Fig. 1.1
Amputation of newt limbs leads to spontaneous regeneration. Limbs were amputated either below (left) or above the elbow (right) and were photographed at the indicated times while regenerating. (Adapted from Goss 1992)
The mammalian fetus is capable of regeneration provided it is at an early enough point along gestation (Colwell et al. 2005). Surveys of organ healing in the mammalian fetus have emphasized the absence of scarring in several animal models of injury during the early stages of gestation (Ferguson and O’Kane 2004). This topic is discussed extensively in this volume.
1.5 A Choice of Paradigm for Studies of Regeneration
The paradigm of limb amputation in the urodeles, young frogs (tadpoles), and lizards is an instance of spontaneous regeneration (Hay 1966; Goss 1969; Wallace 1981; Stocum 1995; Call and Tsonis 2005; Heber-Katz et al. 2013; Brockes and Gates 2014). In these animal models the progress of regeneration of an entire limb can be studied in detail. The injured site itself (site of limb amputation) is quite complex, reflecting loss of an entire hierarchy of tissues and organs rather than loss of a single organ. Due to the large scale of injury, study of limb amputation and its aftermath have been restricted to small amphibians and, for obvious reasons, are rarely conducted experimentally with larger animals. Furthermore, a study of spontaneous regeneration with mammals is also quite challenging, being restricted to the early fetal stage of gestation, understandably not a convenient experimental venue.
The critical distinction between studying the process of regeneration in amphibian vs. mammalian models derives from the intrinsic difference in their response to injury. In studies of amphibian limb regeneration the focus is on reversible injury. Information obtained about the detailed genetic or other pathways towards spontaneous regeneration in amphibians may eventually become applicable in future studies to the adult mammal, arguably the human, where severe injuries typically do not heal spontaneously (see further sections; Christensen et al. 2002; Menger et al. 2010; Song et al. 2010). In contrast, the emphasis in this volume is mostly on models of irreversible injury , i.e., injuries which do not regenerate spontaneously.
Two conditions stand out in such a comparison between amphibians and adult mammals. The adult mammal offers close access to the clinical goal which frequently focuses on treatment of irreversible injury; the amphibian models of reversible injury are remote from this clinical reality. Furthermore, when working with the adult mammal, the investigator is rewarded with a rich selection of experimental conditions for the study of both negative control (repair from irreversible healing) and its positive counterpart (induced regeneration by reversible healing) in the same anatomical location of a given species. It follows that study of irreversible injury in the adult mammal emerges clearly as the basic experimental paradigm for experimental studies of induced regeneration that also lead most efficiently to clinical applications.
1.6 Selection Among Mammalian Models of Organ Injury
The lion’s share of hard, quantitative evidence for induced regeneration in the adult mammal is found in studies of skin and peripheral nerve regeneration where standardized animal models and sophisticated methodology have long been in use. Selection of skin wounds and peripheral nerve wounds for study of regeneration has been deliberately made in this volume in order to take advantage of the large amount of data on healing of these two organs. The available data can then be used to derive baseline information for use in new developments and in several other organs. The author is also mostly familiar with studies of skin and peripheral nerve regeneration based on animal models and clinical applications in these two organs .
Skin wound healing in the adult mammal has been the classical paradigm of organ repair throughout history (Majno 1982). Skin is the first line of defense of the organism to exogenous insults; consequently, skin is the organ that is most often injured, either accidentally, as in a cut or a burn, or intentionally, as part of surgical operations on the internal organs. Being exposed to direct view, the healing of skin is also easier to study than is healing of internal organs. Consequently, references to “wound healing” in the literature usually imply studies of skin wounds, and “scar” typically refers to scar in skin. Studies of skin wound healing in the mammalian fetus have likewise fashioned a paradigm for spontaneous organ regeneration (Mast et al. 1992a; Stocum 1995; Colwell et al. 2005). Because of the wealth of reports in the literature concerned with skin wound healing (Peacock and Van Winkle 1976; Clark and Henson 1988; Mast 1992; Clark 1996b; Kim et al. 2009; Cherubino et al. 2011; Hassan et al. 2014), the skin wound healing paradigm forms an invaluable source of information on the macroscopic as well as molecular biological phenomena that comprise repair and suggest experimental approaches toward regeneration.
