21
The Pentose Phosphate Pathway & Other Pathways of Hexose Metabolism
David A. Bender, PhD & Peter A. Mayes, PhD, DSc
OBJECTIVES
After studying this chapter, you should be able to:
Describe the pentose phosphate pathway and its roles as a source of NADPH and in the synthesis of ribose for nucleotide synthesis.
Describe the uronic acid pathway and its importance for synthesis of glucuronic acid for conjugation reactions and (in animals for which it is not a vitamin) vitamin C.
Describe and explain the consequences of large intakes of fructose.
Describe the synthesis and physiological importance of galactose.
Explain the consequences of genetic defects of glucose 6-phosphate dehydrogenase deficiency (favism), the uronic acid pathway (essential pentosuria), and fructose and galactose metabolism.
BIOMEDICAL IMPORTANCE
The pentose phosphate pathway is an alternative route for the metabolism of glucose. It does not lead to formation of ATP but has two major functions: (1) the formation of NADPH for synthesis of fatty acids and steroids, and maintaining reduced glutathione for antioxidant activity, and (2) the synthesis of ribose for nucleotide and nucleic acid formation. Glucose, fructose, and galactose are the main hexoses absorbed from the gastrointestinal tract, derived from dietary starch, sucrose, and lactose, respectively. Fructose and galactose can be converted to glucose, mainly in the liver.
Genetic deficiency of glucose 6-phosphate dehydrogenase, the first enzyme of the pentose phosphate pathway, is a major cause of acute hemolysis of red blood cells, resulting in hemolytic anemia. Glucuronic acid is synthesized from glucose via the uronic acid pathway, of minor quantitative importance, but of major significance for the conjugation and excretion of metabolites and foreign chemicals (xenobiotics) as glucuronides. A deficiency in the pathway leads to the condition of essential pentosuria. The lack of one enzyme of the pathway (gulonolactone oxidase) in primates and some other animals explains why ascorbic acid (vitamin C) is a dietary requirement for humans but not most other mammals. Deficiencies in the enzymes of fructose and galactose metabolism lead to metabolic diseases such as essential fructosuria, hereditary fructose intolerance, and galactosemia.
THE PENTOSE PHOSPHATE PATHWAY FORMS NADPH & RIBOSE PHOSPHATE
The pentose phosphate pathway (hexose monophosphate shunt) is a more complex pathway than glycolysis (Figure 21–1). Three molecules of glucose 6-phosphate give rise to three molecules of CO2 and three 5-carbon sugars. These are rearranged to regenerate two molecules of glucose 6-phosphate and one molecule of the glycolytic intermediate, glyceraldehyde 3-phosphate. Since two molecules of glyceraldehyde 3-phosphate can regenerate glucose 6-phosphate, the pathway can account for the complete oxidation of glucose.
FIGURE 21–1 Flow chart of pentose phosphate pathway and its connections with the pathway of glycolysis. The full pathway, as indicated, consists of three interconnected cycles in which glucose 6-phosphate is both substrate and end-product. The reactions above the broken line are nonreversible, whereas all reactions under that line are freely reversible apart from that catalyzed by fructose 1,6-bisphosphatase.
REACTIONS OF THE PENTOSE PHOSPHATE PATHWAY OCCUR IN THE CYTOSOL
Like glycolysis, the enzymes of the pentose phosphate pathway are cytosolic. Unlike glycolysis, oxidation is achieved by dehydrogenation using NADP+, not NAD+, as the hydrogen acceptor. The sequence of reactions of the pathway may be divided into two phases: an irreversible oxidative phase and a reversible nonoxidative phase. In the first phase, glucose 6-phosphate undergoes dehydrogenation and decarboxylation to yield a pentose, ribulose 5-phosphate. In the second phase, ribulose 5-phosphate is converted back to glucose 6-phosphate by a series of reactions involving mainly two enzymes: transketolase and transaldolase (see Figure 21–1).
The Oxidative Phase Generates NADPH
Dehydrogenation of glucose 6-phosphate to 6-phosphogluconate occurs via the formation of 6-phosphogluconolactone catalyzed by glucose 6-phosphate dehydrogenase, an NADP-dependent enzyme (Figures 21-1 and 21-2). The hydrolysis of 6-phosphogluconolactone is accomplished by the enzyme gluconolactone hydrolase. A second oxidative step is catalyzed by 6-phosphogluconate dehydrogenase, which also requires NADP+ as hydrogen acceptor. Decarboxylation follows with the formation of the ketopentose ribulose 5-phosphate.
