13
The Respiratory Chain & Oxidative Phosphorylation
Kathleen M. Botham, PhD, DSc & Peter A. Mayes, PhD, DSc
OBJECTIVES
After studying this chapter, you should be able to:
Describe the double membrane structure of mitochondria and indicate the location of various enzymes.
Appreciate that energy from the oxidation of fuel substrates (fats, carbohydrates, amino acids) is almost all liberated in mitochondria as reducing equivalents, which are passed by a process termed electron transport through a series of redox carriers or complexes embedded in the inner mitochondrial membrane known as the respiratory chain until they are finally reacted with oxygen to form water.
Describe the four protein complexes involved in the transfer of electrons through the respiratory chain and explain the roles of flavoproteins, iron sulfur proteins, and coenzyme Q.
Understand how coenzyme Q accepts electrons from NADH via Complex I and from FADH2 via Complex II.
Indicate how electrons are passed from reduced coenzyme Q to cytochrome c via Complex III in the Q cycle.
Explain the process by which reduced cytochrome c is oxidized and oxygen is reduced to water via Complex IV.
Understand how electron transport through the respiratory chain generates a proton gradient across the inner mitochondrial membrane, leading to the buildup of a proton motive force that generates ATP by the process of oxidative phosphorylation.
Describe the structure of the ATP synthase enzyme and explain how it works as a rotary motor to produce ATP from ADP and Pi.
Identify the five conditions controlling the rate of respiration in mitochondria and understand that oxidation of reducing equivalents via the respiratory chain and oxidative phosphorylation are tightly coupled in most circumstances, so that one cannot proceed unless the other is functioning.
Indicate examples of common poisons that block respiration or oxidative phosphorylation and identify their site of action.
Explain, with examples, how uncouplers may act as poisons by dissociating oxidation via the respiratory chain from oxidative phosphorylation, but may also have a physiological role in generating body heat.
Explain the role of exchange transporters present in the inner mitochondrial membrane in allowing ions and metabolites to pass through while preserving electrochemical and osmotic equilibrium.
BIOMEDICAL IMPORTANCE
Aerobic organisms are able to capture a far greater proportion of the available free energy of respiratory substrates than anaerobic organisms. Most of this takes place inside mitochondria, which have been termed the “powerhouses” of the cell. Respiration is coupled to the generation of the high-energy intermediate, ATP, by oxidative phosphorylation. A number of drugs (eg, amobarbital) and poisons (eg, cyanide, carbon monoxide) inhibit oxidative phosphorylation, usually with fatal consequences. Several inherited defects of mitochondria involving components of the respiratory chain and oxidative phosphorylation have been reported. Patients present with myopathy and encephalopathy and often have lactic acidosis.
SPECIFIC ENZYMES ACT AS MARKERS OF COMPARTMENTS SEPARATED BY THE MITOCHONDRIAL MEMBRANES
Mitochondria have an outer membrane that is permeable to most metabolites and an inner membrane that is selectively permeable, enclosing a matrix within (Figure 13–1). The outer membrane is characterized by the presence of various enzymes, including acyl-CoA synthetase and glycerophosphate acyltransferase. Adenylyl kinase and creatine kinase are found in the intermembrane space. The phospholipid cardiolipin is concentrated in the inner membrane together with the enzymes of the respiratory chain, ATP synthase, and various membrane transporters.
FIGURE 13–1 Structure of the mitochondrial membranes. Note that the inner membrane contains many folds or cristae.
THE RESPIRATORY CHAIN OXIDIZES REDUCING EQUIVALENTS & ACTS AS A PROTON PUMP
Most of the energy liberated during the oxidation of carbohydrate, fatty acids, and amino acids is made available within mitochondria as reducing equivalents (—H or electrons) (Figure 13–2). Note that the enzymes of the citric acid cycle and β-oxidation (Chapters 22 & 17) are contained in mitochondria, together with the respiratory chain, which collects and transports reducing equivalents, directing them to their final reaction with oxygen to form water, and the machinery for oxidative phosphorylation, the process by which the liberated free energy is trapped as high-energy phosphate.
FIGURE 13–2 Role of the respiratory chain of mitochondria in the conversion of food energy to ATP. Oxidation of the major foodstuffs leads to the generation of reducing equivalents (2H) that are collected by the respiratory chain for oxidation and coupled generation of ATP.