Another well-known paradigm of wound healing, studied about as frequently as skin, is peripheral nerve healing in adult mammals, often following complete transection (Lundborg 1987; Madison et al. 1992; Valentini 1995; Fu and Gordon 1997; Sanapanich et al. 2002; Dahlin et al. 2007). The data are somewhat more quantitative in this field than in skin due to morphological differences between tissues in the two organs.
The two organs, skin and peripheral nerves, are distinctly different from each other; and so are also the experimental conditions employed to study regeneration of each organ. One of my tasks in writing this volume is to demonstrate the intrinsic similarity between the process of induced regeneration in skin and in peripheral nerves. The evidence shows that certain basic spontaneous healing phenomena in these two organs are practically identical, differing primarily in their topographic anatomy; and that regeneration is induced in both of them using the same scaffold (Soller et al. 2012). This finding justifies the search for trans-organ rules, to be used in studying induced regeneration in other organs as well.
1.7 An Anatomically Well-Defined Wound
We now turn to consideration of the type of injury that will be studied in this volume .
The outcome of an injury in any organ depends profoundly on the precise nature of wound that has been generated. Investigators have studied a large variety of wound types, most of them not suited to the study of induced regeneration. In order to build a useful database from studies of several independent investigations it is necessary to select experiments that have been conducted with a type of wound that is both standardized and appropriate to the purpose of the study. For this reason, the vast majority of experimental data on spontaneous and induced regeneration described in this volume have been drawn from these investigations in which a specific type of anatomically well-defined wound, uniquely suited to the study of induced regeneration, and described in detail in a later chapter, was studied.
In order to emphasize the restrictions applied in selecting a particular kind of wound, as well as to discuss wounds in organs other than skin, I will use the term defect as shorthand to represent the anatomically well-defined wound that is appropriate for study of induced regeneration. Defects in skin and peripheral nerves will be described later in this volume. Use of this term will hopefully provide instant and relatively precise information to the reader about the anatomical site and extent of injury in which the process of interest is taking place. The term “wound” will be retained to denote the result of generalized injury without specification of the type or extent of injury .
1.8 All Organs Can Be Irreversibly Injured
The experimental evidence shows that all organs in the adult can be irreversibly injured. To support this statement we now survey the outcome of healing in a variety of tissues and organs following injury. Only studies of spontaneous healing events in adult mammals, unaided by devices and eventually leading to repair, are reviewed below.
We begin this survey with an internal organ, the liver. After surgical removal of 70 % of its mass, the liver regrows, attaining its original size often by 7–10 days. Such regrowth is recognized as an example of compensatory hypertrophy, which restores the optimal liver mass in relation to body size (Higgins and Anderson 1931; Steer 1995; Santoni-Rugiu et al. 2005; Michalopoulos 2013). In this well-studied experimental paradigm, amputation of three of five lobes of rat liver has been shown to lead to hypertrophy of the two intact lobes until the original liver cell mass has been restored (Bucher 1963). The three resected lobes never grow back (Michalopoulos and DeFrances 1997). Since the residual lobes enlarge, the restored organ has a distinctly different shape from that of the intact organ (Goss 1992; Fig. 1.2). The nature of the tissues that result from healing of each lobe at the site of amputation does not appear to have been studied extensively and questions have arisen concerning the potential of liver tissue to heal as most other organs do. However, following chronic injury of the liver with viral or toxic agents (cirrhosis), fibrous tissue is irreversibly deposited in areas where liver cells have died (Vracko 1974; Seyer and Raghow 1992; Iredale 2008). Not only in chronic injury but following acute injury as well, liver heals locally by contraction and scar formation , as in most other organs. This unexpected conclusion emerges from a study of a well-defined acute injury, the standard perforation in the mouse liver. The results have shown that the healthy liver locally responded with wound contraction and scar formation (Fig. 1.3). In another study with the healthy mouse liver an injury was inflicted that produced stromal disruption. A few days after the injury, the liver contracted around the site and a fibrotic reaction ensued (Masuzaki et al. 2013; Fig. 1.4). Although liver is often cited in the literature as the exceptional organ in adult mammals that regenerates completely and spontaneously following injury, the observations cited here (Figs. 1.3 and 1.4) define a healing process that is clearly different from spontaneous regeneration .

