OBJECTIVES
After reading this chapter, you should be able to:
Name the three catecholamines secreted by the adrenal medulla and summarize their biosynthesis, metabolism, and function.
List the stimuli that increase adrenal medullary secretion.
Differentiate between C18, C19, and C21 steroids and give examples of each.
Outline the steps involved in steroid biosynthesis in the adrenal cortex.
Name the plasma proteins that bind adrenocortical steroids and discuss their physiologic role.
Name the major site of adrenocortical hormone metabolism and the principal metabolites produced from glucocorticoids, adrenal androgens, and aldosterone.
Describe the mechanisms by which glucocorticoids and aldosterone produce changes in cellular function.
List and briefly describe the physiologic and pharmacologic effects of glucocorticoids.
Contrast the physiologic and pathologic effects of adrenal androgens.
Describe the mechanisms that regulate secretion of glucocorticoids and adrenal sex hormones.
List the actions of aldosterone and describe the mechanisms that regulate aldosterone secretion.
Describe the main features of the diseases caused by excess or deficiency of each of the hormones of the adrenal gland.
INTRODUCTION
There are two endocrine organs in the adrenal gland, one surrounding the other. The main secretions of the inner adrenal medulla (Figure 20–1) are the catecholamines epinephrine, norepinephrine, and dopamine; the outer adrenal cortex secretes steroid hormones.
FIGURE 20–1
Human adrenal glands. Adrenocortical tissue is yellow; adrenal medullary tissue is orange. Note the location of the adrenals at the superior pole of each kidney. Also shown are extra-adrenal sites (gray) at which cortical and medullary tissue is sometimes found. (Reproduced with permission from Williams RH: Textbook of Endocrinology, 4th ed. St. Louis, MO: Saunders; 1968.)
The adrenal medulla is in effect a sympathetic ganglion in which the postganglionic neurons have lost their axons and become secretory cells. The cells secrete when stimulated by the preganglionic nerve fibers that reach the gland via the splanchnic nerves. Adrenal medullary hormones work mostly to prepare the body for emergencies, the so-called “fight-or-flight” responses.
The adrenal cortex secretes glucocorticoids, steroids with widespread effects on the metabolism of carbohydrate and protein; and a mineralocorticoid essential to the maintenance of Na+ balance and extracellular fluid (ECF) volume. It is also a secondary site of androgen synthesis, secreting sex hormones such as testosterone, which can exert effects on reproductive function. Mineralocorticoids and the glucocorticoids are necessary for survival. Adrenocortical secretion is controlled primarily by adrenocorticotropic hormone (ACTH) from the anterior pituitary, but mineralocorticoid secretion is also subject to independent control by circulating factors, of which the most important is angiotensin II, a peptide formed in the bloodstream by the action of renin.
ADRENAL MORPHOLOGY
The adrenal medulla, which constitutes 28% of the mass of the adrenal gland, is made up of interlacing cords of densely innervated granule-containing cells that abut on venous sinuses. Two cell types can be distinguished morphologically: an epinephrine-secreting type that has larger, less dense granules; and a norepinephrine-secreting type in which smaller, very dense granules fail to fill the vesicles in which they are contained. In humans, 90% of the cells are the epinephrine-secreting type and 10% are the norepinephrine-secreting type. The type of cell that secretes dopamine is unknown. Paraganglia, small groups of cells resembling those in the adrenal medulla, are found near the thoracic and abdominal sympathetic ganglia (Figure 20–1).
In adult mammals, the adrenal cortex is divided into three zones (Figure 20–2). The outer zona glomerulosa is made up of whorls of cells that are continuous with the columns of cells that form the zona fasciculata. These columns are separated by venous sinuses. The inner portion of the zona fasciculata merges into the zona reticularis, where the cell columns become interlaced in a network. The zona glomerulosa makes up 15% of the mass of the adrenal gland; the zona fasciculata, 50%; and the zona reticularis, 7%. The adrenocortical cells contain abundant lipid, especially in the outer portion of the zona fasciculata. All three cortical zones secrete corticosterone, but the active enzymatic mechanism for aldosterone biosynthesis is limited to the zona glomerulosa, whereas the enzymatic mechanisms for forming cortisol and sex hormones are found in the two inner zones. Furthermore, subspecialization occurs within the inner two zones, with the zona fasciculata secreting mostly glucocorticoids and the zona reticularis secreting mainly sex hormones.
