The Core Model: Drug Discovery and Development via Effective Translational Science and Public–Private Collaboration
The Core Model Corporation, S.A., Panama City, Panama
Drug discovery and development via collaboration is as old as the pharmaceutical industry itself [1]. Since its beginnings back in the middle to late 1800s, the pharmaceutical industry has established extremely close and productive research relationships with universities and research institutes in France, England, Germany, Japan, and the United States, among other nations, which provided industry with an immense wealth of knowledge, ideas, and innovation that were translated by industry into important and revolutionary medical products [2]. Ever since, the impact of academic discoveries has influenced every aspect of drug development, from the initial identification of targets to the understanding of biochemical and genetic pathways within the cell; from the elucidation of mechanistic paradigms involved in specific diseases to the creation of diagnostic tools and biomedically oriented devices. Academia has pioneered scientific knowledge, complemented, and validated the more focused, sophisticated, and refined work that is performed in industry; while industry, on the other hand, has always taken basic scientific research to the next level, to applied science or technology, therefore creating great health care and social and economic benefits for society.
During the last few decades, the influence of academic research has become vital to the biopharmaceutical industry, as exemplified by the creation and growth of the biotechnology industry as well as by the way in which long-established pharmaceutical companies are developing new pharmaceuticals, capitalizing on cutting-edge technologies and discoveries that saw daylight in academic settings (e.g., genomics, postgenomics, proteomics tools, and nanotechnology). The biotechnology tools that have been created in academia and further developed in industry have facilitated a better and unprecedented knowledge of how the cell works at the atomic and molecular levels, which has had a tremendous impact on our understanding of diseases and the creation of novel drugs. A remarkable example is the creation of monoclonal antibodies, a work that started out in academia and that later revolutionized medicine when optimized and applied by industry. Though the pioneering work on monoclonal antibodies in, say, cancer drugs, such as alemtuzumab, imatinib mesylate, cetuximab, trastuzumab, and more recently, bevacizumab (see Table 35.1), was performed in academia, none would have become therapeutic applications if industry had not taken the financial risk to develop them and contribute their know-how and marketing capabilities. Furthermore, these drugs would not have come to the market if it had not been for a very symbiotic relationship, a constant flow of scientists, ideas, experimental results, and materials, between the academic laboratories where these antibodies were originally invented and engineered and the respective companies that optimized and developed them further.
TABLE 35.1. Examples of Monoclonal Antibodies Approved by the Food and Drug Administration


In the last few years, the pharmaceutical industry has been facing many remarkable challenges, such as a significant decrease in productivity in spite of the ever increasing investment in research and development (R&D), loss of marketing exclusivity of some important and highly profitable drugs due to massive patent expirations, major safety setbacks with drugs in development or already in the market, higher regulatory hurdles for drug approval, poor public image, and so on. Accordingly, the pharmaceutical industry has responded to this crisis by adopting a series of strategies, such as increase of price of medicines, patent extension, reformulation, severe cuts in R&D and labor, mergers and acquisitions—none of which can sustain growth in the medium and long-terms, and which, unfortunately, have severe direct and indirect effects in the pockets of patients worldwide. In fact, the last wave of mergers in the recent years has compounded, instead of solving, the pharmaceutical industry’s problems; because, these mergers create not only more managerial layers but also great disruptions in the cultures and programs of these large firms. As a result, it will be impossible to manage them directly from the top, as used to be done, or in a “closed innovation” fashion, in which ideas in these companies were generated internally and taken from concept to commercialization using vertically integrated resources. Instead, an “open innovation” model—one in which new product innovation originates from both internal and external ideas—has become a major component in how drug discovery is being executed by the pharmaceutical industry in recent years.
In addition to the academia-industry relationship, other three components, namely government agencies, public and private funders/investors, and advocacy/philanthropic organizations have played a very important role in drug discovery and development. These elements deserve some discussion and some credit, as the drug creation machinery would not work without them.
To illustrate the great potential that academia–industry/public–private collaboration has in bringing an important drug to the marketplace, this chapter discusses the development of anti-cancer drug bortezomib (Velcade; Millennium Pharmaceuticals) by a biotechnology company—Myogenics/ProScript—started by academics from Harvard University, and the effective use of translational research in this process. I dissect the key academia-industry/public sector-private sector interactions that made the development of this drug a success despite many barriers and offer a model to explain how and why bortezomib was approved in record time. Areas for public–policy initiatives to improve translational research in general are highlighted. The chapter concludes with other examples of public–private sector interactions in drug research that have had varying degrees of success, as some lessons can be learned from them too.
