6 Michael Carroll Mankind has pondered the process of fertility and reproduction since the Palaeolithic period, as evidenced by the numerous Venus figurines discovered from that period, which are believed to represent female fecundity (Figure 6.1). However, the mechanism of procreation was attributed at that time to a more mystical modus. The Greek philosopher and ‘Father of Medicine’, Hippocrates (460–370 BC) approached reproduction rationally and proposed that both males and females produce seeds that combine to give rise to a new human – the ‘two seed theory’. Aristotle (384–322 BC) held the view that the unborn child was preformed in utero, consisting of female fluids and menstrual blood (catamenia), and awaited the male’s semen to elicit its development, bestowing on it form and animation. He considered that the preformed fetus grew like ‘the seeds of plants’. His theory of preformation or ‘epigenesis’ dominated the understanding of reproduction for over 1000 years until the latter part of the European Renaissance. Figure 6.1 The Venus of Willendorf is an 11.1 cm high figurine estimated to have been made between about 28,000 and 25,000 BCE. It was found in 1908 at a palaeolithic site near Willendorf, a village in Lower Austria near the town of Krems. Renewed interest in reproduction and fertilization ensued; for example, the Italian anatomist Hieronymus Fabricius (1537–1619) and his studies of domestic hens lead him to believe that the semen from the rooster was stored in a little sac near the cloaca where it rendered the whole uterus and eggs fertile. William Harvey (1578–1657), a student of Fabricius and the discoverer of blood circulation, was also interested in reproduction and sought a more active role for semen through dissection of female deer, dogs, rabbits, and chickens. However, he found no ‘evidence’ of a physical role for semen in reproduction, but ascribed its role as more ethereal, a conclusion more in accord with Aristotle’s preformationist idea. Harvey concluded that ‘all things come from the egg’ (ex ovo omnia). Developments in technology spawn discoveries in science, and the emergence of the microscope is a fine example. The arrival of printed books and manuscripts through mechanical improvements of Gutenberg’s printing press during the European Renaissance generated a market for ground lenses as an aid to reading. Around 1600, the Dutch spectacle makers, father and son, Hans and Zacharias Janssen, and Hans Lipperskey are accredited with inventing the first microscope, in which they fashioned two lenses at each end of an adjustable tube. Later the English polymath, Robert Hooke (1635–1703) improved on the design (Figure 6.2) and introduced curious readers to microscopic wonders in his magnificent Micrographica (published 1665). This masterpiece contained 38 plates, including a large pullout illustration of a flea, and included the first biological reference to cells. Figure 6.2 (a) Robert Hooke’s microscope and (b) Illustration of Robert Hooke. Source: https://commons.wikimedia.org. One likely reader of Micrographica was the Dutchman, Antonie van Leeuwenhoek (1632–1723). Leeuwenhoek had no formal university education; he worked as a draper and acted as a minor city official in the Dutch town of Delft. He was a meticulous observer and keen craftsman, designing and making hundreds of microscopes. His unique design was different to the compound double‐lens microscopes of Janssen and Hooke. Leeuwenhoek’s were mostly small handheld, single‐lens microscopes with impressive magnifying and resolving powers (Figure 6.3a) with which he made some remarkable discoveries, notably, the first observations and descriptions of small ‘animalcules’ – bacteria and protist – in samples of water. He corresponded with the Royal Society in London, whose members (including Hooke) were impressed with his microscopic skills and meticulous descriptions, and made him a foreign member in 1680. Figure 6.3 (a) Replica of Leeuwenhoek’ microscope. Source: photo by author. (b) Illustration of sperm as observed by Leeuwenhoek. (c) Leeuwenhoek. (d) The Homunculus as described by Hartsoeker. Source: https://commons.wikimedia.org. Leeuwenhoek’s most famous discovery is that of sperm. He believed that the generation of animals was from these ‘animalcules in the male sperm’ and noted their presence in abundance in his own semen and that of the dog, rabbit, and cockerel (Figure 6.3b). Leeuwenhoek (Figure 6.3c) ascribed to the preformationist view of generation, stating that he discovered ‘the parts and membranes of the fetus’, including the head and the shoulders. These animalcules travel to the uterus where they grow and develop – ‘the female served only to afford nourishment to the animalcules of the male