In the endoplasmic reticulum, an isoenzyme of glucose 6-phosphate dehydrogenase, hexose 6-phosphate dehydrogenase, provides NADPH for hydroxylation (mixed function oxidase) reactions, and also for 11-β-hydroxysteroid dehydrogenase-1. This enzyme catalyzes the reduction of (inactive) cortisone to (active) cortisol in liver, the nervous system, and adipose tissue. It is the major source of intracellular cortisol in these tissues and may be important in obesity and the metabolic syndrome.
The Nonoxidative Phase Generates Ribose Precursors
Ribulose 5-phosphate is the substrate for two enzymes. Ribulose 5-phosphate 3-epimerase alters the configuration about carbon 3, forming the epimer xylulose 5-phosphate, also a ketopentose. Ribose 5-phosphate ketoisomerase converts ribulose 5-phosphate to the corresponding aldopentose, ribose 5-phosphate, which is used for nucleotide and nucleic acid synthesis. Transketolase transfers the two-carbon unit comprising carbons 1 and 2 of a ketose onto the aldehyde carbon of an aldose sugar. It therefore affects the conversion of a ketose sugar into an aldose with two carbons less and an aldose sugar into a ketose with two carbons more. The reaction requires Mg2+ and thiamin diphosphate (vitamin B1) as coenzyme. Measurement of erythrocyte transketolase and its activation by thiamin diphosphate provides an index of vitamin B1 nutritional status (Chapter 44). The two-carbon moiety transferred is probably glycolaldehyde bound to thiamin diphosphate. Thus, transketolase catalyzes the transfer of the two-carbon unit from xylulose 5-phosphate to ribose 5-phosphate, producing the seven-carbon ketose sedoheptulose 7-phosphate and the aldose glyceraldehyde 3-phosphate. These two products then undergo transaldolation. Transaldolase catalyzes the transfer of a three-carbon dihydroxyacetone moiety (carbons 1-3) from the ketose sedoheptulose 7-phosphate onto the aldose glyceraldehyde 3-phosphate to form the ketose fructose 6-phosphate and the four-carbon aldose erythrose 4-phosphate. Transaldolase has no cofactor, and the reaction proceeds via the intermediate formation of a Schiff base of dihydroxyacetone to the ε-amino group of a lysine residue in the enzyme. In a further reaction catalyzed by transketolase, xylulose 5-phosphate serves as a donor of glycolaldehyde. In this case, erythrose 4-phosphate is the acceptor, and the products of the reaction are fructose 6- phosphate and glyceraldehyde 3-phosphate.
In order to oxidize glucose completely to CO2 via the pentose phosphate pathway, there must be enzymes present in the tissue to convert glyceraldehyde 3-phosphate to glucose 6-phosphate. This involves reversal of glycolysis and the gluconeogenic enzyme fructose 1,6-bisphosphatase. In tissues that lack this enzyme, glyceraldehyde 3-phosphate follows the normal pathway of glycolysis to pyruvate.
The Two Major Pathways for the Catabolism of Glucose Have Little in Common
Although glucose 6-phosphate is common to both pathways, the pentose phosphate pathway is markedly different from glycolysis. Oxidation utilizes NADP rather than NAD, and CO2, which is not produced in glycolysis, is a characteristic product. No ATP is generated in the pentose phosphate pathway, whereas it is a major product of glycolysis.
The two pathways are, however, connected. Xylulose 5-phosphate activates the protein phosphatase that dephosphorylates the 6-phosphofructo-2-kinase/fructose 2,6-bisphophatase bifunctional enzyme (Chapter 18). This activates the kinase and inactivates the phosphatase, leading to increased formation of fructose 2,6-bisphosphate, increased activity of phosphofructokinase-1, and hence increased glycolytic flux. Xylulose 5-phosphate also activates the protein phosphatase that initiates the nuclear translocation and DNA binding of the carbohydrate response element binding protein, leading to increased synthesis of fatty acids (Chapter 23) in response to a high carbohydrate diet.