Success in Translational Research: Lessons from the Development of Bortezomib
Success in bringing novel drugs to the market is not only a function of the quality of the science, but also of the collaboration between academia and industry, the organization and management of R&D, the public policies that regulate scientific research, and the personal connections among key players. With the aim of understanding the key factors underlying success in drug discovery and development, here we focus on the effective use of translational research and the key academia–industry/public–private interactions in the development of bortezomib (Velcade) [3], an innovative anticancer drug for the treatment of multiple myeloma created by a company started by academics from Harvard University, Myogenics—later renamed as ProScript and then acquired by LeukoSite and Millennium Pharmaceuticals, respectively. A model to explain how and why bortezomib was approved in record time is presented, and areas for public-policy initiatives to improve drug discovery and translational research in general are highlighted.
Cancer is the second-leading cause of death in the United States after heart disease, and the amount of funding that goes into cancer research—public and private—is higher than for any other disease [4–6]. But despite private and public efforts, the process of bringing effective cancer drugs into the market still faces many challenges. Thus, anticancer research and drug development provides an excellent illustration of the relationships and interactions between: basic, applied and translational research (see Box 35.1 for definitions); academia and industry; and public and private funding. The analysis here focuses on the route leading to the proof-of-principle of the therapeutic potential of bortezomib in humans to probe the key interactions between early-stage academic biotech spin-offs, academia, industry, government agencies, public and private investors and advocacy groups.
Translational Research
Translational research is particularly interesting, because it gains from and provides valuable information to both basic and applied research (Box 35.1). In addition, translational research has the potential to promote the creation of new potential therapeutics that are riper for commercialization, thereby decreasing economic risks (due to possible failure) and development costs.
At present, there is a funding gap, at least in academia, for translational research, and especially for clinical trials. So there could be a significant number of projects and potential drugs that have shown promise in preclinical studies that are languishing in academic settings because of the lack of adequate funding to take them into the clinic. (For a more thorough listing of project areas that might inform about a gap in funding for translational research, please see the Rapid Access to Intervention Development (RAID) website in Further information, and for discussion of the Developmental Therapeutics Program at the NCI, see [7]). In many cases, these drugs may not taken up by pharmaceutical or biotech companies because they are not economically or strategically viable, or simply because they neither target large markets nor promise high returns. However, initial approval for smaller markets, while providing benefits to patients, could open the doors for later approvals in larger, and therefore more profitable, markets and provide the necessary validation for “first-in-class” drugs that can be used in several other medical applications. Moreover, trials funded by smaller biotechnology companies could potentially be carried out more effectively if additional funding for translational studies were available. Realistically, small biotechnology companies can barely afford to develop one or two products at the same time, and additional basic or translational research cannot be carried out without the risk of running out of funds before subsequent rounds of funding (see Box 35.2, note 1). It is, therefore, important to understand the impediments that translational research encounters today, and whether there are adequate incentives to invest or participate in it.
The Bayh–Dole Act of 1980 entitles U.S. universities to the intellectual property rights of discoveries made using federal funding. The rationale behind this act was to increase patenting of discoveries and the acceleration of economic growth through the creation of “high-tech” firms that license these technologies from the university. However, licensing these technologies does not necessarily mean that more funding has been channeled into their translation into concrete commercial products (see [8] for a description of the impact of the Bayh–Dole Act). In addition, although the involvement of academic scientists in translational research and entrepreneurial activities may be more evident, even fostered, in areas such as biotech and high-tech “clusters,” these clusters are exceptions; their formation is a complex, expensive and lengthy process and their impact on economic acceleration and growth is unknown or, at least, not easily measurable. In many respects, in the United States and in Europe, the participation of academic scientists in commercial activities may still be seen as a distraction or deviation from their academic duties, and many academic scientists prefer not to leave university circles to pursue opportunities as entrepreneurs, because of the risks involved, economic and otherwise.
Intellectual property rights, although doubtless necessary, can also be an impediment in the process of translating basic science into commercial products. Of course, most companies and investors will not finance translational science and develop drugs without a strong intellectual property position to protect their investment. But after collaboration conversations have started, the terms and conditions of the licensing and technology transfer agreements can often reach absurd levels for either or both parties, and interesting and highly viable projects can reach an impasse, slowing down the translational process. Indeed, there is a perception among people working in academic and biotechnology spin-off companies that a significant amount of the obstacles that they encounter come from the universities themselves, especially when dealing with issues related to ownership and economic dividends.