Components of the Respiratory Chain Are Contained in Four Large Protein Complexes Embedded in the Inner Mitochondrial Membrane
Electrons flow through the respiratory chain through a redox span of 1.1 V from NAD+/NADH to O2/2H2O (Table 12-1), passing through three large protein complexes: NADH-Q oxidoreductase (Complex I), where electrons are transferred from NADH to coenzyme Q (Q) (also called ubiquinone); Q-cytochrome c oxidoreductase (Complex III), which passes the electrons on to cytochrome c; and cytochrome c oxidase (Complex IV), which completes the chain, passing the electrons to O2 and causing it to be reduced to H2O (Figure 13–3). Some substrates with more positive redox potentials than NAD+/NADH (eg, succinate) pass electrons to Q via a fourth complex, succinate-Q reductase (Complex II), rather than Complex I. The four complexes are embedded in the inner mitochondrial membrane, but Q and cytochrome c are mobile. Q diffuses rapidly within the membrane, while cytochrome c is a soluble protein. The flow of electrons through Complexes I, III, and IV results in the pumping of protons from the matrix across the inner mitochondrial membrane into the intermembrane space (Figure 13–7).
FIGURE 13–3 Overview of electron flow through the respiratory chain. (cyt, cytochrome; Q, coenzyme Q or ubiquinone.)
Flavoproteins and Iron-Sulfur Proteins (Fe-S) Are Components of the Respiratory Chain Complexes
Flavoproteins (Chapter 12) are important components of Complexes I and II. The oxidized flavin nucleotide (FMN or FAD) can be reduced in reactions involving the transfer of two electrons (to form FMNH2 or FADH2), but they can also accept one electron to form the semiquinone (Figure 12–2). Iron-sulfur proteins (nonheme iron proteins, Fe-S) are found in Complexes I, II, and III. These may contain one, two, or four Fe atoms linked to inorganic sulfur atoms and/or via cysteine-SH groups to the protein (Figure 13–4). The Fe-S take part in single electron transfer reactions in which one Fe atom undergoes oxidoreduction between Fe2+ and Fe3+.
FIGURE 13–4 Iron-sulfur proteins (Fe-S). (A) The simplest Fe-S with one Fe bound by fourcysteines. (B) 2Fe-2S center. (C) 4Fe-4S center. (Cys, cysteine; Pr, apoprotein; , Inorganic sulfur.)
Q Accepts Electrons via Complexes I and II
NADH-Q oxidoreductase or Complex I is a large L-shaped multisubunit protein that catalyzes electron transfer from NADH to Q, coupled with the transfer of four H+ across the membrane:
Electrons are transferred from NADH to FMN initially, then to a series of Fe-S centers, and finally to Q (Figure 13–5). In Complex II (succinate-Q reductase), FADH2 is formed during the conversion of succinate to fumarate in the citric acid cycle (Figure 17–3) and electrons are then passed via several Fe-S centers to Q (Figure 13–5). Glycerol-3-phosphate (generated in the breakdown of triacylglycerols or from glycolysis, Figure 18–2) and acyl-CoA also pass electrons to Q via different pathways involving flavoproteins (Figure 13–5).
FIGURE 13–5 Flow of electrons through the respiratory chain complexes, showing the entry points for reducing equivalents from important substrates. Q and cyt c are mobile components of the system as indicated by the dotted arrows. The flow through Complex III (the Q cycle) is shown in more detail in Figure 13–6. (ETF, electron transferring flavoprotein; Fe-S, iron-sulfur protein; cyt, cytochrome; Q, coenzyme Q or ubiquinone.)