sperm’, akin to a seed planted in nutrient soil (for his letters to the Royal Society see vanleeuwenhoek.com). The younger contemporary of Leeuwenhoek and fellow Dutchman, Nicolas Hartsoeker (1656–1725) predicted that these preformed little men would look like the drawing of the ‘homunculi’ in these animalcules (Figure 6.3d). Thus, in the seventeenth and eighteenth century, the concept of reproduction lay in two preformationist arenas: the ovists, who contended that generation is derived from the preformed residing in the ovum; and the spermists who proposed the idea of the homunculi and the planting of the male seed. It was not until the careful and diligent experimentalist, and Italian Catholic priest, Lazzaro Spallanzani (1729–1799) turned his attention to the question of reproduction that the true role of both ovum and sperm were unraveled. Spallanzani was a great scientist and thinker – his interests and expertise included physics, chemistry, geology, and biology. He carefully designed controlled experiments, describing the methodology in enough detail for others to repeat his work. In terms of his approach to scientific research, he was ahead of his time. He debunked the theory of spontaneous generation over 100 years before Louis Pasteur’s swan‐neck flask experiments. He first postulated how bats navigate in the darkness using their ears (echolocation) and observed the regenerative properties of salamander limbs. However, some of his most ingenious experiments related to fertilization and reproduction. In one experiment, he examined the nature of the sperm’s aura spermatica. This was one prevailing theory, which explained the generation of animals and was derived from the ideas of preformationists such as Harvey, proposing that a vapour emanating from semen triggered embryonic development. To investigate this, Spallanzani placed semen from a toad in a watch glass while eggs from the female were placed in the bottom of another watch glass turned upside down. The eggs were separated from the sperm by a few millimetres. After several hours, he noted the eggs were covered ‘as if by a dew’, from the condensation of the evaporated seminal fluid. However, none of the eggs developed. This refuted the property of the aura spermatica of sperm. In another, rather creative experiment, he prized male frogs from amplexus (the mating position where the males grasps the female with its front legs) and fitted them with tight taffeta britches before replacing them to resume their mating position. In this way, he was able to demonstrate unequivocally, the role of the egg and semen in the generation of animals. Thus, with the taffeta barrier, none of the eggs developed. But when he scraped the semen from the britches and added it to the eggs, they all developed into tadpoles. This was one of the earliest demonstrations of in vitro fertilization (IVF) and the proof that fertilization took place by the physical contact between semen and egg. In an attempt to distinguish which fraction of semen was responsible for fertilization, Spallanzani separated the sperm from the semen by filtration and exposed the eggs to the filtrate upon which they developed. If he had examined these filtrates microscopically, he would have observed swimming little animalcules. However, he did not, and erroneously concluded that semen had the fertilizing property, not the sperm. It would be another 100 years before direct interaction of sperm and eggs could be characterized. The developments and improvements in optics and microscopic techniques in the mid‐ to late nineteenth century, together with the elucidation of the cell theory by Virchow, Schwann, and Schleiden paved the pathway for a more accurate explanation and description of the process of fertilization. Two embryologists, Oscar Hertwig (1849–1922) and Hermann Fol (1845–1892) – both students of the great biologist Ernst Haeckel – independently described sperm entry into the egg and the subsequent union of male and female nuclei. To observe fertilization, Hertwig used the sea urchin, while Fol used the starfish – taking advantage of the numerous, large transparent eggs released by both species. Thus, it was nearly 200 years after Leeuwenhoek’s discovery that it was finally recognized that both egg and sperm were intimately involved and the fusion of both gametes was essential for fertilization to take place. There were many other individuals who played an essential role along this journey; the above mentioned are a few key players. For a more in‐depth narrative see: Pinto‐Correia (1997), Jardine (1999) and Cobb (2006). Fertilization can be described as a multistep process that results in the restoration of diploidy and the generation of a new organism through the combination