The Gastrointestinal Tract
Gastrointestinal disorders present relatively commonly to doctors, and biochemical laboratory tests have important roles in their investigation. This chapter looks at gastrointestinal pathophysiology and how chemical pathology tests may be useful in diagnosis and treatment.
PHYSIOLOGY AND BIOCHEMISTRY OF NORMAL DIGESTION
The major functions of the gastrointestinal tract are the digestion and absorption of nutrients. Additionally, it synthesizes certain hormones along its length that act locally (paracrine hormones), so controlling gut motility and the release of digestive enzymes and secretions. The gastrointestinal tract handles about 8-9 L of fluid per day, with the majority of this derived from endogenous secretions. Fluid reabsorption by the gastrointestinal tract (mainly the small intestine) is highly efficient (98 per cent), with only 100-200 mL lost daily in the stools.
Digestion and absorption depend on food and the products of its digestion being diluted with a large volume of fluid, mostly an ultrafiltrate of extracellular fluid filtered through the ‘tight junctions’ between epithelial cells, mainly in the duodenum. Some is reabsorbed in the proximal jejunum, along the osmotic gradient created by reabsorption of the products of digestion; a large amount remains in the lumen to be reclaimed more distally.
A small amount of the nutrients from desquamated intestinal cells also enters the lumen; most is reabsorbed. Almost all the fat in normal faeces is of endogenous origin, which is relevant when interpreting the results of a faecal fat estimation. A much smaller volume of fluid enters the lumen by active secretion. As a result, the pH and the electrolyte and enzyme concentrations change during the passage of fluid through the tract in such a way that the conditions for enzyme activity are near optimal for digestion. Adjustment of pH and electrolyte concentrations occurs in the distal ileum and colon; sodium reabsorption (and therefore water reabsorption) is enhanced by aldosterone in the colon. The colon can metabolize undigested carbohydrates into short-chain fatty acids such as butyrate, propionate and acetate. These are an important energy source for the colon.
Disturbances of water, electrolyte and hydrogen ion homeostasis may occur in small intestinal or colonic disease. If there is loss of fluid and electrolytes from the upper intestinal tract because of vomiting or because the function of intestinal cells is so perturbed that the amount of fluid and electrolytes entering the distal parts exceeds the reabsorptive capacity, similar changes may occur (see also Chapter 2).
Digestive enzymes usually act on complex molecules such as protein, polysaccharides and fat. This process starts in the mouth, where food is broken down by mastication and is mixed with saliva containing α-amylase. In the stomach, acid fluid is added and the low pH initiates protein digestion by pepsin. The stomach also secretes intrinsic factor, essential for vitamin B12 absorption from the terminal ileum. However, most digestion takes place in the duodenum and upper jejunum, where alkaline fluid is added to the now liquid intestinal contents. Pancreatic enzymes digest proteins to small peptides and amino acids, polysaccharides to monosaccharides, disaccharides and oligosaccharides, and fat to monoglycerides and fatty acids.
GASTRIC FUNCTION
The main components of gastric secretion are hydrochloric acid, pepsin and intrinsic factor, all of which are important for normal digestion and absorption. Loss of hydrochloric acid by vomiting, as
in pyloric stenosis, may cause a metabolic alkalosis (Chapter 4). Gastric secretion may be stimulated by the following:
in pyloric stenosis, may cause a metabolic alkalosis (Chapter 4). Gastric secretion may be stimulated by the following:
The vagus nerve, which in turn responds to stimuli from the cerebral cortex, normally resulting from the sight, smell and taste of food. Hypoglycaemia can stimulate gastric secretion and so can be used to assess the completeness of a vagotomy.
Gastrin, which is carried by the bloodstream to the parietal cells of the stomach; its action is mediated by histamine. Gastrin secretion is inhibited (by negative feedback) by acid in the pylorus. Calcium also stimulates gastrin secretion and this may explain the relatively high incidence of peptic ulceration in patients with chronic hypercalcaemia.
Histamine stimulates gastric secretion after binding to specific cell surface receptors, of which there are at least two types:
those for which antihistamines compete with histamine (H1 receptors): these are found on smooth muscle cells,
those on which antihistamines have no effect: these H2 receptors are found on gastric parietal cells.
