41
The Diversity of the Endocrine System
OBJECTIVES
After studying this chapter, you should be able to:
Explain the basic principles of endocrine hormone action, including the determinants of hormone target cell response and the determinants of hormone concentration at target cells.
Understand the broad diversity and mechanisms of action of endocrine hormones.
Appreciate the complex steps involved in the production, transport, and storage of hormones.
ACTH
Adrenocorticotropic hormone
ANF
Atrial natriuretic factor
cAMP
Cyclic adenosine monophosphate
CBG
Corticosteroid-binding globulin
CG
Chorionic gonadotropin
cGMP
Cyclic guanosine monophosphate
CLIP
Corticotropin-like intermediate lobe peptide
DBH
Dopamine β-hydroxylase
DHEA
Dehydroepiandrosterone
DHT
Dihydrotestosterone
DIT
Diiodotyrosine
DOC
Deoxycorticosterone
EGF
Epidermal growth factor
FSH
Follicle-stimulating hormone
GH
Growth hormone
IGF-I
Insulin-like growth factor-I
LH
Luteotropic hormone
LPH
Lipotropin
MIT
Monoiodotyrosine
MSH
Melanocyte-stimulating hormone
OHSD
Hydroxysteroid dehydrogenase
PNMT
Phenylethanolamine-N-methyltransferase
POMC
Pro-opiomelanocortin
SHBG
Sex hormone-binding globulin
StAR
Steroidogenic acute regulatory (protein)
TBG
Thyroxine-binding globulin
TEBG
Testosterone-estrogen-binding globulin
TRH
Thyrotropin-releasing hormone
TSH
Thyrotropin-stimulating hormone
BIOMEDICAL IMPORTANCE
The survival of multicellular organisms depends on their ability to adapt to a constantly changing environment. Intercellular communication mechanisms are necessary requirements for this adaptation. The nervous system and the endocrine system provide this intercellular, organism-wide communication. The nervous system was originally viewed as providing a fixed communication system, whereas the endocrine system supplied hormones, which are mobile messages. In fact, there is a remarkable convergence of these regulatory systems. For example, neural regulation of the endocrine system is important in the production and secretion of some hormones; many neurotransmitters resemble hormones in their synthesis, transport, and mechanism of action; and many hormones are synthesized in the nervous system. The word “hormone” is derived from a Greek term that means to arouse to activity. As classically defined, a hormone is a substance that is synthesized in one organ and transported by the circulatory system to act on another tissue. However, this original description is too restrictive because hormones can act on adjacent cells (paracrine action) and on the cell in which they were synthesized (autocrine action) without entering the systemic circulation. A diverse array of hormones—each with distinctive mechanisms of action and properties of biosynthesis, storage, secretion, transport, and metabolism—has evolved to provide homeostatic responses. This biochemical diversity is the topic of this chapter.
THE TARGET CELL CONCEPT
There are about 200 types of differentiated cells in humans. Only a few produce hormones, but virtually all of the 75 trillion cells in a human are targets of one or more of the >50 known hormones. The concept of the target cell is a useful way of looking at hormone action. It was thought that hormones affected a single cell type—or only a few kinds of cells—and that a hormone elicited a unique biochemical or physiologic action. We now know that a given hormone can affect several different cell types; that more than one hormone can affect a given cell type; and that hormones can exert many different effects in one cell or in different cells. With the discovery of specific cell-surface and intracellular hormone receptors, the definition of a target has been expanded to include any cell in which the hormone (ligand) binds to its receptor, whether or not a biochemical or physiologic response has yet been determined.
Several factors determine the response of a target cell to a hormone. These can be thought of in two general ways: (1) as factors that affect the concentration of the hormone at the target cell (Table 41-1) and (2) as factors that affect the actual response of the target cell to the hormone (Table 41-2).
TABLE 41–1 Determinants of the Concentration of a Hormone at the Target Cell
TABLE 41-2 Determinants of the Target Cell Response
HORMONE RECEPTORS ARE OF CENTRAL IMPORTANCE
Receptors Discriminate Precisely
One of the major challenges faced in making the hormone-based communication system work is illustrated in Figure 41–1. Hormones are present at very low concentrations in the extracellular fluid, generally in the atto- to nanomolar range (10-15 to 10-9 mol/L). This concentration is much lower than that of the many structurally similar molecules (sterols, amino acids, peptides, and proteins) and other molecules that circulate at concentrations in the micro- to millimolar (10-6 to 10-3 mol/L) range. Target cells, therefore, must distinguish not only between different hormones present in small amounts but also between a given hormone and the 106– to 109-fold excess of other similar molecules. This high degree of discrimination is provided by cell-associated recognition molecules called receptors. Hormones initiate their biologic effects by binding to specific receptors, and since any effective control system also must provide a means of stopping a response, hormone-induced actions generally but not always terminate when the effector dissociates from the receptor (Figure 38–1; Type A response).