FIGURE 20–2
Section through an adrenal gland showing both the medulla and the zones of the cortex, as well as the hormones they secrete. (Reproduced with permission from Widmaier EP, Raff H, Strang KT: Vander’s Human Physiology: The Mechanisms of Body Function, 11th ed. New York, NY: McGraw-Hill; 2008.)
Arterial blood reaches the adrenal from many small branches of the phrenic and renal arteries and the aorta. From a plexus in the capsule, blood flows through the cortex to the sinusoids of the medulla. The medulla is also supplied by a few arterioles that pass directly to it from the capsule. In most species, including humans, blood from the medulla flows into a central adrenal vein. The blood flow through the adrenal is large, as it is in most endocrine glands.
During fetal life, the human adrenal is large and under pituitary control, but the three zones of the permanent cortex represent only 20% of the gland. The remaining 80% is the large fetal adrenal cortex, which undergoes rapid degeneration at the time of birth. A major function of this fetal adrenal is synthesis and secretion of sulfate conjugates of androgens that are converted in the placenta to estrogens (see Chapter 22). No structure is comparable to the human fetal adrenal in laboratory animals.
An important function of the zona glomerulosa, in addition to aldosterone synthesis, is the formation of new cortical cells. The adrenal medulla does not regenerate, but when the inner two zones of the cortex are removed, a new zona fasciculata and zona reticularis regenerate from glomerular cells attached to the capsule. Small capsular remnants regrow large pieces of adrenocortical tissue. Immediately after hypophysectomy, the zona fasciculata and zona reticularis begin to atrophy, whereas the zona glomerulosa is unchanged because of the action of angiotensin II on this zone. The ability to secrete aldosterone and conserve Na+ is normal for some time after hypophysectomy, but in long-standing hypopituitarism, aldosterone deficiency may develop, apparently because of the absence of a pituitary factor that maintains the responsiveness of the zona glomerulosa. Injections of ACTH and stimuli that cause endogenous ACTH secretion produce hypertrophy of the zona fasciculata and zona reticularis but actually decrease, rather than increase, the size of the zona glomerulosa.
The cells of the adrenal cortex contain large amounts of smooth endoplasmic reticulum, which is involved in the steroid-forming process. Other steps in steroid biosynthesis occur in the mitochondria. The structure of steroid-secreting cells is very similar throughout the body. The typical features of such cells are shown in Figure 20–3.
FIGURE 20–3
Schematic overview of the structures of steroid-secreting cells and the intracellular pathway of steroid synthesis. LDL, low-density lipoprotein; PKA, protein kinase A. (Reproduced with permission from Widmaier EP, Raff H, Strang KT: Vander’s Human Physiology: The Mechanisms of Body Function, 11th ed. New York, NY: McGraw-Hill; 2008.)
ADRENAL MEDULLA: STRUCTURE & FUNCTION OF MEDULLARY HORMONES
Norepinephrine, epinephrine, and small amounts of dopamine are synthesized by the adrenal medulla. Cats and some other species secrete mainly norepinephrine, but in dogs and humans, most of the catecholamine output in the adrenal vein is epinephrine. Norepinephrine also enters the circulation from noradrenergic nerve endings.
The structures of norepinephrine, epinephrine, and dopamine and the pathways for their biosynthesis and metabolism are discussed in Chapter 7. Norepinephrine is formed by hydroxylation and decarboxylation of tyrosine, and epinephrine by methylation of norepinephrine. Phenylethanolamine-N-methyltransferase (PNMT), the enzyme that catalyzes the formation of epinephrine from norepinephrine, is found in appreciable quantities only in the brain and the adrenal medulla. Adrenal medullary PNMT is induced by glucocorticoids. Although relatively large amounts are required, the glucocorticoid concentration is high in the blood draining from the cortex to the medulla. After hypophysectomy, the glucocorticoid concentration of this blood falls and epinephrine synthesis is decreased. In addition, glucocorticoids are apparently necessary for the normal development of the adrenal medulla; in 21β-hydroxylase deficiency, glucocorticoid secretion is reduced during fetal life and the adrenal medulla is dysplastic. In untreated 21β-hydroxylase deficiency, circulating catecholamines are low after birth.