Hypersecretion of gastric juice may cause duodenal ulceration. However, there is overlap between the amount of acid secreted in normal subjects and in those with duodenal ulceration. The estimation of gastric acidity is of very limited diagnostic value. In the very rare Zollinger-Ellison syndrome, acid secretion is very high due to excessive gastrin produced by a gastrinoma – a tumour more usually involving the pancreas or duodenum (see later).
Drugs such as ranitidine compete with histamine for the H2 receptors, so directly reducing acid secretion. Proton pump inhibitors such as omeprazole and lansoprazole have to some degree surpassed these agents.
Hyposecretion of gastric juice occurs most commonly in association with pernicious anaemia, due to the formation of antibodies to the parietal cells of the gastric mucosa. Extensive carcinoma of the stomach and chronic gastritis may also cause gastric hyposecretion. Plasma gastrin concentrations are raised, because reduced acid secretion causes the loss of negative feedback inhibition.
Tests of gastric function involving the measurement of acid secretion have largely been superseded by endoscopic examination of the stomach and duodenum and by histological examination of the biopsy material obtained.
Post-gastrectomy syndromes
There may be some degree of malabsorption after gastrectomy. Rapid passage of the contents of the small gastric remnant into the duodenum may be associated with the following:
The early dumping syndrome Soon after a meal, the patient may experience abdominal discomfort and feel faint and nauseated. These symptoms may be caused by the rapid passage of hypertonic fluid into the duodenum. Before this abnormally large load can be absorbed, water passes along the osmotic gradient from the extracellular space into the lumen. The reduced plasma volume causes faintness and the large volume of fluid causes abdominal discomfort.
The late dumping syndrome (or post-gastrectomy hypoglycaemia) If a meal containing a high glucose concentration passes quickly into the duodenum, glucose absorption is very rapid, stimulating a surge in insulin secretion. The resultant ‘overswing’ of plasma glucose concentration may cause hypoglycaemic symptoms, typically occurring about 2 h after a meal. This is a form of reactive hypoglycaemia (see Chapter 12).
Both these dumping syndromes can be minimized if frequent, small, low-carbohydrate meals are taken.
NORMAL INTESTINAL ABSORPTION
There are three phases of digestion and absorption: luminal phase, mucosal phase and post-absorptive phase.
Absorption depends on:
the presence of nutrient in an absorbable form (and therefore on normal digestion),
the integrity and large surface area of absorptive cells,
a normal ratio of the rate of absorption to the rate of passage of contents through the intestinal tract.
The absorptive area of the small intestine is very large. The mucosa forms macroscopically visible folds, increasing the area considerably. Microscopically, these folds are covered with villi lined with absorptive cells (enterocytes), which increase the area about eight-fold. A large number of minute projections (microvilli) cover the surface of each enterocyte, separated by microvillous spaces. These increase the absorptive
area about a further 20-fold. The absorptive area is significantly reduced if the villi are flattened, as they are, for example, in gluten-sensitive enteropathy.
area about a further 20-fold. The absorptive area is significantly reduced if the villi are flattened, as they are, for example, in gluten-sensitive enteropathy.
Absorption also depends on whether a molecule is:
water soluble, so that it can be absorbed either by active transport against a physicochemical gradient or by passive diffusion along gradients,
lipid soluble, enabling it to diffuse across the lipid membranes of the enterocytes.
The release of intestinal secretions and the control of gut motility are, in part, controlled by a series of peptide hormones produced in the mucosa of the gastrointestinal tract. Like other hormones, their release is under feedback control by either the product or the physiological response of the target tissue.
Cells that synthesize gut peptides are scattered throughout the length of the gastrointestinal tract, although the secretory cells are often concentrated in one segment of the tract. Many of these peptides are present in other organs, particularly the brain, where they probably act as neurotransmitters.
Gastrin is released from the gastrin-secreting G cells in the gastric antrum in response to distension and to protein. It stimulates the contraction of the stomach muscles and the secretion of gastric acid. Acid inhibits gastrin release by negative feedback.
