30 Colleen Lynch and Brendan Ball Preimplantation testing of embryos is seen by many, especially the general public, as a new and experimental technology. However, the first babies were born following this treatment little over 10 years following the birth of the first in vitro fertilization (IVF) baby (Handyside et al. 1990). In fact, Robert Edwards, a pioneer of both IVF and preimplantation testing, performed sexing of rabbit blastocysts (Edwards and Gardner 1967) 10 years before the world’s first IVF birth, that would eventually lead to his Nobel prize. Preimplantation testing of embryos requires IVF technologies, given that couples have to undergo IVF treatment to produce embryos for testing. Recent advances in both IVF technologies, molecular genetics, and cytogenetics have opened the door to offering such treatments to a much wider range of patients. There are two main categories of preimplantation testing: (i) preimplantation genetic diagnosis (PGD) involving testing for monogenic disease, tissue type, gender, and chromosome rearrangements, and (ii) preimplantation genetic screening (PGS) for the identification of chromosomally normal, or euploid, embryos in IVF treatment cycles (this is also known as PGD‐AS, where AS stands for aneuploidy screening). In recent years, PGD has garnered wider acceptance as a feasible reproductive option for couples at risk of passing a heritable condition to their children. The selection and use of unaffected embryos is, for most, an acceptable alternative to prenatal diagnosis and selective termination. For some, however, there is still the issue of what happens to affected embryos and the message it sends to affected individuals. PGS remains more controversial, even within the scientific and medical communities, given different practices and technologies have shown conflicting results, for example decreasing pregnancy rates, providing no benefit, and increasing pregnancy rates (Mastenbroek et al. 2011). The theory behind the intervention – the high percentage of aneuploidy in human embryos – remains sound, however, and recent studies involving blastocyst biopsy and comprehensive chromosome screening are proving promising. PGD was developed in response to couples at risk of passing heritable conditions to their children. The diagnosis of affected embryos prior to implantation and conception circumvents the need for prenatal testing and selective termination. PGD is used in the following situations: The first PGD cases were performed in 1989 for X‐linked monogenic disorders (Handyside et al. 1990). At this time, the specific genes and mutations involved in many conditions were unknown. Thus, in the situation of X‐linked monogenic disorders, couples were offered prenatal diagnosis via chorionic villus sampling or amniocentesis with cytogenetic analysis to determine the gender of the fetus. Although only 50% of male fetuses would be affected, in some cases the inability to perform specific disease testing meant that to avoid the condition, selective termination of male pregnancies was the only option. PGD was developed as an alternative for couples who had already undergone previous terminations or had trouble conceiving naturally. PGD was performed as a clinical treatment for the first time at Hammersmith Hospital in the UK. Couples at risk of having children with the X‐linked conditions Lesch‐Nyhan syndrome, adrenoleukodystrophy, Duchenne muscular dystrophy, and X‐linked mental retardation underwent IVF cycles with embryo biopsy at the Day 3 stage. Analysis was performed by amplification of a Y chromosome specific repeat sequence detected via gel electrophoresis – in the absence of amplification the embryo was inferred to be female. This was made possible by the development of the polymerase chain reaction (PCR), allowing exponential amplification of specific DNA targets. The short amount of time required for the analysis protocol meant that embryos could be transferred on the same day as biopsy, as blastocyst culture would not be robust or routine for a number of years. Later, the technique was improved by amplification of both X‐ and Y‐linked sequences and then the use of fluorescent in situ hybridization (FISH) allowing for the visualization of both sex chromosomes, XX or XY, and reducing the possibility of misdiagnosis. The introduction of FISH to single‐cell work opened up the possibility of PGD to couples