of the maternal and paternal (haploid) genomes. To achieve this both gametes must obviously meet. For marine organisms (and other external fertilizers) this can pose a problem, especially when the gametes are spawned in vast volumes of water and their environment is shared with many other species, some possibly close relatives, that may have shed their gametes at the same time, increasing the risk of cross‐fertilization. When the gametes of marine animals are released into the water, in such a dilute concentration, the sperm require some means of attraction to the oocyte of their species. This species‐specific sperm attraction is known as sperm chemotaxis and may be defined operationally as a ‘modulation of the direction of movement of spermatozoa up a gradient of a chemoattractant’ (or down the gradient of a chemorepellent) (Eisenbach 1999). Sperm chemotaxis has been well documented in species such as molluscs, echinoderms (sea urchins and starfish) and ascidians (sea‐squirts) (Miller 1985; Yoshida et al. 1993). The precise nature of these sperm attractants varies between species. Most have been identified as sperm‐activating peptides or SAPs (Miller 1985). In the sea urchin, the SAP isolated from the egg jelly layer of the sea urchin, Arbacia punctulata, is known as ‘resact’, and is a potent chemoattractant for A. punctulata spermatozoa (Ward et al. 1985). In addition, this peptide is specific for A. punctulata and stimulates sperm motility by activating the sperm receptor’s guanylyl cyclase thus generating cyclic guanosine monophosphate (cGMP) and subsequent influx of Ca2+ which controls swimming behaviour during chemotaxis (Cook et al. 1994). The journey of the sperm is more complicated with internal fertilizers such as mammals where the sperm is placed directly in the female reproductive tract and has to make its way to the region where the oocyte awaits (Figure 6.4). Figure 6.4 The journey of sperm in the human female reproductive tract. Source: Blausen Medical, https://commons.wikimedia.org. In humans, the site of sperm deposition is the cranial vagina, at the external os of the cervix (Harper 1994). Soon after ejaculation, human seminal plasma spontaneously coagulates, due to the activity of semenogelin (I–III) proteins expressed exclusively in the seminal vesicles (de Lamirande 2007), which it has been proposed retains the sperm at the cervical external os (Harper 1994), preventing premature capacitation and providing protection from the acidic vaginal environment and from oxidative damage (de Lamirande and Lamothe 2010). This coagulum begins to break down within 30–60 min postejaculation by the proteolytic activity of prostate‐specific antigen (Mattsson et al. 2014). However, within a few minutes postejaculation, sperm begin to leave the seminal plasma and pass through the cervical canal (Sobrero and McLeod 1962). The cervical mucus provides the first real barrier to sperm movement, and the degree of cervical mucus hydration directly correlates with sperm migration. A mucus sample with the highest percentage of water and lowest protein and glycoprotein concentration has the most positive effect on sperm migration (Morales et al. 1993). Moreover, the percentage hydration can change throughout the ovulatory cycle, (Katz et al. 1997) with maximum mucus hydration levels correlating with increased pregnancy rates (Bigelow et al. 2004). The cervix is an immunologically competent organ and in humans, coitus and insemination into the vagina stimulate leukocyte (neutrophils and macrophages) migration into the cervix, as well as into the vagina (Pandya and Cohen 1985; Thompson et al. 1992). Neutrophils also migrate readily through midcycle human cervical mucus (Parkhurst and Saltzman 1994). The role of these leukocytes as agents against sperm is uncertain, as their presence does not seem to interfere with fecundity. However, they may be present in response to potential microbial pathogens that can accompany sperm. Another hypothesis proposes that postcapacitated sperm are targeted by leukocytes and phagocytosed, thus removing spent, superfluous sperm (Eisenbach 2003; Oren‐Benaroya et al. 2007). Periovulatory cervical mucus contains IgA, and the vagina and uterus contain elevated levels of IgG. Under normal circumstances, certain immunoglobulins target sperm, and in some women these antisperm antibodies can impair fertility (Jones, 1994; Franklin et al. 1999; Kalaydijev et al. 2002). Furthermore, about one‐third of infertile women with a poor post‐coital test appear to have anti‐sperm antibodies in their cervical mucus (Clarke et al. 1984). However, it seems that sperm have developed methods to evade the immunosurveillance of the female reproductive tract. During maturation in the epididymis, the