Cholecystokinin stimulates contraction of the gall bladder and the release of pancreatic digestive enzymes.
Secretin stimulates the release of pancreatic fluid rich in bicarbonate.
Pancreatic polypeptide inhibits pancreatic secretion.
Vasoactive intestinal polypeptide (VIP) and motilin regulate gut motility.
Carbohydrate absorption
Polysaccharides, such as starch, consist either of straight chains of glucose molecules joined by 1:4 linkages, called amylose, or of branch chains, in which the branches are joined by 1:6 linkages, called amylopectin. Salivary and pancreatic α-amylases hydrolyse starch to 1:4 disaccharides such as maltose (glucose + glucose). Pancreatic amylase is quantitatively the more important. A few larger branch-chain saccharides (limit dextrans) remain undigested.
Disaccharides [maltose, sucrose (glucose + fructose) and lactose (glucose + galactose)] are hydrolysed to their constituent monosaccharides by the appropriate disaccharidases, β-galactosidases (lactase) and α-glucosidases (maltase, sucrase), located on the surface of enterocytes (‘brush border’), especially in the proximal jejunum. Sucrase also hydrolyses the 1:4, and isomaltase the 1:6, linkages of limit dextrans.
Monosaccharides are actively absorbed in the duodenum and proximal jejunum. Unlike for fructose, a common active process absorbs glucose and galactose. Carbohydrate absorption depends on:
the presence of amylase, and therefore on normal pancreatic function (polysaccharides only),
the presence of disaccharidases on the luminal membrane of intestinal cells (disaccharides),
normal intestinal mucosal cells with normal active transport mechanisms (monosaccharides).
Polysaccharides can be absorbed only if all three mechanisms are functioning.
Xylose absorption test
This can be used to test the integrity of the intestinal mucosa. Xylose is a pentose that, like glucose, can be absorbed without digestion (by facilitated diffusion), but the metabolism of which is not hormone controlled; therefore, unlike glucose, it is not affected by insulin secretion.
An oral dose of xylose is absorbed by normally functioning upper small intestinal cells. Metabolism of the absorbed pentose is very slow and, because it is freely filtered by the glomeruli, most of it appears in the urine. In the xylose absorption test, either the amount excreted in the urine during a fixed period or, in children, the plasma concentration at a defined time after the dose is measured.
Intestinal disease (for example blind loops) or impaired mucosal surface area (as in coeliac disease) may impair xylose absorption and therefore reduces excretion. Pancreatic disease does not affect either process. Therefore this test can distinguish between pancreatic insufficiency and conditions affecting the intestinal mucosa.
Unfortunately, there are several sources of error in the xylose test:
Xylose is absorbed mostly from the upper small intestine and absorption may therefore be normal despite significant ileal dysfunction, for example in Crohn’s disease.
The urine test depends on accurate collections over a short time interval and therefore even more significant errors may occur than in 24-h collections.
Mildly impaired renal function decreases glomerular filtration of xylose and may give falsely low results in the urine tests, or a falsely high plasma concentration. This is a common cause of misleading results in the elderly. The xylose absorption test should not be performed if glomerular function is even only marginally impaired.
Both plasma concentrations and urine excretion depend partly on the volume of distribution of the absorbed xylose. In oedematous or very obese patients, results may be falsely low.
A possible scheme for carrying out the xylose test is now discussed.
Procedure
A dose of 5 g of xylose is preferable to one of 25 g because a high xylose concentration within the intestinal lumen may, like a large dose of glucose, have an osmotic effect that causes symptoms and affects the results.
The patient fasts overnight.
08.00 h The bladder is emptied and the specimen discarded. Then 5 g of xylose dissolved in 200 mL of water is given orally. All specimens passed between 08.00 h and 10.00 h are put into a bottle labelled number 1.
10.00 h The bladder is emptied and the specimen is put into bottle number 1, which is now complete. All specimens passed between 10.00 h and 13.00 h are put into a bottle labelled number 2.
13.00 h The bladder is emptied and the specimen is put into bottle number 2, which is now complete. Both bottles are sent to the laboratory for analysis.