where one carried a chromosome rearrangement – a reciprocal or robertsonian translocation or an inversion. This was first applied in the mid‐1990s, using FISH probes specific to the chromosomal breakpoints and enabling the detection of different unbalanced segregation patterns (Munne et al. 1998), although it had already been applied to general aneuploidy screening. The use of PGD for chromosome rearrangements remained more controversial as some groups showed that couples would be successful in the same time frame naturally when compared with multiple PGD cycles (Scriven et al. 2013). However, this failed to consider the emotional impact of recurrent miscarriage for couples. The advent of array‐based comparative genomic hybridization (aCGH), then next generation sequencing (NGS), allowed off‐the‐shelf PGD testing for patients with chromosome rearrangements which would identify not only unbalanced segregants, but also any other aneuploidy present in the sample (Fiorentino et al. 2011; Zhang et al. 2016) (Figure 30.1). This has contributed to higher pregnancy rates and reduced miscarriage rates, and a wider application of the treatment. Figure 30.1 Preimplantation genetic diagnosis for a reciprocal translocation between the long arm chromosome 9 and the short arm of chromosome 11. The top and bottom images show analysis of the same trophectoderm sample via array‐based comparative genomic hybridization and next generation sequencing respectively, revealing an unbalanced female karyotype. Reproduced with permission of Cooper Genomics. In 1992, the molecular techniques that first allowed the gender determination of embryos were extended to look at specific disease‐causing genetic mutations. Whilst the majority of groups continued to focus on blastomere biopsy, some undertook a combination of polar body and blastomere analysis – a position that was as much to do with legal implications as science. The first monogenic condition for which PGD was undertaken to detect a specific disease‐causing mutation in human embryos was cystic fibrosis (Handyside et al. 1992) – previously, much research had taken place on mouse embryos. The deltaF508 mutation is the highest frequency cystic fibrosis transmembrane conductance regulator (CFTR) mutation and is a 3 base pair deletion. Thus a nested PCR of the region and gel electrophoresis allowed the detection of DNA homo‐ and heteroduplexes and the identification of affected, unaffected, and carrier embryos. However, this technique was vulnerable to failed amplification of a specific allele, as the first gender selection cases had been. The introduction of multiplexing protocols allowed multiple linked markers to be used to follow disease inheritance (Dreesen et al. 2000) and then robust and accurate methods of whole genome amplification negated the need for multiplexing and greatly increased the number of linked markers that could be run and improved the reliability of testing (Spits et al. 2006). This also allowed more than one genetic condition to be tested for, or more commonly, to test for both disease and HLA type in embryos. For many years, these technologies were employed with little change to the diagnosis of monogenic disorders. However, the advent of single nucleotide polymorphism (SNP) arrays promises to be the biggest paradigm shift in the field since its introduction (Natesan et al. 2014) and potentially allows the tandem identification of meotic aneuploidy. Karyomapping involves using DNA samples from the couple requesting treatment and a reference (e.g. an affected child) to identify the parental origin at all SNP loci. This can be related to embryo samples to see if they have inherited the parental SNPs associated with the genetic condition (Figure 30.2). The genome wide coverage of this technique means that it can be used for the majority of single gene disorders and removes the need for the development of patient‐specific tests. Figure 30.2 Software analysis of karyomapping data (Illumina). Preimplantation genetic diagnosis for a genetic mutation at 16p13.3. In the case of a recessive genetic condition where the reference was an affected child, embryo 8 would be affected, embryos 1 and 4 would be paternal carriers, and embryo 10 would be a maternal carrier. In the case of a paternally inherited dominant condition, where the reference was an affected child, embryos 1, 4, and 8 would be affected and embryo 