Fig. 1.2
Liver does not regenerate spontaneously at the anatomical site of injury. Following excision of the median and left lateral lobes of an adult rat liver (broken line shows shape of intact organ) only the caudate and the right lateral lobes remained, representing about one third of the intact organ. Three weeks after the injury the organ enlarged by compensatory hypertrophy back to a total mass equivalent to the initial mass of the uninjured liver. However, the excised lobes did not grow back. (Adapted from Goss 1992)

Fig. 1.3
The healthy adult mouse liver responds to perforation by irreversible contraction and scar formation. Top: a. Deformation of liver perimeter due to contraction after perforation with a cylindrical tool. A full-thickness injury, 3-mm diameter, was made using a biopsy punch on the left liver lobe of adult male C57BL/6 mice. The explanted liver lobe is shown 4 weeks after injury. Arrow indicates the line defect and the area of contraction resulting in a disruption of the smooth contour of the organ. Scale: Each tick mark represents 1 mm. b. Fibrosis in the contracted wound. Histology (trichrome stain) shows the fibrotic tissue (blue) that lines the edges of the full-thickness wound. Scale bar: 200 mm. Bottom: (c, d) Mechanical model of deforming liver tissue using finite element analysis, shows predicted indentation of outer tissue perimeter with increasing closure of the circular wound. (Ho et al. Suppl. Data in Soller et al. 2012)

Fig. 1.4
Contraction and scar formation in the healthy mouse liver. The injury was an incision about 1 cm long that went completely through the liver of adult male C57BL/6 mice. a Immunohistochemical staining showed no collagen type I deposition 6 h after the injury. b By 4 days, staining showed that type I collagen deposition occurred in a double line pattern flanking the injury (arrows). c By 28 days, the double line had coalesced into a single line, indicating that the parenchyma had contracted and showed a fibrotic response (scar formation). These results were confirmed using Sirius Red. (From Masuzaki et al. 2012, with permission)
Shifting our attention from the liver to the cardiovascular system, we find that the blood vessel wall can be injured not only by direct trauma but also by chronic hypertension. The formation of atherosclerotic plaque appears to be a pathologic response to intimal injury (Stemerman 1973). One of the results of such chronic abuse is accumulation of elastin and the formation of fibrotic tissue based on collagen (Davidson et al. 1992; Chapman et al. 2010). The combined effects of the repair process are increased stiffness of the blood vessel wall and stenosis, or even occlusion, of the lumen (Stemerman and Ross 1972; Davidson et al. 1992). Injuries in arteries may increase intimal thickness which is associated with interference of blood flow (Zhang et al. 2013).
In another example from the cardiovascular system, we find that cardiac muscle has been reported to be incapable of spontaneous regeneration (Polezhaev 1972; Stocum 1995). The cytology of death of heart muscle due to lack of oxygen (ischemia) has been studied extensively and the results of the healing process appear to be nearly identical to findings obtained following surgical manipulations of this tissue. In none of these cases new muscle formation was observed; fibrous tissue repair was instead observed universally (McMinn 1969). However, evidence has been also presented that human cardiac ECM appears to direct differentiation of pluripotent stem cells towards a cardiomyocyte phenotype (Oberwallner et al. 2014).
Tissues of the CNS (spinal cord, brain) are notoriously resistant to regeneration (Cajal 1928; Hay 1966; Stocum 1995). Unlike the response to injury in the peripheral nervous system (PNS), a crush injury in the spinal cord apparently is not followed by any measure of spontaneous regeneration (Eng et al. 1987; Liuzzi and Lasek 1987); instead, scar formation has been reported (Cajal 1928) and has been considered a major cause of lack of regeneration in the injured spinal cord (Kiernan 1979). It has been suggested that there is an intrinsic difference between the healing patterns of lesions in the PNS and CNS: The endoneurial tubes that are ubiquitously present in peripheral nerves, and that are often credited with the small but finite amount of regeneration in the PNS, are absent in the CNS. It has also been hypothesized that spinal axon elongation fails to occur because nonneuronal (glial) cells in the CNS lack appropriate guiding tracks (Rutka et al. 1988). Another approach emphasizes the importance of integrin attachment to their ECM ligands as a condition for upregulating axonal growth and assigns the lower regenerative capacity of the CNS to the absence of such integrins from CNS axons (Eva et al. 2012).