sperm surface undergoes dramatic changes, acquiring properties that are vital for its survival and performance in the female tract. One such change is the addition to the sperm surface of an atypical β‐defensin polypeptide, one of which, the β‐defensin DEFB126 becomes adsorbed to the entire sperm surface as it moves through the epididymal duct. Furthermore, it has been proposed that negatively charged glycans on DEFB126 facilitate efficient sperm movement through cervical mucus and cloak sperm from immune surveillance molecules (Yudin et al. 2005; Tollner et al. 2012). Some studies suggest that the cervix also functions as a reservoir, storing sperm in the cervical crypts, since motile sperm have been recovered from the cervix after 5 days of insemination (Gould et al. 1984). These sperm may be protected from the hostile immune environment of the cervix (Tollner et al. 2012), thus providing a source of sperm for a few days after coitus. However, it is unclear if these cervical sperm reach the Fallopian tube. In 1951, Rubenstein and co‐workers removed several motile sperm from the Fallopian tubes of a woman following hysterectomy, 30 min after insemination, and in another study sperm were recovered from the Fallopian tube of women who had a bilateral salpingectomy 5 min after insemination (Settlage et al. 1973). In other words, sperm can traverse the uterus rapidly. However, due to the lack of standardization of the experimental protocols and patient selection more research was warranted. Williams et al. (1993) conducted a more careful study where they inseminated women (attending the clinic for planned hysterectomies) with either their partner’s, or donor semen. Approximately 18 h later, both Fallopian tubes were ligatured into ampullary, isthmic, and intramural regions and each region flushed to assess the presence of sperm. Surprisingly, a median of only 251 sperm were recovered with the ovulatory ampulla containing more sperm than the nonovulatory ampulla. Theoretical calculations on the rate of sperm locomotion and the dimensions of the uterus and tube make it unlikely that the sperm travel through the uterus by intrinsic movement alone. Sperm transport is therefore most probably due to the action of uterine cilia and contractions of the myometrium. It has been reported that uterine smooth muscle contractions increase in intensity during the late follicular phase coinciding with the presence of sperm if sexual intercourse has taken place (Kunz et al. 1996). Furthermore, the role of oxytocin, which is released during intercourse, may further facilitate sperm transport by inducing uterine contractions (Kunz et al. 2007). The next stage of the sperm’s journey is centred on the uterotubal junction, which is filled with mucus and provides a further barrier to sperm such that only those with good motility and morphology are permitted to traverse it. Additionally, the presence of certain sperm surface proteins may play an essential role in the passage (Suarez and Pacey 2006; Suarez 2016). The Fallopian tube provides a more hospitable milieu than the vagina or cervix, and it has been postulated that the tubal isthmus may function as a sperm reservoir. This is likely for a variety of species (Suarez and Pacey 2006). However, in one study a distinct sperm reservoir was not observed in the human tubes (Williams et al. 1993). Pregnancy can occur as long as 5 days before ovulation (Wilcox et al. 1995) and therefore sperm must be stored at some location(s) along the female reproductive tract. Given that human sperm do attach to endosalpingeal epithelium in vitro (Pacey et al. 1995; Vigil et al. 2012) (Figure 6.5), it likely that the human Fallopian tube functions as a sperm reservoir as noted in other mammal species. Figure 6.5 Scanning electron micrograph of an explant of human oviduct taken 3 h after insemination with human spermatozoa. Note the spermatozoon (arrow) of normal morphology attached by its acrosomal region to several cilia. Ciliated epithelial cells predominate, but there are also some nonciliated epithelial cells (arrow head) covered by microvilli. Observe the mucous material (asterisk) near the spermatozoon tail. The scale bar represents 10 µm. Reproduced from Vigil et al. (2012) by permission of Oxford University Press. As has been described, of the 200–300 million sperm ejaculated at the cervical external os, only about 200 reach the oocyte. In addition, there are more sperm in the ovulatory ampulla than in the nonovulatory ampulla (Williams et al. 1993). In order for the sperm to reach the site of ovulation there is likely a guidance mechanism involved. The requirement for a chemoattractant for sperm in mammals was believed to be unnecessary since the sperm were ejaculated directly