10 would be unaffected. The detailed haploblock chart shows the single nucleotide polymorphism calling along chromosome 16 for embryo 4. Reproduced with permission of Cooper Genomics. Regulation of PGD and PGS differs from country to country– whilst there may be no regulation in one country, it may be outlawed entirely in another. In addition to offering treatment in line with local regulatory requirements and accrediting or licensing bodies, IVF clinics should have their own guidelines for staff, especially if offering PGD (Harton et al. 2011a). These may include stipulating which conditions PGD can be offered for and consideration of: For each case, the clinic may also wish to consider the reliability of the test available, likelihood of success, and safety (including any medical contraindications where an individual is affected by the condition for which testing is being performed). The clinic should also be able to provide or access appropriate counselling for couples seeking treatment including: The introduction of FISH to single cell PGD was only subsequent to chromosome screening and PGS (Munne et al. 1993). The age‐related decline in natural and IVF birth rates is linked to increased aneuploidy rates (Figure 30.3), which contribute to failed implantation, miscarriage, and increased rates of Down, Edwards, and Patau syndromes. Theoretically, the identification and negative selection of chromosomally abnormal embryos should mitigate the age effect in IVF and increase implantation and reduce miscarriage rates in patients of advanced maternal age. Thus, PGS was generally indicated in patients with advanced maternal age, recurrent miscarriage, and recurrent implantation failure and was reported to improve success rates (Munne 2002). However, there were a number of issues with this strategy. Firstly, FISH could only test a limited number of chromosomes– generally between five and eight. The chromosomes tested were those associated with miscarriage and live birth, meaning many abnormalities that would result in failed implantation were undetected. Secondly, there were a number of technical artefacts affecting the reliability of the testing: loss of micronuclei during cell fixing, split signals, and overlapping signals. Thirdly, the incidence and impact of mosaicism was not fully understood. Thus, when randomized clinical trials (RCTs) were published it was demonstrated that PGS in fact gave no advantage, or even reduced success rates (Mastenbroek et al. 2011). This was controversial at the time and hotly contended by the initial pioneers of the treatment who continued to evidence improved success rates (Munne et al. 2007). However, it did lead to many changes in practice, most notably the introduction of comprehensive chromosome screening (CCS). Despite more recent RCTs and systematic reviews supporting the use of PGS when CCS is employed, the majority of the scientific community seems to remain skeptical (Gleicher and Orvieto 2017). Figure 30.3 Data collected by Cooper Genomics showing the percentage of aneuploid embryos by maternal age. Reproduced with permission of Cooper Genomics. Human reproduction is characteristic among mammalian species for the high rate of chromosomally abnormal gametes and embryos produced. Ten to fifteen percent of clinically recognized pregnancies end in first trimester miscarriage, which does not include occult or missed abortions. Transient implantation may occur with minimal disruption to the menstrual cycle and an individual might never realize that it has occurred. More than half of these events, 60–80%, are a result of aneuploidy in the embryo. Additionally, an even higher rate of aneuploidy exists at the embryo stage than detected in pregnancy given that many chromosomally abnormal embryos will simply fail to implant. Thus, the rationale behind PGS remains sound and the advent of CCS has led to evidence for it to be best employed as a method of embryo selection in patients with a good prognosis. Unlike PGD, PGS has seen vast and regular changes in the technologies employed – from the advent of PGS via FISH looking at a single data point on a limited number of chromosomes, to quantitative PCR (qPCR) looking at two to four data points per chromosome, array‐based comparative hybridization looking at hundreds of points per chromosome, and NGS looking at tens of thousands of data points per chromosome (Handyside 2013).