In plasma, about 95% of the dopamine and 70% of the norepinephrine and epinephrine are conjugated to sulfate. Sulfate conjugates are inactive and their function is unsettled. In recumbent humans, the normal plasma level of free norepinephrine is about 300 pg/mL (1.8 nmol/L). On standing, the level increases 50–100% (Figure 20–4). The plasma norepinephrine level is generally unchanged after adrenalectomy, but the free epinephrine level, which is normally about 30 pg/mL (0.16 nmol/L), falls to essentially zero. The epinephrine found in tissues other than the adrenal medulla and the brain is for the most part absorbed from the bloodstream rather than synthesized in situ. Interestingly, low levels of epinephrine reappear in the blood some time after bilateral adrenalectomy, and these levels are regulated like those secreted by the adrenal medulla. They may come from cells such as the intrinsic cardiac adrenergic (ICA) cells (see Chapter 13), but their exact source is unknown.
FIGURE 20–4
Norepinephrine and epinephrine levels in human venous blood in various physiologic and pathologic states. Note that the horizontal scales are different. The numbers to the left in parentheses are the numbers of subjects tested. In each case, the vertical dashed line identifies the threshold plasma concentration at which detectable physiologic changes are observed. (Modified and reproduced with permission from Cryer PE: Physiology and pathophysiology of the human sympathoadrenal neuroendocrine system. N Engl J Med 1980; Aug 21; 303(8):436–444.)
Plasma dopamine levels are normally very low, about 0.13 nmol/L. Most plasma dopamine is thought to be derived from sympathetic noradrenergic ganglia.
The catecholamines have a half-life of about 2 min in the circulation. For the most part, they are methoxylated and then oxidized to 3-methoxy-4-hydroxymandelic acid (vanillylmandelic acid [VMA]; see Chapter 7). About 50% of the secreted catecholamines appear in the urine as free or conjugated metanephrine and normetanephrine, and 35% as VMA. Only small amounts of free norepinephrine and epinephrine are excreted. In normal humans, about 30 μg of norepinephrine, 6 μg of epinephrine, and 700 μg of VMA are excreted per day.
In the medulla, norepinephrine and epinephrine are stored in granules with ATP. The granules also contain chromogranin A (see Chapter 7). Secretion is initiated by acetylcholine released from the preganglionic neurons that innervate the secretory cells. Acetylcholine activates cation channels allowing Ca2+ to enter the cells from the ECF and trigger the exocytosis of the granules. In this manner, catecholamines, ATP, and proteins from the granules are all released into the blood together.
Epinephrine-containing cells of the medulla also contain and secrete opioid peptides (see Chapter 7). The precursor molecule is preproenkephalin. Most of the circulating metenkephalin comes from the adrenal medulla. The circulating opioid peptides do not cross the blood–brain barrier.
Adrenomedullin, a vasodepressor polypeptide found in the adrenal medulla, is discussed in Chapter 32.
In addition to mimicking the effects of noradrenergic nervous discharge, norepinephrine and epinephrine exert metabolic effects that include glycogenolysis in liver and skeletal muscle, mobilization of free fatty acids (FFA), increased plasma lactate, and stimulation of the metabolic rate. The effects of norepinephrine and epinephrine are brought about by actions on two classes of receptors: α- and β-adrenergic receptors. α-Receptors are subdivided into two groups, α1 and α2 receptors, and β-receptors into three groups, β1, β2, and β3 receptors (see Chapter 7). There are three subtypes of α1-receptors and three subtypes of α2-receptors (see Table 7–2).
Norepinephrine and epinephrine both increase the force and rate of contraction of the isolated heart. These responses are mediated by β1-receptors. The catecholamines also increase myocardial excitability, causing extrasystoles and, occasionally, more serious cardiac arrhythmias. Norepinephrine produces vasoconstriction in most if not all organs via α1-receptors, but epinephrine dilates the blood vessels in skeletal muscle and the liver via β2-receptors. This usually overbalances the vasoconstriction produced by epinephrine elsewhere, and the total peripheral resistance drops. When norepinephrine is infused slowly in normal animals or humans, the systolic and diastolic blood pressures rise. The hypertension stimulates the carotid and aortic baroreceptors, producing reflex bradycardia that overrides the direct cardioacceleratory effect of norepinephrine. Consequently, cardiac output per minute falls. Epinephrine causes a widening of the pulse pressure but because baroreceptor stimulation is insufficient to obscure the direct effect of the hormone on the heart, cardiac rate and output increase. These changes are summarized in Figure 20–5.