into the female reproductive tract and a sufficient number of sperm would reach the oocyte. The identity of the mammalian sperm chemoattractant remains unknown. However, the oocyte and cells of the surrounding cumulus oophorus are thought to secrete sperm chemoattractants independently (Sun et al. 2005). Thus, several studies have shown sperm chemotaxis to follicular fluid, and fractions of follicular fluid contain heparin, progesterone, atrial natriuretic peptide, oxytocin, and acetylcholine – all of which demonstrate sperm chemoattractant properties (Eisenbach 1999). There is growing evidence supporting a role for progesterone as the mammalian sperm chemoattractant (Teves et al. 2006). This hormone is secreted by the cumulus cells (Guidobaldi et al. 2008) and induces sperm hyperactivation by binding to the Ca2+ channel CatSper on the sperm membrane (Lishko et al. 2011; Strünker et al. 2011). However, some data suggest that progesterone might be a weak, but not the major chemoattractant derived from follicular fluid. Progesterone may well cause human sperm accumulation mainly by inducing hyperactivation‐like motility and, as a consequence, sperm trapping (Jasiwal et al. 1999). An unexpected group of receptor proteins – members of the odorant receptor (OR) family normally expressed on ciliary membranes of nasal olfactory sensory neurons – have been detected on sperm (Parmentier et al. 1992) where they may play a role in sperm chemotaxis (Spher et al. 2003). OR hOR17 mediates changes in both sperm intracellular Ca2+ and swimming behaviour, via a cyclic adenosine monophosphate (cAMP)‐regulated pathway (Spehr et al. 2006). The list of potential OR functions in mammals has now been significantly extended to include sperm–oocyte chemical communication (Flegel et al. 2016). Furthermore, studies are now investigating an important role of sperm ORs and idiopathic infertility in men (Ottaviano et al. 2013). Mammalian fertilization does not occur when mature sperm from a fresh ejaculate are placed with oocytes in vitro. This observation bewildered researchers until 1951 when Chang and Austin independently reported that mammalian sperm must reside in the female reproductive tract for a predetermined period of time before they gain the ability to fertilize the oocyte. Austin introduced the term ‘capacitation’ stating ‘the sperm must undergo some form of physiologic change or capacitation before it is capable of penetrating the egg’ (Chang 1951; Austin 1952). Capacitation comprises a reversible set of physiological changes that involve the removal of cholesterol by albumin in the female reproductive tract and a prerequisite for hyperactivation (see below), and the acrosome reaction. In vivo, it involves the removal of inhibitory factors from seminal plasma and the interaction of the sperm with components in the female reproductive tract (Jaiswal and Eisenbach, 2002). In humans, capacitation most likely begins in the cervix (Overstreet et al. 1991). However, it has been postulated that not all the sperm will capacitate simultaneously and that the process is more a transient event, occurring throughout the female reproductive tract (Eisenbach 1995). The removal of cholesterol permits the influx of bicarbonate and Ca2+ ions, which in turn activate adenylate cyclase, thereby elevating cAMP concentrations. The increased cAMP levels activate protein kinase A, which then phosphorylates several tyrosine kinases, including chaperone proteins. These migrate to the sperm head where they are phosphorylated. One of these chaperone proteins is Izumo – a protein critical for sperm–egg fusion (see below) (Aitken and Nixon 2013). During capacitation sperm become hyperactivated – that is, they swim with higher velocity and generate greater force and tail movements. Hyperactivation is brought about by the influx of Ca2+ through the opening of the membrane Ca2+ channel, CatSper, located on the sperm tail (Quill et al. 2003). Furthermore, progesterone has been identified as a regulator of CatSper (Lishko et al. 2011; Strunker et al. 2011). As progesterone is produced from the cumulus cells and has been proposed to be the sperm chemoattractant, the interaction between this steroid hormone and CatSper becomes more evident. Hyperactivated sperm are released from the oviductal epithelium and migrate up the Fallopian tube towards the oocyte where they engage the cumulus mass. This directed journey is attributed not only to the activity of chemoattractants and CatSper, but the local environment. For example, alterations in pH can influence the activity of CatSper and it has been proposed there is a temperature gradient of 2 °C between the isthmus of the Fallopian tube and the warmer ampullary region (Bahat and Eisenbach 2006).