Interpretation
In the normal subject, more than 1.5 g xylose should be excreted during the 5 h. About 50 per cent or more of the total excretion should occur during the first 2 h. In mild intestinal malabsorption, the total 5-h excretion may be normal, but delayed absorption is reflected in a 2:5-h ratio of less than 40 per cent. In pancreatic malabsorption, the result should be normal.
Protein absorption
Protein in the intestinal lumen is derived from the diet, intestinal secretions and desquamated mucosal cells. Dietary protein is broken down by gastric pepsin and in the duodenum by trypsin and other proteolytic enzymes secreted in pancreatic juice; pancreatic trypsinogen is converted to active trypsin by enterokinase (enteropeptidase) located on the brush border. The products of digestion are small peptides and amino acids. Many peptides are further hydrolysed by peptidases on the brush border.
Amino acids are actively absorbed in the small intestine. Small peptides are absorbed by an active transport mechanism, independently of amino acids and, with a few exceptions, are hydrolysed intracellularly. Protein absorption depends on the presence of pancreatic proteolytic enzymes (and therefore on normal pancreatic function) and an intestinal mucosa with normal active transport mechanisms.
Lipid absorption
Digestion of fats
Triglycerides are the main form of dietary fat and are esters of glycerol with three, usually different, fatty acids. They are insoluble in water. Cholesterol, in the intestinal lumen, is derived from bile salts and from the diet; only about 30 per cent of the dietary cholesterol is absorbed (see Chapter 13).
Primary bile acids are synthesized in the liver from cholesterol, conjugated with the amino acid glycine or taurine in the liver, and then enter the intestinal lumen in the bile. In the alkaline duodenal fluid their sodium salts act as detergents, emulsifying and so facilitating the digestion and absorption of fats. Most of the bile salts are actively reabsorbed in the distal ileum, although some enter the colon where they are converted by bacteria to secondary bile salts, some of which are absorbed. The absorbed bile salts are recirculated to the liver and resecreted into the bile (the enterohepatic circulation).
Some bacteria within the gut lumen contain enzymes that catalyse the deconjugation of bile salts; unconjugated bile salts emulsify fat less effectively than conjugated ones and this may contribute to fat malabsorption. Triglycerides are emulsified by bile salts within the duodenum. They are hydrolysed by pancreatic lipase at the glycerol/fatty acid bond, primarily in positions 1 and 3. The end products are mainly 2-monoglycerides, some diglycerides and free fatty acids. Colipase, a peptide coenzyme secreted by the pancreas, is essential for lipase activity. It anchors lipase at the fat/water interface, prevents the inhibition of the enzyme by bile salts and reduces its pH optimum from about 8.5 to about 6.5; the latter is the pH in the upper intestinal lumen.
Micelle formation
Monoglycerides and free fatty acids aggregate with bile salts to form water-miscible micelles. The micelles
also contain free cholesterol liberated by the hydrolysis of cholesterol esters in the lumen and phospholipids, as well as fat-soluble vitamins (A, D, E and K). The diameter of the negatively charged micelles is between 100 and 1000 times smaller than that of the emulsion particles. Both their small size and their charge allow them to pass through the narrow microvillous spaces.
also contain free cholesterol liberated by the hydrolysis of cholesterol esters in the lumen and phospholipids, as well as fat-soluble vitamins (A, D, E and K). The diameter of the negatively charged micelles is between 100 and 1000 times smaller than that of the emulsion particles. Both their small size and their charge allow them to pass through the narrow microvillous spaces.
Within enterocytes, triglycerides are resynthesized from monoglycerides and fatty acids, and cholesterol is re-esterified. Triglycerides, cholesterol esters and phospholipids, together with fat-soluble vitamins, combine with apolipoproteins manufactured in enterocytes to form chylomicrons. These are suspended in water and pass into the lymphatic circulation.
Some short-chain and medium-chain free fatty acids pass directly through the intestinal cells into the portal bloodstream.
The absorption of lipids and fat-soluble vitamins depends on:
an adequate absorptive area of the intestinal mucosa for chylomicron formation,
the presence of bile salts,
the digestion of triglycerides by lipase, and therefore on normal pancreatic function.