So, how did bortezomib, a first-in-class drug that originated in an academic biotechnology spin-off, make it to market so rapidly? This is a particularly interesting question considering the fragile funding base of its originator, the considerable obstacles (internal and external) encountered, and the risks involved in pursuing a new molecular target—the proteasome—with a new and ill-famed class of inhibitor (boronates; see Box 35.2, note 2).
Properties of Bortezomib
Bortezomib (also known as MG-341/PS-341/LDP-341/ML-341) is a first-in-class proteasome inhibitor for the treatment of multiple myeloma, an incurable cancer of the blood (see Box 35.2, note 3). The proteasome is a hollow and cylindrical enzymatic complex present in both the cytoplasm and the nucleus of all eukaryotic cells and is necessary for the degradation of over 80% of the cell’s proteins. This is an important step in the regulation of other cellular functions, including signal transduction pathways that regulate cell growth and proliferation [9–11].
In blocking the proteasome’s catalytic active site, bortezomib inhibits an important cellular mechanism that regulates the cell cycle via the activation of nuclear factor-κB (NF-κB) [11–13] (Figure 35.1). Preventing NF-κB activation leads to apoptosis and renders malignant cells more vulnerable to chemotherapy and radiation [11]. Although not a cure for multiple myeloma, bortezomib has had significant effects in prolonging the life of patients who received at least two prior therapies and demonstrated disease progression on the last therapy [14, 15] (see also Multiple Myeloma Research Foundation in Further Information). The following is a short and simplified account of how this drug came to the market.

Discovery and Development of Bortezomib
In 1992, Alfred Goldberg decided to use the growing basic knowledge on the proteasome to create a biotechnology company focused on one goal: using inhibitors to block the proteasome, with the aims of investigating the physiological roles of the proteasome and translating basic proteasome research into a therapeutic application (the history of proteasome research is far too extensive for this article; see reference [16] for a description of the history and key players). The company, Myogenics, was founded in 1993, and its objective was to target the ubiquitin-proteasome pathway to slow down the process of muscle wasting (cachexia) associated with fast protein degradation.
To create the company, Goldberg formed partnerships with two scientists at Harvard: Kenneth Rock, an immunologist and pathologist who had collaborated with Goldberg on studies of proteasome and antigen presentation; and Michael Rosenblatt, who brought a wealth of experience in drug development. Tom Maniatis, co-discoverer of the NF-κB pathway, who collaborated with Goldberg on gene transcription linked to the proteasome [12], subsequently also joined.
In 1993, the company hired its first CEO, Frans Stassen, who came from Ciba-Geigy and had significant experience in drug development. A team of enzymologists (led by Ross Stein, who came from Merck) was also employed, and created the first inhibitors of the proteasome, which would eventually lead to bortezomib: peptide aldehyde analogues of the favored substrates of the proteasome’s chymotrypsin-like active site [17]. These inhibitors including MG132, which is still widely used in basic research, were distributed freely to many academic researchers. Next, Julian Adams (who was hired from Boehringer as Head Chemist, and who later became Executive Vice President of R&D) and the team of chemists that he led used, between 1994 and 1995, a straightforward medicinal chemistry approach to create a dipeptide boronate, named MG-341 (Ref. [18]) and other inhibitors such as MG-519/PS-519.
An important characteristic of Myogenics was its extremely close collaboration with academia, in which important scientific knowledge on the proteasome and the pathways involved in inflammation and apoptosis was quickly created via productive partnership between several of the founders at Harvard and the scientists in the company. For example, the first inhibitors created by the company were immediately tested on cells in the laboratories of Goldberg and Rock, and this research afforded insights into the effect of proteasome inhibition on inflammation.
One of the initial key findings from the laboratories of Rock and Goldberg was that blocking the proteasome in vivo did not immediately alter the normal life of the cell immediately [16]. Ensuing studies carried out in collaboration between Goldberg and Maniatis’s laboratory at Harvard, such as the discovery by Vito Palombella and colleagues that the proteasome is very important in the activation of NF-κB [12], which is in turn involved in the inflammatory response [19], led to a change of focus in the company: from muscle wasting to inflammation, and a corresponding change in company name, from Myogenics to ProScript. In August 1994, Avram Hershko suggested to Adams that the company investigate cancer as a potential disease target (J. Adams, personal communication), and investigations carried out by the company scientists showed that the proteasome inhibitors blocked the proliferation of cancer cells in vitro, bearing in mind the role of NF-κB in gene regulation. This strongly increased the interest in cancer, although the company continued to be focused on inflammation.