FIGURE 41–1 Specificity and selectivity of hormone receptors. Many different molecules circulate in the extracellular fluid (ECF), but only a few are recognized by hormone receptors. Receptors must select these molecules from among high concentrations of the other molecules. This simplified drawing shows that a cell may have no hormone receptors (1), have one receptor (2+5+6), have receptors for several hormones (3), or have a receptor but no hormone in the vicinity (4).
A target cell is defined by its ability to selectively bind a given hormone to its cognate receptor. Several biochemical features of this interaction are important in order for hormone-receptor interactions to be physiologically relevant: (1) binding should be specific, ie, displaceable by agonist or antagonist; (2) binding should be saturable; and (3) binding should occur within the concentration range of the expected biologic response.
Both Recognition & Coupling Domains Occur on Receptors
All receptors have at least two functional domains. A recognition domain binds the hormone ligand and a second region generates a signal that couples hormone recognition to some intracellular function. This coupling, or signal transduction, occurs in two general ways. Polypeptide and protein hormones and the catecholamines bind to receptors located in the plasma membrane and thereby generate a signal that regulates various intracellular functions, often by changing the activity of an enzyme. In contrast, steroid, retinoid, and thyroid hormones interact with intracellular receptors, and it is this ligand-receptor complex that directly provides the signal, generally to specific genes whose rate of transcription is thereby affected.
The domains responsible for hormone recognition and signal generation have been identified in the protein polypeptide and catecholamine hormone receptors. Steroid, thyroid, and retinoid hormone receptors have several functional domains: one site binds the hormone; another binds to specific DNA regions; a third is involved in the interaction with other coregulator proteins that result in the activation (or repression) of gene transcription; and a fourth may specify binding to one or more other proteins that influence the intracellular trafficking of the receptor.
The dual functions of binding and coupling ultimately define a receptor, and it is the coupling of hormone binding to signal transduction—so-called receptor-effector coupling— that provides the first step in amplification of the hormonal response. This dual purpose also distinguishes the target cell receptor from the plasma carrier proteins that bind hormone but do not generate a signal (see Table 41-6).
Receptors Are Proteins
Several classes of peptide hormone receptors have been defined. For example, the insulin receptor is a heterotetramer composed of two copies of two different protein subunits (α2β2) linked by multiple disulfide bonds in which the extracellular α subunit binds insulin and the membrane-spanning β subunit transduces the signal through the tyrosine protein kinase domain located in the cytoplasmic portion of this polypeptide. The receptors for insulin-like growth factor I (IGF-I) and epidermal growth factor (EGF) are generally similar in structure to the insulin receptor. The growth hormone and prolactin receptors also span the plasma membrane of target cells but do not contain intrinsic protein kinase activity. Ligand binding to these receptors, however, results in the association and activation of a completely different protein kinase signaling pathway, the JakStat pathway. Polypeptide hormone and catecholamine receptors, which transduce signals by altering the rate of production of cAMP through G-proteins, are characterized by the presence of seven domains that span the plasma membrane. Protein kinase activation and the generation of cyclic AMP (cAMP, 3′5’-adenylic acid; see Figure 19–5) is a downstream action of this class of receptor (see Chapter 42 for further details).
A comparison of several different steroid receptors with thyroid hormone receptors revealed a remarkable conservation of the amino acid sequence in certain regions, particularly in the DNA-binding domains. This led to the realization that receptors of the steroid or thyroid type are members of a large superfamily of nuclear receptors. Many related members of this family currently have no known ligand and thus are called orphan receptors. The nuclear receptor superfamily plays a critical role in the regulation of gene transcription by hormones, as described in Chapter 42.
HORMONES CAN BE CLASSIFIED IN SEVERAL WAYS
Hormones can be classified according to chemical composition, solubility properties, location of receptors, and the nature of the signal used to mediate hormonal action within the cell. A classification based on the last two properties is illustrated in Table 41-3; and general features of each group are illustrated in Table 41-4.