The Biology of Fertilization
A Brief History
The Journey of the Sperm
Finding the Oocyte
Capacitation, Hyperactivation, and the Acrosome Reaction
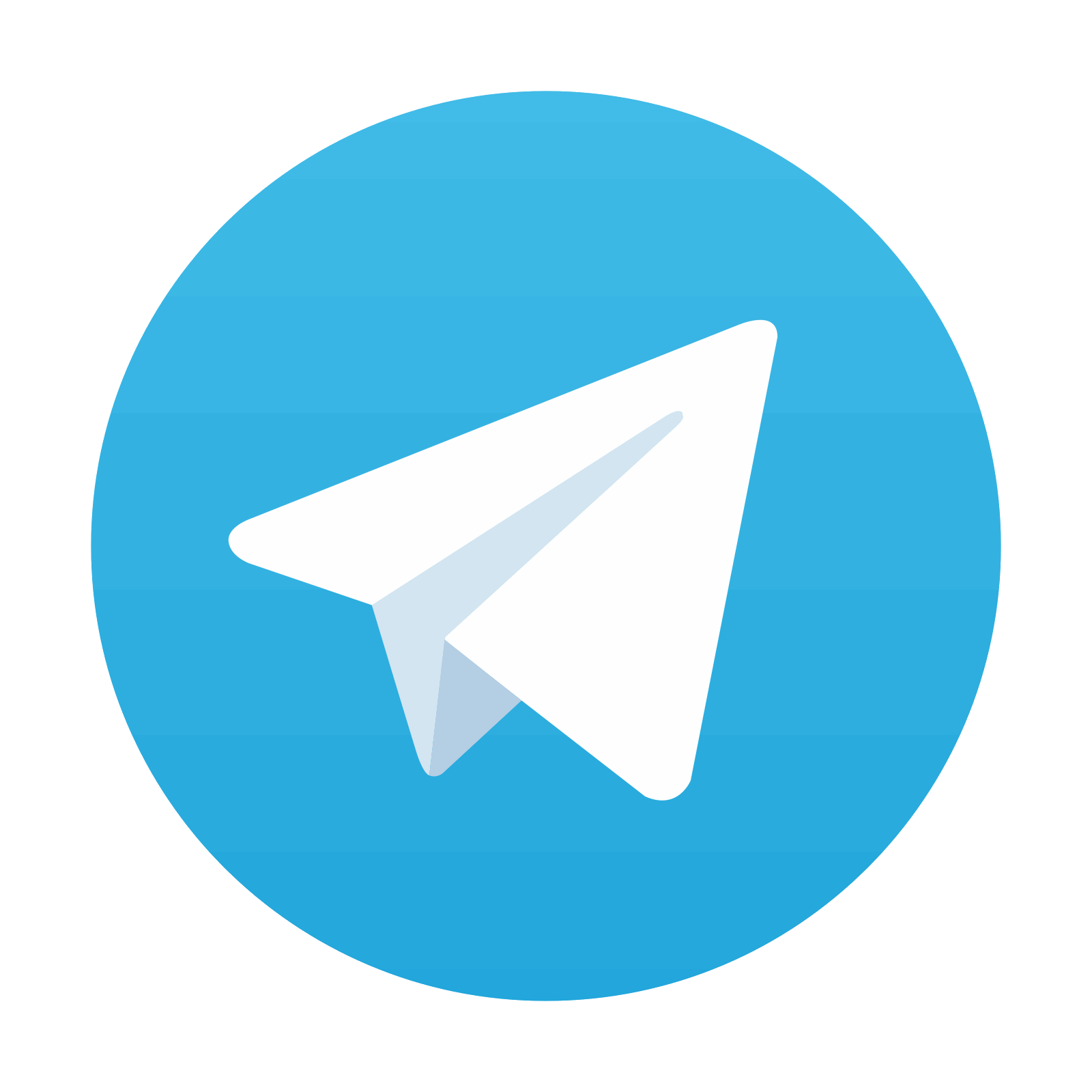
Stay updated, free articles. Join our Telegram channel

Full access? Get Clinical Tree
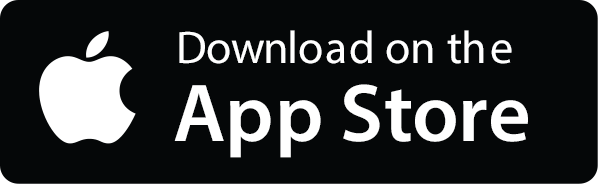
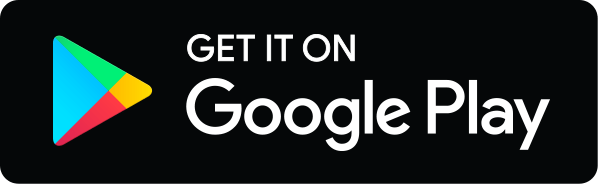