Catecholamines increase alertness (see Chapter 14). Epinephrine and norepinephrine are equally potent in this regard, although in humans epinephrine usually evokes more anxiety and fear.
The catecholamines have several different actions that affect blood glucose. Epinephrine and norepinephrine both cause glycogenolysis. They produce this effect via β-adrenergic receptors that increase cyclic adenosine monophosphate (cAMP), with activation of phosphorylase, and via α-adrenergic receptors that increase intracellular Ca2+ (see Chapter 7). In addition, the catecholamines increase the secretion of insulin and glucagon via β-adrenergic mechanisms and inhibit the secretion of these hormones via α-adrenergic mechanisms.
Norepinephrine and epinephrine also produce a prompt rise in the metabolic rate that is independent of the liver and a smaller, delayed rise that is abolished by hepatectomy and coincides with the rise in blood lactate concentration. The initial rise in metabolic rate may be due to cutaneous vasoconstriction, which decreases heat loss and leads to a rise in body temperature, or to increased muscular activity, or both. The second rise is probably due to oxidation of lactate in the liver. Mice unable to make norepinephrine or epinephrine because their dopamine β-hydroxylase gene is knocked out are intolerant of cold, but surprisingly, their basal metabolic rate is elevated. The cause of this elevation is unknown.
When injected, epinephrine and norepinephrine cause an initial rise in plasma K+ because of release of K+ from the liver and then a prolonged fall in plasma K+ because of an increased entry of K+ into skeletal muscle that is mediated by β2-adrenergic receptors. Some evidence suggests that activation of α-receptors opposes this effect.
The increases in plasma norepinephrine and epinephrine that are needed to produce the various effects listed above have been determined by infusion of catecholamines in resting humans. In general, the threshold for the cardiovascular and the metabolic effects of norepinephrine is about 1500 pg/mL, that is, about five times the resting value (Figure 20–4). Epinephrine, on the other hand, produces tachycardia when the plasma level is about 50 pg/mL, that is, about twice the resting value. The threshold for increased systolic blood pressure and lipolysis is about 75 pg/mL; the threshold for hyperglycemia, increased plasma lactate, and decreased diastolic blood pressure is about 150 pg/mL; and the threshold for the α-mediated decrease in insulin secretion is about 400 pg/mL. Plasma epinephrine often exceeds these thresholds. On the other hand, plasma norepinephrine rarely exceeds the threshold for its cardiovascular and metabolic effects, and most of its effects are due to its local release from postganglionic sympathetic neurons. Most adrenal medullary tumors (pheochromocytomas) secrete norepinephrine or epinephrine or both and produce sustained hypertension. However, 15% of epinephrine-secreting tumors secrete this catecholamine episodically, producing intermittent bouts of palpitations, headache, glycosuria, and extreme systolic hypertension. These same symptoms are produced by intravenous injection of a large dose of epinephrine.
The physiologic function of the dopamine in the circulation is unknown. However, injected dopamine produces renal vasodilation, probably by acting on a specific dopaminergic receptor. It also produces vasodilation in the mesentery. Elsewhere, it produces vasoconstriction, probably by releasing norepinephrine, and it has a positive inotropic effect on the heart by an action on β1-adrenergic receptors. The net effect of moderate doses of dopamine is an increase in systolic pressure and no change in diastolic pressure. Because of these actions, dopamine is useful in the treatment of traumatic and cardiogenic shock (see Chapter 32).
Dopamine is made in the renal cortex. It causes natriuresis and may exert this effect by inhibiting renal Na, K, ATPase.
REGULATION OF ADRENAL MEDULLARY SECRETION
Certain drugs act directly on the adrenal medulla, but physiologic stimuli affect medullary secretion through the nervous system. Catecholamine secretion is low in basal states, but the secretion of epinephrine and, to a lesser extent, that of norepinephrine is reduced even further during sleep.
Increased adrenal medullary secretion is part of the diffuse sympathetic discharge provoked in emergency situations, which Cannon called the “emergency function of the sympathoadrenal system.” The ways in which this discharge prepares the individual for flight or fight are described in Chapter 13, and the increases in plasma catecholamines under various conditions are shown in Figure 20–4.