The Q Cycle Couples Electron Transfer to Proton Transport in Complex III
Electrons are passed from QH2 to cytochrome c via Complex III (Q-cytochrome c oxidoreductase):
The process is believed to involve cytochromes c1, bL and bH and a Rieske Fe-S (an unusual Fe-S in which one of the Fe atoms is linked to two histidine residues rather than two cysteine residues) (Figure 13–5) and is known as the Q cycle (Figure 13–6). Q may exist in three forms: the oxidized quinone, the reduced quinol, or the semiquinone (Figure 13–6). The semiquinone is formed transiently during the cycle, one turn of which results in the oxidation of 2QH2 to Q, releasing 4H+ into the intermembrane space, and the reduction of one Q to QH2, causing 2H+ to be taken up from the matrix (Figure 13–6). Note that while Q carries two electrons, the cytochromes carry only one, thus the oxidation of one QH2
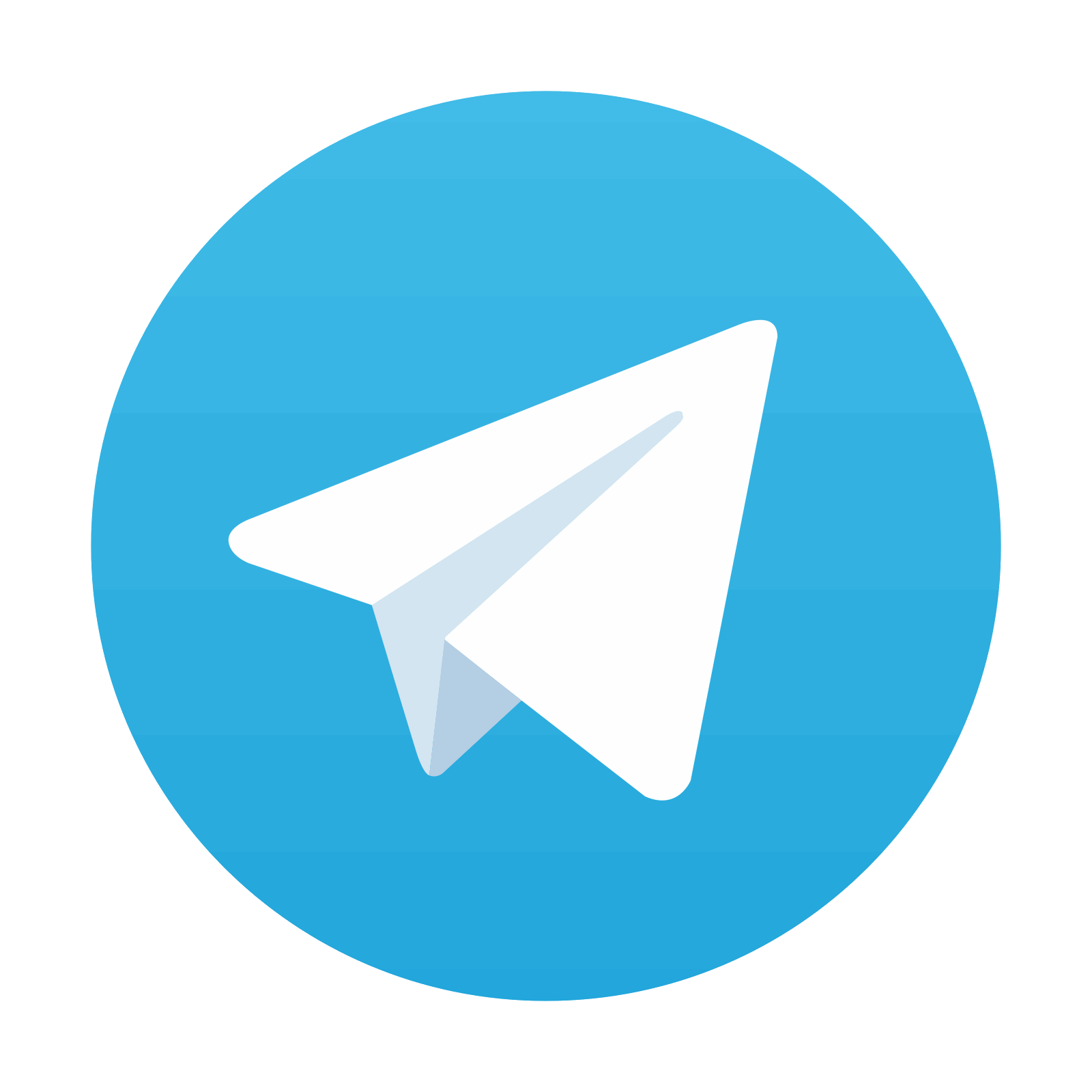
Stay updated, free articles. Join our Telegram channel

Full access? Get Clinical Tree
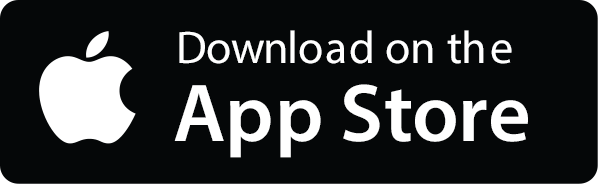
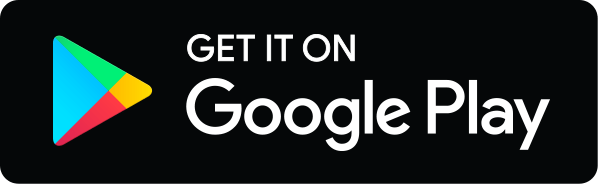