17
The Citric Acid Cycle: The Catabolism of Acetyl-CoA
David A. Bender, PhD & Peter A. Mayes, PhD, DSc
OBJECTIVES
After studying this chapter, you should be able to:
Describe the reactions of the citric acid cycle and the reactions that lead to the production of reducing equivalents that are oxidized in the mitochondrial electron transport chain to yield ATP.
Explain the importance of vitamins in the citric acid cycle.
Explain how the citric acid cycle provides both a route for catabolism of amino acids and also a route for their synthesis.
Describe the main anaplerotic pathways that permit replenishment of citric acid cycle intermediates, and how the withdrawal of oxaloacetate for gluconeogenesis is controlled.
Describe the role of the citric acid cycle in fatty acid synthesis.
Explain how the activity of the citric acid cycle is controlled by the availability of oxidized cofactors.
Explain how hyperammonemia can lead to loss of consciousness.
BIOMEDICAL IMPORTANCE
The citric acid cycle (Krebs cycle, tricarboxylic acid cycle) is a sequence of reactions in mitochondria that oxidizes the acetyl moiety of acetyl-CoA and reduces coenzymes that are reoxidized through the electron transport chain, linked to the formation of ATP.
The citric acid cycle is the final common pathway for the oxidation of carbohydrate, lipid, and protein because glucose, fatty acids, and most amino acids are metabolized to acetyl-CoA or intermediates of the cycle. It also has a central role in gluconeogenesis, lipogenesis, and interconversion of amino acids. Many of these processes occur in most tissues, but liver is the only tissue in which all occur to a significant extent. The repercussions are therefore profound when, for example, large numbers of hepatic cells are damaged as in acute hepatitis or replaced by connective tissue (as in cirrhosis). The few genetic defects of citric acid cycle enzymes that have been reported are associated with severe neurological damage as a result of very considerably impaired ATP formation in the central nervous system.
Hyperammonemia, as occurs in advanced liver disease, leads to loss of consciousness, coma, and convulsions as a result of impaired activity of the citric acid cycle, leading to reduced formation of ATP. Ammonia both depletes citric acid cycle intermediates (by withdrawing α-ketoglutarate for the formation of glutamate and glutamine) and also inhibits the oxidative decarboxylation of α-ketoglutarate.
THE CITRIC ACID CYCLE PROVIDES SUBSTRATE FOR THE RESPIRATORY CHAIN
The cycle starts with reaction between the acetyl moiety of acetyl-CoA and the four-carbon dicarboxylic acid oxaloacetate, forming a six-carbon tricarboxylic acid, citrate. In the subsequent reactions, two molecules of CO2 are released and oxaloacetate is regenerated (Figure 17–1). Only a small quantity of oxaloacetate is needed for the oxidation of a large quantity of acetyl-CoA; it can be considered as playing a catalytic role, since it is regenerated at the end of the cycle.
FIGURE 17–1 The citric acid cycle, illustrating the catalytic role of oxaloacetate.
The citric acid cycle is an integral part of the process by which much of the free energy liberated during the oxidation of fuels is made available. During the oxidation of acetyl-CoA, coenzymes are reduced and subsequently reoxidized in the respiratory chain, linked to the formation of ATP (oxidative phosphorylation, Figure 17–2; see also Chapter 13). This process is aerobic, requiring oxygen as the final oxidant of the reduced coenzymes. The enzymes of the citric acid cycle are located in the mitochondrial matrix, either free or attached to the inner mitochondrial membrane and the crista membrane, where the enzymes and coenzymes of the respiratory chain are also found (Chapter 13).
FIGURE 17–2 The citric acid cycle: the major catabolic pathway for acetyl-CoA in aerobic organisms. Acetyl-CoA, the product of carbohydrate, protein, and lipid catabolism, is taken into the cycle and oxidized to CO2 with the release of reducing equivalents (2H). Subsequent oxidation of 2H in the respiratory chain leads to phosphorylation of ADP to ATP. For one turn of the cycle, nine ATP are generated via oxidative phosphorylation and one ATP (or GTP) arises at substrate level from the conversion of succinyl-CoA to succinate.
REACTIONS OF THE CITRIC ACID CYCLE LIBERATE REDUCING EQUIVALENTS & CO2
The initial reaction between acetyl-CoA and oxaloacetate to form citrate is catalyzed by citrate synthase, which forms a carboncarbon bond between the methyl carbon of acetyl-CoA and the carbonyl carbon of oxaloacetate (Figure 17–3). The thioester bond of the resultant citryl-CoA is hydrolyzed, releasing citrate and CoASH—an exothermic reaction.
FIGURE 17–3 The citric acid (Krebs) cycle. Oxidation of NADH and FADH2 in the respiratory chain leads to the formation of ATP via oxidative phosphorylation. In order to follow the passage of acetyl-CoA through the cycle, the two carbon atoms of the acetyl radical are shown labeled on the carboxyl carbon (*) and on the methyl carbon (•). Although two carbon atoms are lost as CO2 in one turn of the cycle, these atoms are not derived from the acetyl-CoA that has immediately entered the cycle, but from that portion of the citrate molecule that was derived from oxaloacetate. However, on completion of a single turn of the cycle, the oxaloacetate that is regenerated is now labeled, which leads to labeled CO2 being evolved during the second turn of the cycle. Because succinate is a symmetric compound, “randomization” of label occurs at this step so that all four carbon atoms of oxaloacetate appear to be labeled after one turn of the cycle. During gluconeogenesis, some of the label in oxaloacetate is incorporated into glucose and glycogen (Figure 20–1). The sites of inhibition () by fluoroacetate, malonate, and arsenite are indicated.