The metabolic effects of circulating catecholamines are probably important, especially in certain situations. The calorigenic action of catecholamines in animals exposed to cold is an example, and so is the glycogenolytic effect (see Chapter 24) in combating hypoglycemia.
When adrenal medullary secretion is increased, the ratio of norepinephrine to epinephrine in the adrenal effluent is generally unchanged. However, norepinephrine secretion tends to be selectively increased by emotional stresses with which the individual is familiar, whereas epinephrine secretion rises selectively in situations in which the individual does not know what to expect.
ADRENAL CORTEX: STRUCTURE & BIOSYNTHESIS OF ADRENOCORTICAL HORMONES
The hormones of the adrenal cortex are derivatives of cholesterol. Like cholesterol, bile acids, vitamin D, and ovarian and testicular steroids, they contain the cyclopentanoperhydrophenanthrene nucleus (Figure 20–6). Gonadal and adrenocortical steroids are of three types: C21 steroids, which have a two-carbon side chain at position 17; C19 steroids, which have a keto or hydroxyl group at position 17; and C18 steroids, which, in addition to a 17-keto or hydroxyl group, have no angular methyl group attached to position 10. The adrenal cortex secretes primarily C21 and C19 steroids. Most of the C19 steroids have a keto group at position 17 and are therefore called 17-ketosteroids. The C21 steroids that have a hydroxyl group at the 17 position in addition to the side chain are often called 17-hydroxycorticoids or 17-hydroxycorticosteroids.
FIGURE 20–6
Basic structure of adrenocortical and gonadal steroids. The letters in the formula for cholesterol identify the four basic rings, and the numbers identify the positions in the molecule. As shown here, the angular methyl groups (positions 18 and 19) are usually indicated simply by straight lines.
The C19 steroids have androgenic activity. The C21 steroids are classified, using Selye’s terminology, as mineralocorticoids or glucocorticoids. All secreted C21 steroids have both mineralocorticoid and glucocorticoid activity; mineralocorticoids are those in which effects on Na+ and K+ excretion predominate and glucocorticoids are those in which effects on glucose and protein metabolism predominate.
The details of steroid nomenclature and isomerism can be found elsewhere. However, it is pertinent to mention that the Greek letter Δ indicates a double bond and that the groups that lie above the plane of each of the steroid rings are indicated by the Greek letter β and a solid line (—OH), whereas those that lie below the plane are indicated by α and a dashed line (- – -OH). Thus, the C21 steroids secreted by the adrenal have a Δ4-3-keto configuration in the A ring. In most naturally occurring adrenal steroids, 17-hydroxy groups are in the α configuration, whereas 3-, 11-, and 21-hydroxy groups are in the β configuration. The 18-aldehyde configuration of naturally occurring aldosterone is the D form. L-aldosterone is physiologically inactive.
Innumerable steroids have been isolated from adrenal tissue, but the only steroids normally secreted in physiologically significant amounts are the mineralocorticoid aldosterone, the glucocorticoids cortisol and corticosterone, and the androgens dehydroepiandrosterone (DHEA) and androstenedione. The structures of these steroids are shown in Figure 20–7 and Figure 20–8. Deoxycorticosterone is a mineralocorticoid that is normally secreted in about the same amount as aldosterone (Table 20–1) but has only 3% of the mineralocorticoid activity of aldosterone. Its effect on mineral metabolism is usually negligible, but in diseases in which its secretion is increased, its effect can be appreciable. Most of the estrogens that are not formed in the ovaries are produced in the circulation from adrenal androstenedione. Almost all the dehydroepiandrosterone is secreted conjugated with sulfate, although most if not all of the other steroids are secreted in the free, unconjugated form (Clinical Box 20–1).
Name | Synonyms | Average Plasma Concentration (Free and Bound)a μg/dL) | Average Amount Secreted (mg/24 h) |
---|---|---|---|
Cortisol | Compound F, hydrocortisone | 13.9 | 10 |
Corticosterone | Compound B | 0.4 | 3 |
Aldosterone | 0.0006 | 0.15 | |
Deoxycorticosterone | DOC | 0.0006 | 0.20 |
Dehydroepiandrosterone sulfate | DHEAS | 175.0 | 20 |
FIGURE 20–7
Outline of hormone biosynthesis in the zona fasciculata and zona reticularis of the adrenal cortex. The major secretory products are underlined. The enzymes for the reactions are shown on the left and at the top of the chart. When a particular enzyme is deficient, hormone production is blocked at the points indicated by the shaded bars.