TABLE 41–3 Classification of Hormones by Mechanism of Action
TABLE 41–4 General Features of Hormone Classes
The hormones in group I are lipophilic. After secretion, these hormones associate with plasma transport or carrier proteins, a process that circumvents the problem of solubility while prolonging the plasma half-life of the hormone. The relative percentages of bound and free hormone are determined by the amount, binding affinity, and binding capacity of the transport protein. The free hormone, which is the biologically active form, readily traverses the lipophilic plasma membrane of all cells and encounters receptors in either the cytosol or nucleus of target cells. The ligand-receptor complex is assumed to be the intracellular messenger in this group.
The second major group consists of water-soluble hormones that bind to specific receptors spanning the plasma membrane of the target cell. Hormones that bind to these surface receptors of cells communicate with intracellular metabolic processes through intermediary molecules called second messengers (the hormone itself is the first messenger), which are generated as a consequence of the ligand-receptor interaction. The second messenger concept arose from an observation that epinephrine binds to the plasma membrane of certain cells and increases intracellular cAMP. This was followed by a series of experiments in which cAMP was found to mediate the effects of many hormones. Hormones that employ this mechanism are shown in group II.A of Table 41-3. Atrial natriuretic factor (ANF) uses cGMP as its second messenger (group II.B). Several hormones, many of which were previously thought to affect cAMP, appear to use ionic calcium (Ca2+) or metabolites of complex phosphoinositides (or both) as the intracellular second messenger signal. These are shown in group II.C of the table. The intracellular messenger for group II.D is a protein kinase-phosphatase cascades; several have been identified, and a given hormone may use more than one kinase cascade. A few hormones fit into more than one category, and assignments change as new information is discovered.
DIVERSITY OF THE ENDOCRINE SYSTEM
Hormones Are Synthesized in a Variety of Cellular Arrangements
Hormones are synthesized in discrete organs designed solely for this specific purpose, such as the thyroid (triiodothyronine), adrenal (glucocorticoids and mineralocorticoids), and the pituitary (TSH, FSH, LH, growth hormone, prolactin, ACTH). Some organs are designed to perform two distinct but closely related functions. For example, the ovaries produce mature oocytes and the reproductive hormones estradiol and progesterone. The testes produce mature spermatozoa and testosterone. Hormones are also produced in specialized cells within other organs such as the small intestine (glucagon-like peptide), thyroid (calcitonin), and kidney (angiotensin II). Finally, the synthesis of some hormones requires the parenchymal cells of more than one organ—eg, the skin, liver, and kidney are required for the production of 1,25(OH)2-D3 (calcitriol). Examples of this diversity in the approach to hormone synthesis, each of which has evolved to fulfill a specific purpose, are discussed below.
Hormones Are Chemically Diverse
Hormones are synthesized from a wide variety of chemical building blocks. A large series is derived from cholesterol. These include the glucocorticoids, mineralocorticoids, estrogens, progestins, and 1,25(OH)2-D3 (Figure 41–2). In some cases, a steroid hormone is the precursor molecule for another hormone. For example, progesterone is a hormone in its own right but is also a precursor in the formation of glucocorticoids, mineralocorticoids, testosterone, and estrogens. Testosterone is an obligatory intermediate in the biosynthesis of estradiol and in the formation of dihydrotestosterone (DHT). In these examples, described in detail below, the final product is determined by the cell type and the associated set of enzymes in which the precursor exists.
FIGURE 41–2 Chemical diversity of hormones: (A) cholesterol derivatives; (B) tyrosine derivatives; (C) peptides of various sizes; (D) glycoproteins (TSH, FSH, and LH) with common α subunits and unique β subunits.
The amino acid tyrosine is the starting point in the synthesis of both the catecholamines and thyroid hormones tetraiodothyronine (thyroxine; T4) and triiodothyronine (T3) (Figure 41–2). T3 and T4 are unique in that they require the addition of iodine (as I–) for bioactivity. Since dietary iodine is very scarce in many parts of the world, an intricate mechanism for accumulating and retaining I– has evolved.