The musculoskeletal system offers additional examples of the irreversibility of injury. Articular cartilage, the thin but tough tissue layer that lines the surfaces of bones in a joint, lacks blood flow. Accordingly, when cartilage is injured, there is almost no evidence of the classical inflammatory response in wounds that normally supplies the defect site within minutes with blood elements as well as with a variety of growth factors and tissue cell types. The cartilage cell (chondrocyte) is the lone defender of tissue integrity in cartilage and a very limited amount of synthesis of new tissue occurs (Campbell 1969). Much more exuberant is the response to a deeper injury, extending underneath cartilage and into the subchondral bone region (Stocum 1995). An inflammatory response does in fact result from this deeper injury. Eventually, the cartilage defect is filled in with tissue that appears to be partly hyaline cartilage, suggesting synthesis of fibrocartilage, and indicating repair (Wornom and Buchman 1992). Current efforts are directed toward restoration of part of the subchondral bone, rather than restoration of articular cartilage per se (Ochi et al. 2004; Swieszkowski et al. 2007; Needham et al. 2014).
Transection of skeletal muscle has been studied extensively. The severity of injury varies widely across surgical incisions, wartime injuries and sports-related injuries. After a clean cut, sarcoplasm retracts over a short distance inside the sarcolemma, leaving an empty tube that is soon filled by invading leukocytes and macrophages. Provided that the cut ends are held closely together, budding and union of cut fibers occurs, suggesting that effective regeneration has taken place across the very short gap length (McMinn 1969). On the other hand, evidence of scar formation has been reported when the distance between the cut ends has been greater than a few millimeters (Volkman 1893; Allbrook 1962). It has been hypothesized that scar formation in the gap effectively prevents connection of muscle fibers (McMinn 1969). In contrast, injury of skeletal muscle by methods (freezing, ischemia) that killed the cells without disorganizing the matrix, was followed by repopulation of the defect with new cells and recovery of physiological structure after 3 weeks (Vracko and Benditt 1972). Contrasts in healing behavior between injuries of different severity are being studied with an eye toward understanding the cellular pathways that account for the typically degenerative processes of muscle healing (Gharaibeh et al. 2012; Shin et al. 2014).
The examples in this section illustrate the prevalence of irreversible injury in several, distinctly different, anatomical sites in the adult mammal. No organ, not even liver, is spared the irreversible loss of its structure and function at the site of injury (Fig 1.5).

Fig. 1.5
Most organs heal irreversibly, i.e., by repair rather than by regeneration, after extensive acute injury in the stroma or following chronic disease. The examples shown above illustrate repair processes in (clockwise from top left) the heart muscle, liver, eye, heart valve and kidney. (Adapted with permission from ICON Learning Systems, a subsidiary of Havas MediMedia USA Inc., original illustration by Frank H. Netter, MD. Copyright, 1977)
1.9 Nature of Injured Tissue vs. Critical Size of Defect
Consideration must now be given to the detailed type of injury in an organ that heals irreversibly. There is need to consider in some detail the size of wound that will be studied as well as the nature of tissues that will be experimentally injured. It often appears that the size of the wound, or the extent of the injury, dictates the outcome of the healing process. In a number of organs a small wound often heals reversibly while a wound in the same organ that is deep or large in volume may heal irreversibly. In this section, we will review tissues in different organs in order to answer the question: What makes an injury irreversible? Is it the size of the resulting wound or is it the identity of injured tissues?