Faecal fat estimation test
Faecal fat excretion should represent the difference between the fat absorbed and that entering the gastrointestinal tract from the diet and from the body. The absorption of fat and, more importantly, the addition of fat to the intestinal contents occur throughout the small intestine. A single 24-h collection of faeces gives very inaccurate results for two reasons: the transit time from the duodenum to the rectum is variable, and rectal emptying is variable and may not be complete.
It has been suggested by some experts that the faecal fat test is too inaccurate for clinical use and should be abandoned. Indeed visual inspection looking for fat globules in stools may be as sensitive and specific.
Consecutive 24-h collections give faecal fat results that may vary by several hundred per cent. The longer the period of collection, the more accurate the calculated daily mean result; ideally, stools should be collected for a minimum of 3 days.
Interpretation
A mean daily fat excretion of more than 18 mmol (5 g) indicates steatorrhoea. The result is not affected by diet within very wide limits, because in the normal subject almost all the fat is of endogenous origin. However, if the patient is on a very low-fat diet, mild steatorrhoea may be undetected.
The triolein breath test
The triolein breath test has also been used to assess fat absorption. Absorbed fat is ultimately metabolized to CO2 and water. If 14C-labelled triglyceride (triolein) is given by mouth, the ratio of 14CO2 to unlabelled CO2 can be measured in expired air. A low ratio usually indicates impaired fat absorption. The assay uses expensive isotopes and requires special expertise. Although the results correlate with faecal fat measurements, these limitations have prevented its widespread adoption.
Vitamin B12 absorption
Vitamin B12 can be absorbed only when it has formed a complex with intrinsic factor, a glycoprotein secreted by the parietal cells of the stomach (see Chapter 15). This complex is resistant to proteolytic digestion and binds to specific cell receptors in the distal ileum, from where it is absorbed. Some intestinal bacteria need vitamin B12 for growth and may prevent its absorption by competing for it with intestinal cells. Intestinal bacterial overgrowth is associated with intestinal strictures, diverticula and ‘blind loops’. Vitamin B12 absorption therefore depends on normal gastric secretion of intrinsic factor, intestinal mucosa in the distal ileum and intestinal bacterial flora.
Absorption of other water-soluble vitamins
Most of the other water-soluble vitamins (C and the B group, except B12) are absorbed in the upper small intestine, probably by specific transport mechanisms. Clinical deficiencies of all but folate are relatively uncommon in the malabsorption syndromes, probably because their absorption does not depend on that of fat. Folate deficiency may be caused by intestinal malabsorption, inadequate intake or increased use by intestinal bacteria (see Chapter 15).
Calcium and magnesium absorption
Calcium is absorbed mainly from the duodenum and upper jejunum in the free ionized form; this is enhanced by the vitamin D metabolite 1,25-dihydroxycholecalciferol, which influences both active transport and passive diffusion. Calcium absorption is impaired by the formation of insoluble salts with free fatty acids and with phosphate. Much of the faecal calcium is endogenous in origin, being derived from intestinal secretions (see Chapter 6).
An active process that may be shared with calcium also absorbs magnesium. Calcium and magnesium absorption depends on:
a low concentration of fatty acids and phosphate in the intestinal lumen,
the absorption and metabolism of vitamin D, and therefore on normal fat absorption,
normal intestinal mucosal cells.
Iron absorption
Iron is absorbed in the duodenum and upper jejunum in the ferrous form (Fe2+). Absorption is increased by anaemia of any kind and probably also by vitamin C. Some of the iron absorbed into intestinal cells enters the plasma, but some is lost into the lumen with desquamated cells (see Chapter 21).
NORMAL PANCREATIC FUNCTION
Pancreatic secretions can be divided into endocrine and exocrine components. The endocrine function and insulin and glucagon that control the plasma glucose concentration are discussed in Chapter 12. The exocrine secretions are made up of two components, the alkaline pancreatic fluid and the digestive enzymes. The alkaline fluid is primarily responsible for neutralizing gastric acid secretions, thus providing an optimal environment for duodenal digestive enzyme activity. These enzymes include the proteases trypsin, elastase and chymotrypsin, and amylase and lipase. Some of the proteases are secreted as precursors and are converted to the active form within the intestinal lumen.