Reducing Equivalents Are Generated in Those Tissues Specializing in Reductive Syntheses
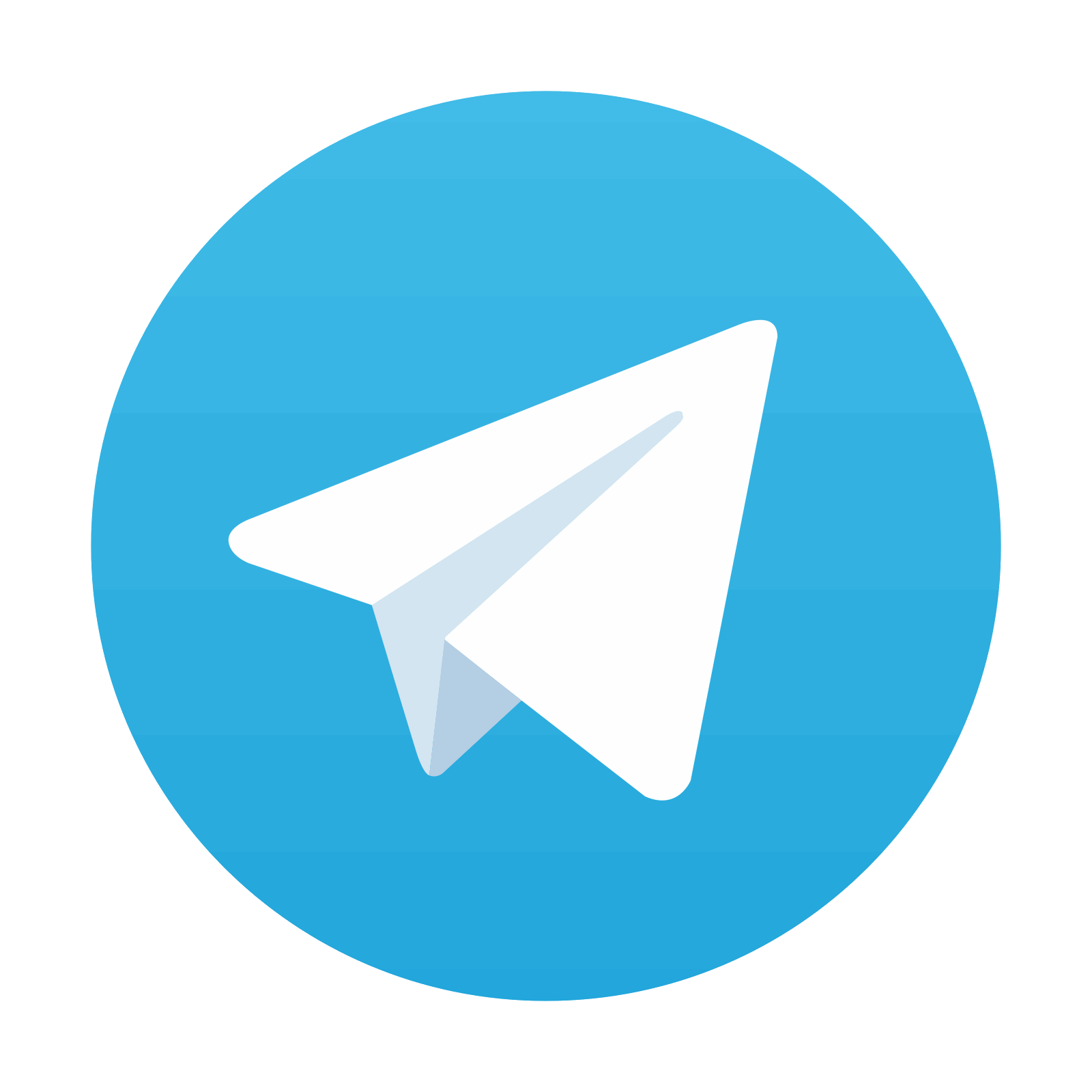
Stay updated, free articles. Join our Telegram channel

Full access? Get Clinical Tree
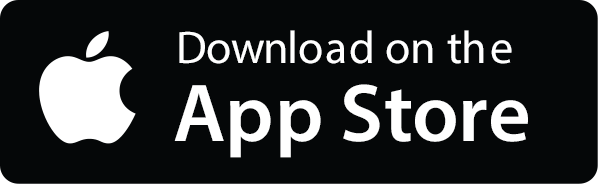
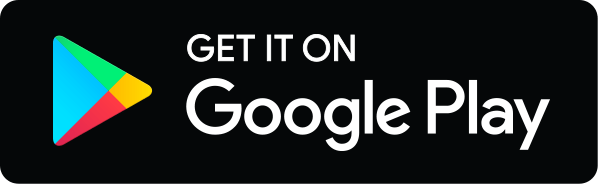