In all species from amphibia to humans, the major C21 steroid hormones secreted by adrenocortical tissue appear to be aldosterone, cortisol, and corticosterone, although the ratio of cortisol to corticosterone varies. Birds, mice, and rats secrete corticosterone almost exclusively; dogs secrete approximately equal amounts of the two glucocorticoids; and cats, sheep, monkeys, and humans secrete predominantly cortisol. In humans, the ratio of secreted cortisol to corticosterone is approximately 7:1.
The major paths by which the naturally occurring adrenocortical hormones are synthesized in the body are summarized in Figures 20–7 and 20–8. The precursor of all steroids is cholesterol. Some of the cholesterol is synthesized from acetate, but most of it is taken up from LDL in the circulation. LDL receptors are especially abundant in adrenocortical cells. The cholesterol is esterified and stored in lipid droplets. Cholesterol ester hydrolase catalyzes the formation of free cholesterol in the lipid droplets (Figure 20–9). The cholesterol is transported to mitochondria by a sterol carrier protein. In the mitochondria, it is converted to pregnenolone in a reaction catalyzed by an enzyme known as cholesterol desmolase or side-chain cleavage enzyme. This enzyme, like most of the enzymes involved in steroid biosynthesis, is a member of the cytochrome P450 superfamily and is also known as P450scc or CYP11A1. For convenience, the various names of the enzymes involved in adrenocortical steroid biosynthesis are summarized in Table 20–3.
FIGURE 20–9
Mechanism of action of ACTH on cortisol-secreting cells in the inner two zones of the adrenal cortex. When ACTH binds to its receptor (R), adenylyl cyclase (AC) is activated via Gs. The resulting increase in cAMP activates protein kinase A, and the kinase phosphorylates cholesteryl ester hydrolase (CEH), increasing its activity. Consequently, more free cholesterol is formed and converted to pregnenolone. Note that in the subsequent steps in steroid biosynthesis, products are shuttled between the mitochondria and the smooth endoplasmic reticulum (SER). Corticosterone is also synthesized and secreted.
CLINICAL BOX 20–1 Synthetic Steroids
As with many other naturally occurring substances, the activity of adrenocortical steroids can be increased by altering their structure. A number of synthetic steroids are available that have many times the activity of cortisol. The relative glucocorticoid and mineralocorticoid potencies of the natural steroids are compared with those of the synthetic steroids 9α-fluorocortisol, prednisolone, and dexamethasone in Table 20–2. The potency of dexamethasone is due to its high affinity for glucocorticoid receptors and its long half-life. Prednisolone also has a long half-life.
Steroid | Glucocorticoid Activity | Mineralocorticoid Activity |
---|---|---|
Cortisol | 1.0 | 1.0 |
Corticosterone | 0.3 | 15 |
Aldosterone | 0.3 | 3000 |
Deoxycorticosterone | 0.2 | 100 |
Costisone | 0.7 | 0.8 |
Prednisolone | 4 | 0.8 |
9α-Fluorocortisol | 10 | 125 |
Dexamethasone | 25 | –0 |
Trivial Name | P450 | CYP | Location |
---|---|---|---|
Cholesterol desmolase; side-chain cleavage enzyme | P450SCC | CYP11A1 | Mitochondria |
3β-Hydroxysteroid dehydrogenase | … | … | SER |
17α-Hydroxylase, 17,20-lyase | P450C17 | CYP17 | Mitochondria |
21β-Hydroxylase | P450C21 | CYP21A2 | SER |
11β-Hydroxylase | P450C11 | CYP11B1 | Mitochondria |
Aldosterone synthase | P450C11AS | CYP11B2 | Mitochondria |
Pregnenolone moves to the smooth endoplasmic reticulum, where some of it is dehydrogenated to form progesterone in a reaction catalyzed by 3β-hydroxysteroid dehydrogenase.