Many hormones are polypeptides or glycoproteins. These range in size from the small thyrotropin-releasing hormone (TRH), a tripeptide, to single-chain polypeptides like adrenocorticotropic hormone (ACTH; 39 amino acids), parathyroid hormone (PTH; 84 amino acids), and growth hormone (GH; 191 amino acids) (Figure 41–2). Insulin is an AB chain heterodimer of 21 and 30 amino acids, respectively. Follicle-stimulating hormone (FSH), luteinizing hormone (LH), thyroid-stimulating hormone (TSH), and chorionic gonadotropin (CG) are glycoprotein hormones of αβ heterodimeric structure. The α chain is identical in all of these hormones, and distinct β chains impart hormone uniqueness. These hormones have a molecular mass in the range of 25-30 kDa depending on the degree of glycosylation and the length of the β chain.
Hormones Are Synthesized & Modified for Full Activity in a Variety of Ways
Some hormones are synthesized in final form and secreted immediately. Included in this class are hormones derived from cholesterol. Some, such as the catecholamines are synthesized in final form and stored in the producing cells, while others, like insulin, are synthesized from precursor molecules in the producing cell, and then are processed and secreted upon a physiologic cue (plasma glucose concentrations). Finally, still others are converted to active forms from precursor molecules in the periphery (T3 and DHT). All of these examples are discussed in more detail below.
MANY HORMONES ARE MADE FROM CHOLESTEROL
Adrenal Steroidogenesis
The adrenal steroid hormones are synthesized from cholesterol, which is mostly derived from the plasma, but a small portion is synthesized in situ from acetyl-CoA via mevalonate and squalene. Much of the cholesterol in the adrenal is esterified and stored in cytoplasmic lipid droplets. Upon stimulation of the adrenal by ACTH, an esterase is activated, and the free cholesterol formed is transported into the mitochondrion, where a cytochrome P450 side chain cleavage enzyme (P450scc) converts cholesterol to pregnenolone. Cleavage of the side chain involves sequential hydroxylations, first at C22 and then at C20, followed by side chain cleavage (removal of the six-carbon fragment isocaproaldehyde) to give the 21-carbon steroid (Figure 41–3, top). An ACTH-dependent steroidogenic acute regulatory (StAR) protein is essential for the transport of cholesterol to P450scc in the inner mitochondrial membrane.
FIGURE 41–3 Cholesterol side-chain cleavage and basic steroid hormone structures. The basic sterol rings are identified by the letters A-D. The carbon atoms are numbered 1-21, starting with the A ring.
All mammalian steroid hormones are formed from cholesterol via pregnenolone through a series of reactions that occur in either the mitochondria or endoplasmic reticulum of the producing cell. Hydroxylases that require molecular oxygen and NADPH are essential, and dehydrogenases, an isomerase, and a lyase reaction are also necessary for certain steps. There is cellular specificity in adrenal steroidogenesis. For instance, 18-hydroxylase and 19-hydroxysteroid dehydrogenises, which are required for aldosterone synthesis, are found only in the zona glomerulosa cells (the outer region of the adrenal cortex), so that the biosynthesis of this mineralocorticoid is confined to this region. A schematic representation of the pathways involved in the synthesis of the three major classes of adrenal steroids is presented in Figure 41–4. The enzymes are shown in the rectangular boxes, and the modifications at each step are shaded.
FIGURE 41–4 Pathways involved in the synthesis of the three major classes of adrenal steroids (mineralocorticoids, glucocorticoids, and androgens). Enzymes are shown in the rectangular boxes, and the modifications at each step are shaded. Note that the 17α-hydroxylase and 17,20-lyase activities are both part of one enzyme, designated P450c17. (Slightly modified and reproduced, with permission, from Harding BW: In: Endocrinology, vol 2. DeGroot LJ (editors). Grune & Stratton, 1979. Copyright © 1979 Elsevier Inc. Reprinted with permission from Elsevier.)