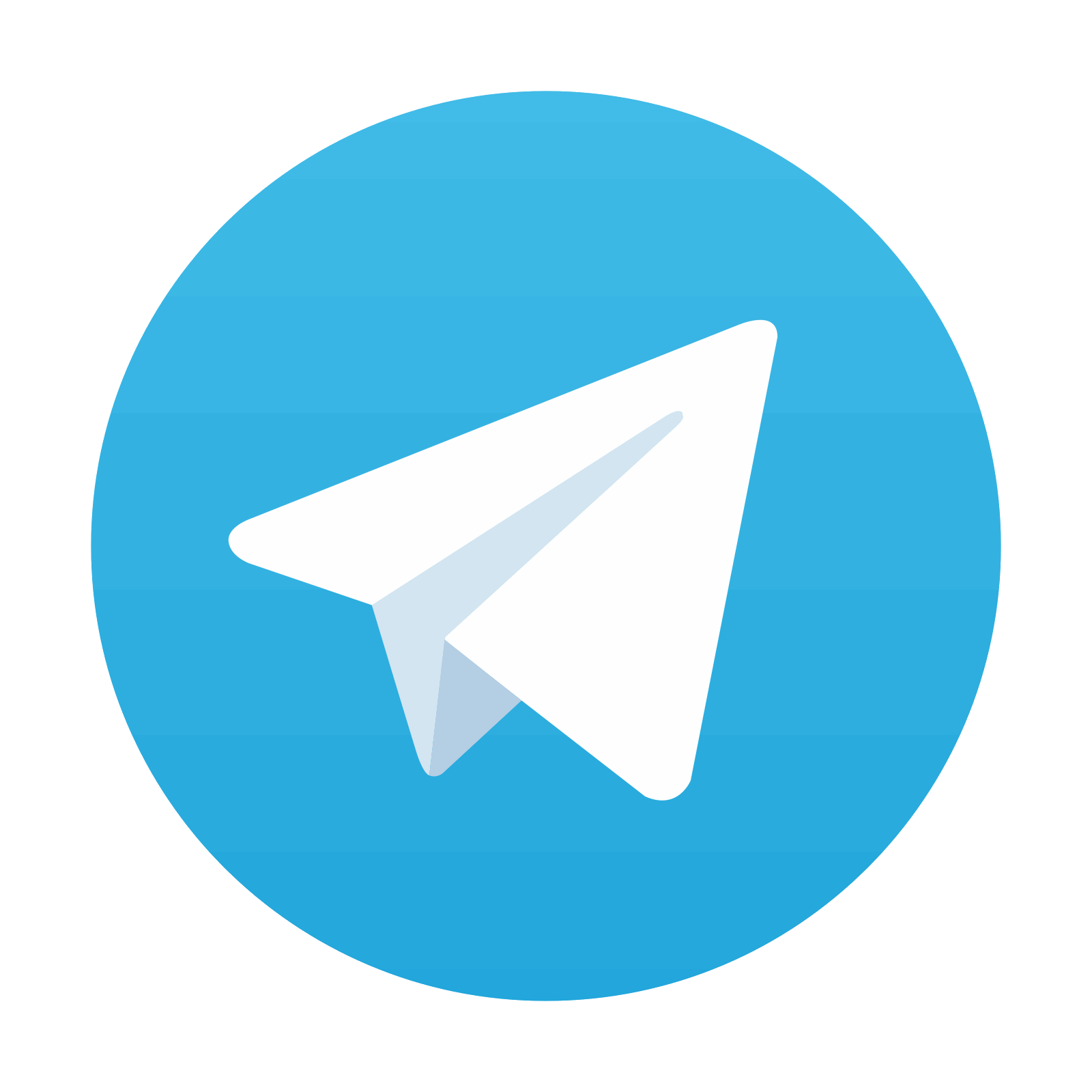
Stay updated, free articles. Join our Telegram channel

Full access? Get Clinical Tree
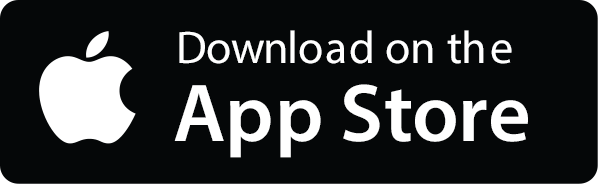
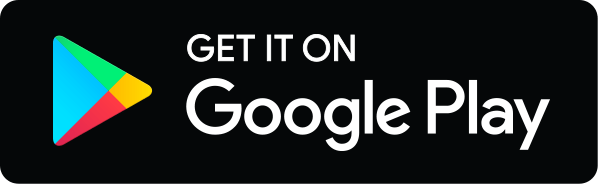