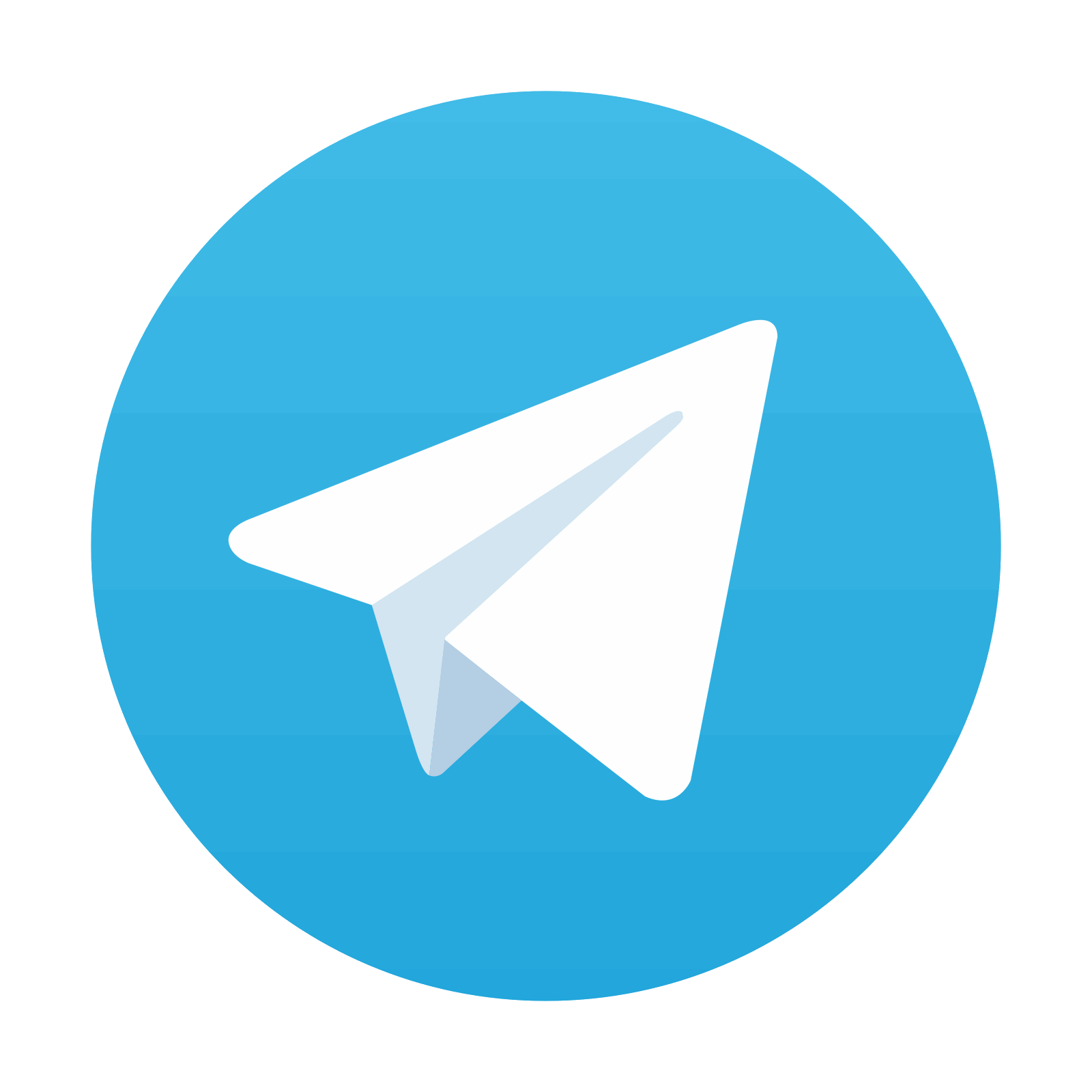
Stay updated, free articles. Join our Telegram channel

Full access? Get Clinical Tree
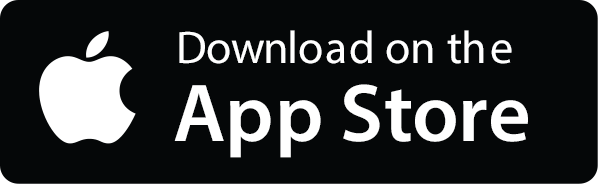
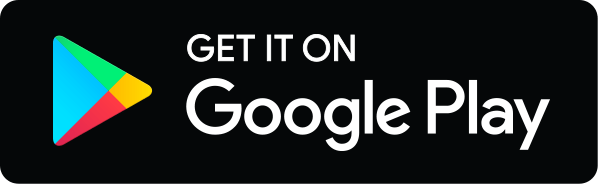