Gut peptides control pancreatic secretion and are released from the duodenum in response to a rise in the hydrogen ion concentration or to the presence of food. They include secretin (which stimulates the release of a high volume of alkaline fluid) and cholecystokinin (which stimulates the release of a fluid rich in enzymes).
Tests of exocrine pancreatic function
Plasma enzymes
Plasma enzyme measurements are of limited value in assessing exocrine function. They include the following.
Amylase
This enzyme consists of two forms, one of salivary gland origin and one of pancreatic origin. In acute pancreatitis, total plasma amylase activity is usually significantly increased due to release from damaged cells.
Plasma amylase is discussed more fully in Chapter 18 in the context of enzymology. Typically, plasma amylase activity increases about five-fold in acute pancreatitis, but plasma enzyme activities of up to, and even above, this value may be reached in a number of other disorders, in particular after intestinal perforation and renal failure.
Occasionally, the plasma enzyme activity in acute pancreatitis may not be very high and usually falls rapidly as the enzyme is excreted in the urine. Consequently, a high plasma amylase activity is only a rough guide to the presence of acute pancreatitis, and normal or only slightly raised values do not exclude the diagnosis. Plasma amylase concentrations can be spuriously low or normal in acute pancreatitis evoked by severe hypertriglyceridaemia. In such circumstances a raised urinary amylase concentration may facilitate diagnosis or clearing the plasma lipaemia by centrifugation prior to assay. Haemorrhagic pancreatitis or chronic pancreatitis can also show a normal plasma amylase concentration.
Lipase
This enzyme is more specific for the pancreas and can be useful to measure if the source of a raised plasma amylase concentration is not obvious. Lipase has a longer half-life than amylase and thus may be useful in the late diagnosis of acute pancreatitis, when amylase activity can fall within the reference range.
Trypsin
This may be used to screen for cystic fibrosis (see later and Chapter 27) during the first 6 weeks of life. Blockage of pancreatic ductules by sticky mucous secretion causes high plasma trypsin concentrations. After about 6 weeks, plasma concentrations may fall as pancreatic insufficiency develops; normal levels do not exclude the diagnosis.
Duodenal enzymes
Measurement of pancreatic enzymes and the bicarbonate concentration in duodenal aspirates before and after stimulation with cholecystokinin and secretin is not very suitable for routine use because of the difficulty in positioning the duodenal tube and in quantitative sampling of the secretions.
‘Tubeless tests’ have been developed that avoid the need for intubation and that overcome the difficulties of invasive sample collection.
The PABA test
A synthetic peptide, labelled with para-aminobenzoic acid (PABA), is taken orally. After PABA has been split from the peptide by chymotrypsin, it is absorbed and excreted in the urine. Urinary excretion of PABA
is significantly reduced in chronic pancreatitis. Abnormal results may occur if there is renal glomerular dysfunction, liver disease or malabsorption, even in the presence of normal pancreatic function. The effect of these conditions is assessed by repeating the test with unconjugated PABA, which eliminates the need for digestion before absorption.
is significantly reduced in chronic pancreatitis. Abnormal results may occur if there is renal glomerular dysfunction, liver disease or malabsorption, even in the presence of normal pancreatic function. The effect of these conditions is assessed by repeating the test with unconjugated PABA, which eliminates the need for digestion before absorption.