Citrate is isomerized to isocitrate by the enzyme aconitase (aconitate hydratase); the reaction occurs in two steps: dehydration to cis-aconitate and rehydration to isocitrate. Although citrate is a symmetric molecule, aconitase reacts with citrate asymmetrically, so that the two carbon atoms that are lost in subsequent reactions of the cycle are not those that were added from acetyl-CoA. This asymmetric behavior is the result of channeling—transfer of the product of citrate synthase directly onto the active site of aconitase, without entering free solution. This provides integration of citric acid cycle activity and the provision of citrate in the cytosol as a source of acetyl-CoA for fatty acid synthesis. Citrate is only available in free solution to be transported from the mitochondria to the cytosol for fatty acid synthesis when aconitase is inhibited by accumulation of its product, isocitrate.
The poison fluoracetate
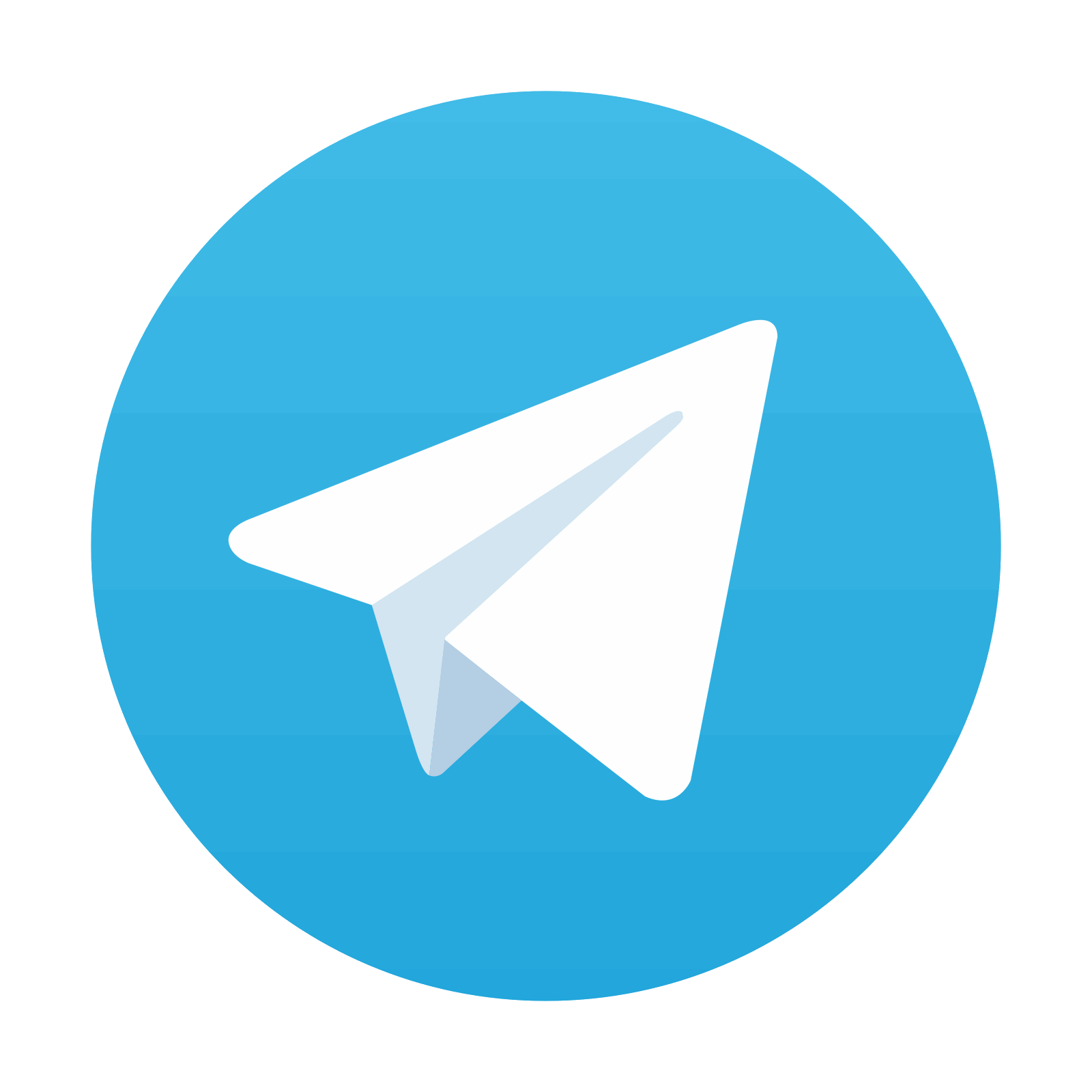
Stay updated, free articles. Join our Telegram channel

Full access? Get Clinical Tree
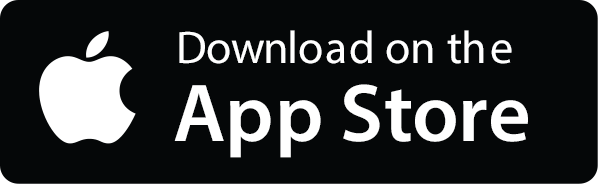
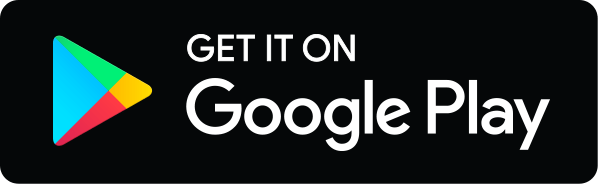