Preimplantation Genetic Diagnosis and Screening
Introduction
Preimplantation Genetic Diagnosis
PGD Technologies
Regulation and Guidelines
Preimplantation Genetic Screening
PGS Technologies
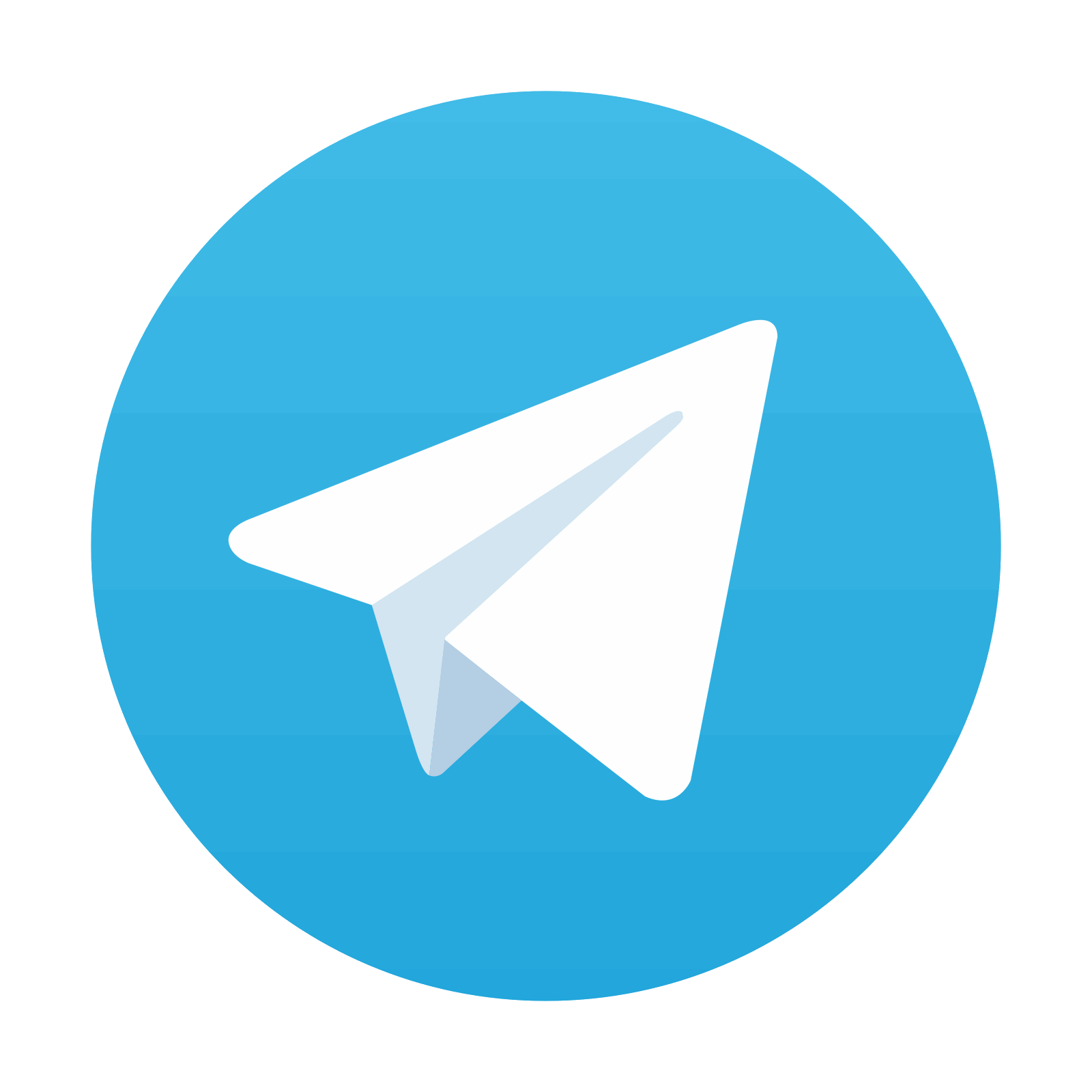
Stay updated, free articles. Join our Telegram channel

Full access? Get Clinical Tree
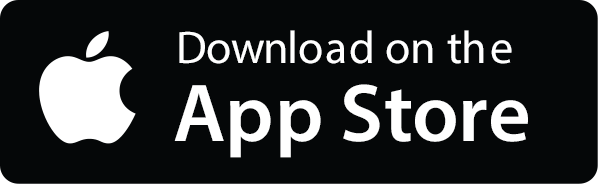
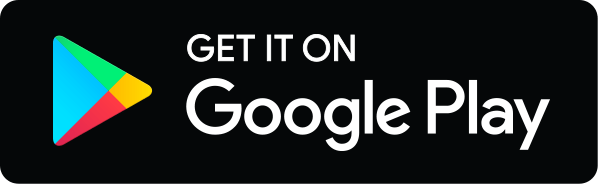