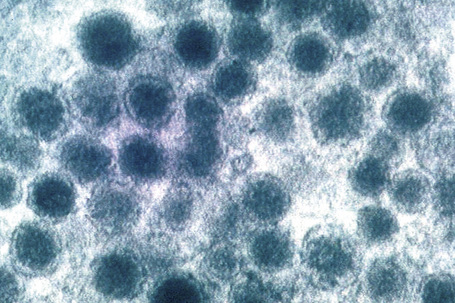
Fig. 1.44 Merkel cell granules: they are membrane bound and measure approximately 150 nm in diameter.
By courtesy of A.S. Breathnach, MD (1977) Electron microscopy of cutaneous nerves and receptors. Journal of Investigative Dermatology 69, 8–26. Blackwell Publishing Inc., USA.
There are two hypotheses for the origin of Merkel cells: one possibility is that they differentiate from epidermal keratinocyte-like cells and the other is that they arise from stem cells of neural crest origin that migrated during embryogenesis, in similar fashion to melanocytes.13 Merkel cell hyperplasia is a common histological finding and may accompany keratinocyte hyperproliferation as well as being frequently seen in adnexal tumors such as nevus sebaceus, trichoblastomas, trichoepitheliomas, and nodular hidradenomas.14 Merkel cell hyperplasia is associated with hyperplasia of nerve endings that occurs in neurofibromas, neurilemomas, nodular prurigo, or neurodermatitis. It is not clear whether Merkel cell carcinoma originates from Merkel cells or their precursors but the latter may be more likely given that many dermal Merkel cell carcinomas do not connect with the epidermis.
Intercellular junctions
Desmosomes are the major intercellular adhesion complexes in the epidermis. They anchor keratin intermediate filaments to the cell membrane and link adjacent keratinocytes (Fig. 1.45). Desmosomes are found in the epidermis, myocardium, meninges and cortex of lymph nodes. Ultrastructurally, desmosomes contain plaques of electron-dense material running along the cytoplasm parallel to the junctional region, in which three bands can be distinguished: an electron-dense band next to the plasma membrane, a less dense band, and then a fibrillar area (Fig. 1.46).1 Identical components are present on opposing cells which are separated by an intercellular space of 30 nm within which there is an electron-dense midline. There are three main protein components of desmosomes in the epidermis: the desmosomal cadherins, the armadillo family of nuclear and junctional proteins, and the plakins (Fig. 1.47).2 The transmembranous cadherins comprise mostly heterophilic associations of desmogleins and desmocollins. There are four main epidermis-specific desmogleins (Dsg1–4) and three desmocollins (Dsc1–3). These show differentiation-specific expression. For example, Dsg1 and Dsc1 are found predominantly in the superficial layers of the epidermis whereas Dsg3 and Dsc3 show greater expression in basal keratinocytes. The intracellular parts of the cadherins interact with the keratin filament network via the desmosomal plaque proteins, mainly desmoplakin, plakoglobin and plakophilin.1
< div class='tao-gold-member'>
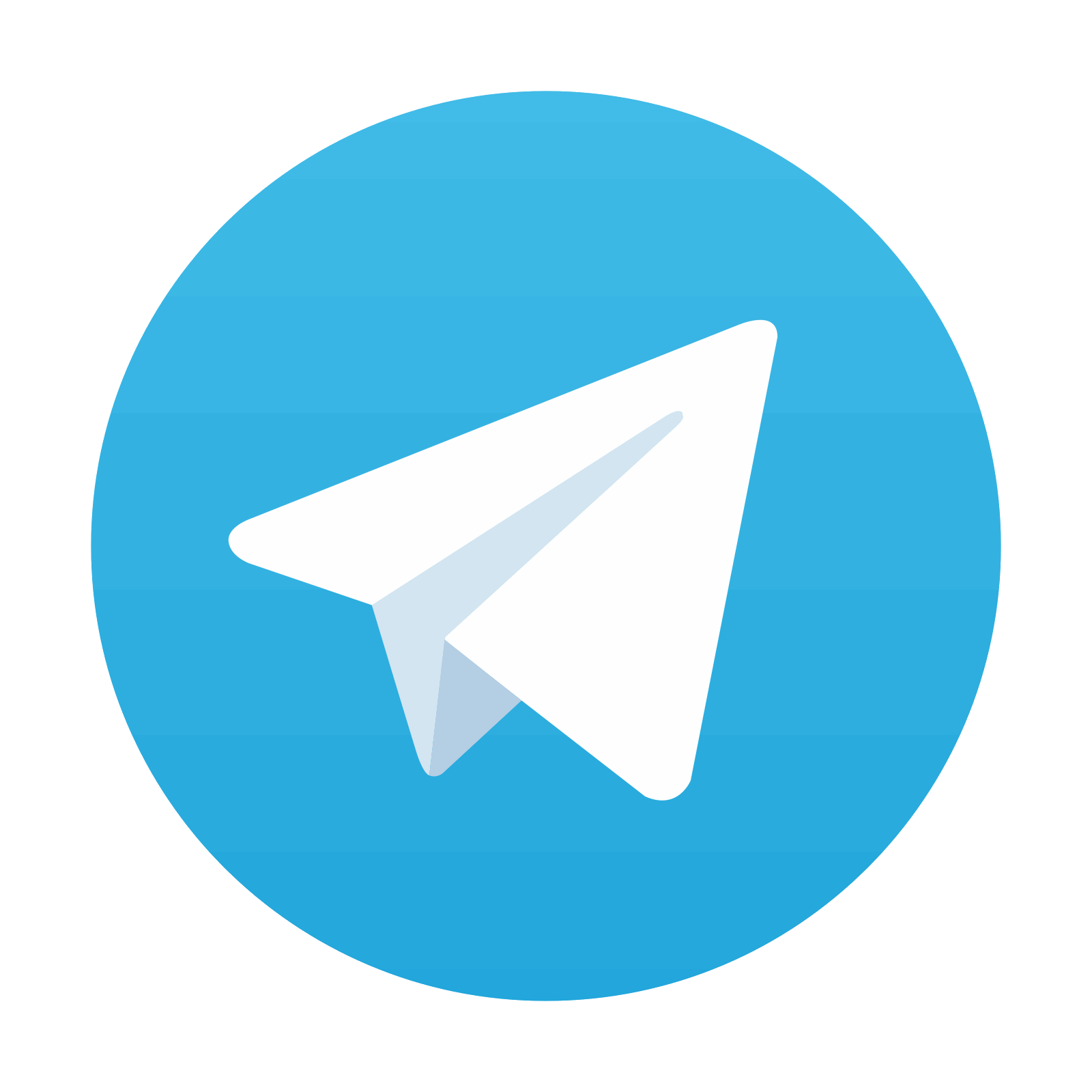
Stay updated, free articles. Join our Telegram channel

Full access? Get Clinical Tree
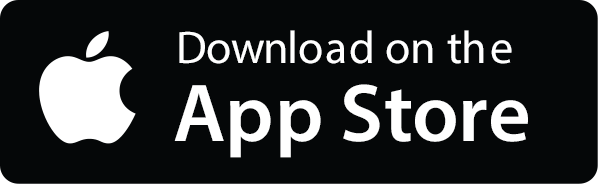
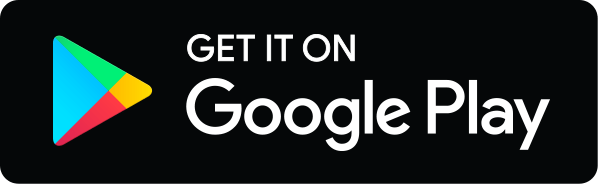