At this time, there was considerable tension between the founders, the company scientists and the Scientific Advisory Board (SAB) owing to differences in opinion on the direction and strategies that the company should pursue: muscle wasting, inflammation or cancer. A step forward in the cancer direction was the establishment of a collaboration between ProScript and Beverly Teicher from the Dana Farber Cancer Institute. Teicher was introduced to the company by Bruce Zetter (Harvard), who had been, in turn, introduced by Goldberg to the company as a SAB member. The group at ProScript then initiated their first proof-of-concept study in cancer in collaboration with Teicher in 1995. At this time, Teicher was studying angiogenesis and the role of toxic agents such as alkylating agents in cancer cells and provided ProScript with the first tumor mice models. By 1997, the group had shown that bortezomib, known as PS-341 at the time, inhibits tumor growth and metastasis in a mouse model of lung cancer; the results were published in 1999 [20]. However, there was considerable skepticism about the drug based on what was considered its potential toxicity in humans.
In addition, Adams, through Maniatis, met David Livingston, a leading figure in the mechanistic field of oncology, and asked him to join ProScript’s SAB. Livingston’s role was crucial in steering the company to thought leaders in the cancer field, such as Kenneth Anderson from Dana Farber. At the end of 1995, ProScript established a collaboration agreement with Hoechst Marion Roussel (HMR) to develop orally active anti-inflammatory and anticancer agents based on ProScript’s ubiquitin-proteasome inhibition technology (see Box 35.2, note 4). A year later the company signed a drug discovery/development collaboration with Hoffmann-La Roche (Nippon Roche) on compounds to treat cachexia, muscle wasting associated with cancer (see Box 35.2, note 5). These important collaborations were secured by ProScript’s second CEO, Richard Bagley, who was crucial not only in establishing these collaborations but also in further negotiations between ProScript and these companies, including the recovery of rights related to proteasome inhibitors from HMR.
Between 1996 and 1997, ProScript approached the NCI for collaboration. During this period, the NCI was interested in looking for new chemotherapeutic agents and had a large collection of cell lines in which PS-341 could be tested. This was a direct collaboration with Edward Sausville, Head of the Developmental Therapeutics Program (DTP) at NCI and Chair of the NCI Decision Network (the body that makes decisions on the commitment of NCI funds to new drug development initiatives arising either from NCI or from outside) and his team. After this collaboration and the initial data that resulted from it, the company started to focus increasingly on cancer, although it continued to pursue inflammation as well (see Box 35.2, note 6).
Through 1997, ProScript continued to collect animal data and established collaborations with several academic researchers [21], among them, Christopher Logothetis, Chairman of Genitourinary Oncology at the MD Anderson Cancer Center (MDACC). Logothetis was introduced to ProScript by David McConkey (MD Anderson) and was invaluable early on for the progression of ProScript’s trials (P. Elliott, personal communication). Through Logothetis, ProScript met Howard Soule, Chief of Science Officer at CaP Cure, now the Prostate Cancer Foundation.
After encouraging results from the NCI and animal studies carried out by the pharmacology group led by Elliott at ProScript [22], the company won unanimous approval and funding from the NCI, CaP Cure and two academic groups, the Memorial Sloan Kettering Cancer Center (MSKCC) and the University of North Carolina (UNC) to conduct Phase 1 clinical trials. After successful initiation of the Phase 1 trial at MDACC, two additional trials were started. The first was at Memorial Sloan Kettering with David Spriggs and the second was with Robert Orlowski at UNC, who was introduced to the company by James Cusack and Albert Baldwin (UNC). Both trials were funded by the individual institutions, which shows that it is possible to find external funding for trials of promising drugs and thereby avoid the rapid depletion of the limited resources of a small biotechnology company. The trial at UNC focused on hematological malignancies and it was this trial that demonstrated (in 2000) that bortezomib was active in multiple myeloma [23] (see Box 35.3, note 1).

Full access? Get Clinical Tree
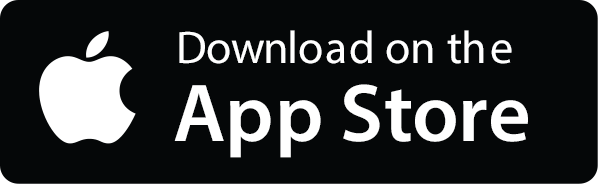
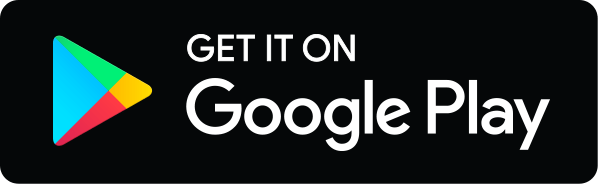