Mineralocorticoid Synthesis
Synthesis of aldosterone follows the mineralocorticoid pathway and occurs in the zona glomerulosa. Pregnenolone is converted to progesterone by the action of two smooth endoplasmic reticulum enzymes, 3β-hydroxysteroid dehydrogenase (3β-OHSD) and Δ5,4-isomerase. Progesterone is hydroxylated at the C21 position to form 11-deoxycorticosterone (DOC), which is an active (Na+-retaining) mineralocorticoid. The next hydroxylation, at C11
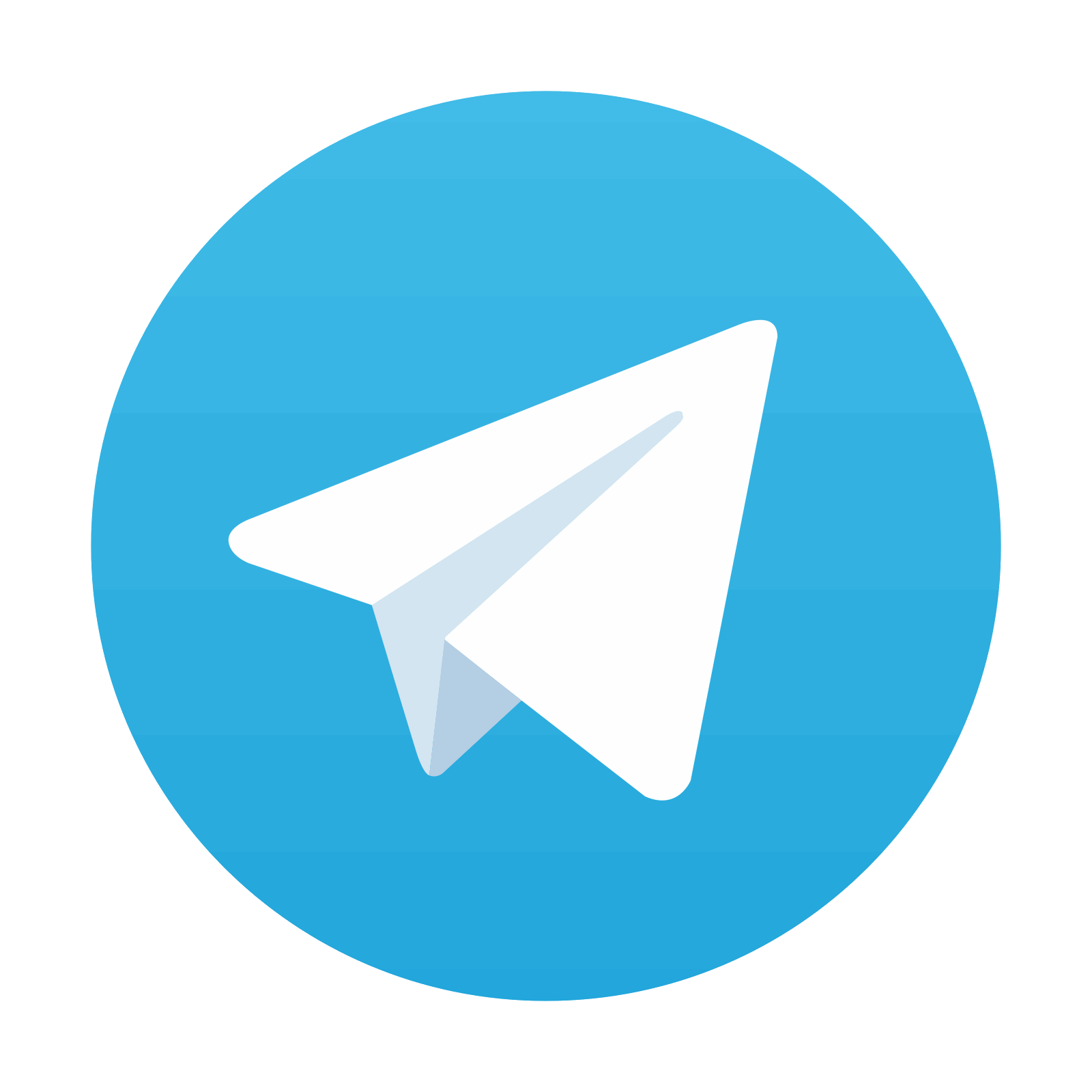
Stay updated, free articles. Join our Telegram channel

Full access? Get Clinical Tree
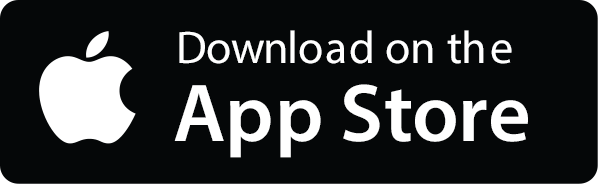
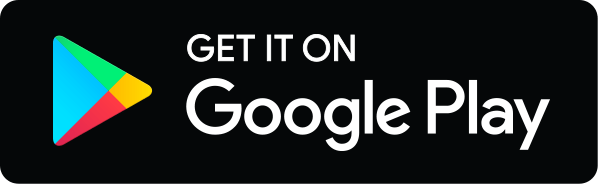