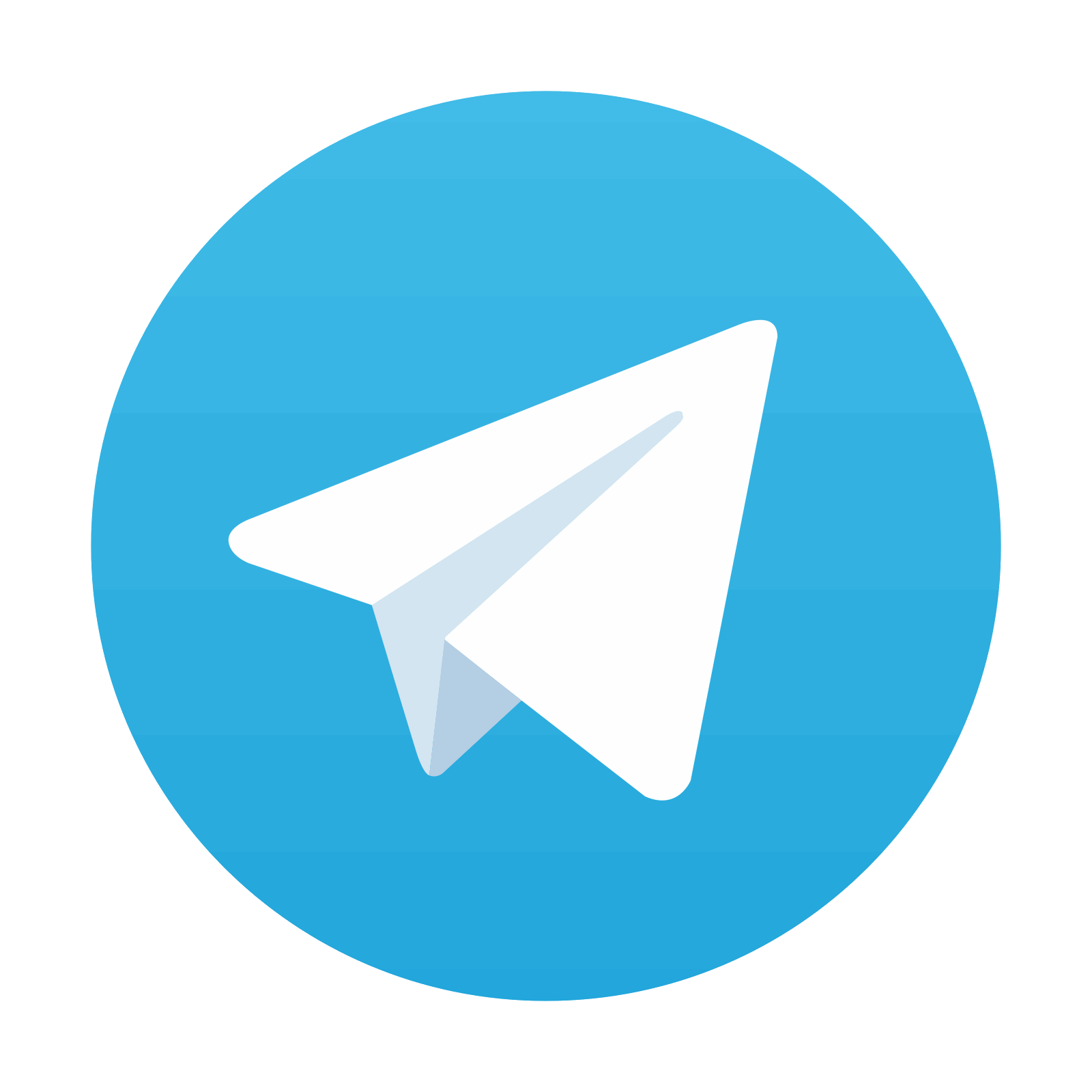
Stay updated, free articles. Join our Telegram channel

Full access? Get Clinical Tree
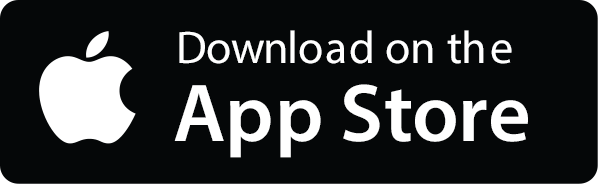
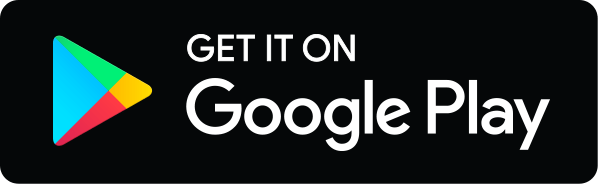
