17 Techniques in neuropathology
The components of the normal nervous system
The neuron is an excitable cell that is responsible for processing and transmitting information. Neurons communicate with each other via intercellular interfaces called synapses. At the synapse, an electrical impulse in the presynaptic neuron causes it to release a chemical transmitter that diffuses across a narrow gap to influence the electrical activity of the postsynaptic target neuron. Neurons have several components (Fig. 17.1):
• The cell body (or ‘soma’), which contains various subcellular organelles responsible for the metabolic upkeep of the cell.
• The axon, an elongated fibrous process that transmits electrical impulses away from the soma to synapses with either other neurons or muscle fibers. This may be a meter or more in length in the case of the lower motor neurons that reside in the lower (lumbar) spinal cord and innervate muscles of the lower leg.
• The dendritic tree, a plexus of cell process responsible for receiving synaptic inputs from other neurons.
• The nucleus, which resides in the cell body and is the site of storage of the cell’s genetic code in DNA.

Figure 17.1 Diagram showing a neuron with its component parts together with other cells of the central nervous system.
(Courtesy of Patrick Elliott of the Medical Illustration Department, Royal Hallamshire Hospital, Sheffield, UK.)
Two naturally occurring pigments may be observed to accumulate in the brain with age: lipofuscin and neuromelanin. Both are generally believed to represent cellular waste products. Lipofuscin is a yellow-brown, autoflourescent, granular substance composed of peroxidized protein and lipids. It is seen in larger neurons, such as the lower motor neurons of the spinal cord and pyramidal cells of the hippocampus in the context of Alzheimer pathology. Large amounts of lipofuscin-like pigment accumulate in the context of inherited neuronal ceroid lipofuscinoses, of which Batten’s disease is the most common (Goebel & Wisniewski 2004). Neuromelanin is most commonly seen in the cytoplasm of neurons of the substantia nigra and the locus ceruleus. It is the cause of the macroscopic pigmentation of these structures. Under the microscope, it is a dark brown, granular material that is believed to be the by-product of oxidative metabolism of catecholamines (Sulzer et al. 2008).
• Astrocytes, which have a number of functions. They maintain the extracellular ion and neurotransmitter balance. They are involved in repair and scarring responses to brain damage and also form part of the blood–brain barrier, which protects the brain from harmful blood-borne substances.
• Oligodendroglia, which form myelin, a phospholipid sheath around nerve axons that enhances the speed of conduction of impulses.
• Ependymal cells, which line the ventricles of the brain and central canal of the spinal cord.
Techniques for staining neurons
Tinctorial stains for Nissl substance
Hematoxylin and eosin (H&E) preparations demonstrate most important features of neurons. However, Nissl preparations are also popular for examining the basic architecture of neural tissue and its components. These are often combined with the luxol fast blue myelin stain. Granules of Nissl substance are found in the cell body (Fig. 17.1) and correspond to rough endoplasmic reticulum. They are basophilic due to the associated nucleic acid (Palay & Palade 1955). Many basic dyes (e.g. neutral red, methylene blue, azur, pyronin, thionin, toluidine blue and cresyl fast violet) stain Nissl substance. Variation in the stain used, pH and degree of differentiation allow preparations to label either Nissl substance alone, or Nissl substance in combination with cell nuclei.
Motor neurons generally have very coarse (‘tigroid’) Nissl substance, and regions such as the anterior horns of the spinal cord, where these cells are abundant, are good tissues to use when learning these stains (Fig. 17.2). For paraffin-embedded sections of formalin-fixed tissue, the cresyl fast violet stain is reliable and relatively straightforward. As such it is by far the most commonly used Nissl preparation. Toluidine blue may also be used, whilst Einarson’s gallocyanin method, being more suited to alcohol-fixed tissue, is largely unused (Kellett 1963).
Cresyl fast violet (Nissl) stain for paraffin sections
Preparation of stain
Cresyl fast violet | 0.5 g |
Distilled water | 100 ml |
Glacial acetic acid | 250 μl |
Alcohol | 100 ml |
Method
1. Dewax sections and bring to water.
2. Cover with filtered cresyl fast violet; stain for 10–20 minutes.
3. Rinse briefly in distilled water.
4. Differentiate in 0.25% acetic alcohol until most of the stain has been removed (4–8 seconds).
5. Briefly pass through absolute alcohol into xylene and check microscopically.
6. Repeat steps 4 and 5 if necessary, giving less differentiation when repeating.
Notes
a. If only Nissl substance is required to be demonstrated, the stain is acidified with 0.25% acetic acid.
b. Estimation of cortical neuronal density is made on 25 µm thick sections.
c. The cresyl violet method can be used as a counterstain when demonstrating myelin with the Kluver and Barrera method (see below).
Immunohistochemistry of neurons
1. Neuronal cytoskeletal proteins. Neurofilaments (NF) are intermediate filaments specifically expressed by mature neurons. They are composed of protein subunits that are classified by molecular weight into NF-L, NF-M, and NF-H which may be variably phosphorylated (Gotow 2000). Antisera raised against different neurofilament proteins in different states of phosphorylation are available. NF-H, in particular, and NF-M, to a lesser extent, are normally unphosphorylated in the neuronal cell body, but become phosphorylated in the axons. Thus, antibodies to phosphorylated NF-H mark axons but not cell bodies in normal nervous system tissues. Antibodies to non-phosphorylated neurofilament will label neuronal somata (Trojanowski et al. 1986). Microtubule-associated protein 2 (MAP-2) is a protein involved with microtubule assembly and is expressed by neurons in dendrites and cell bodies (Maccioni & Cambiazo 1995; Shafit-Zagardo & Kalcheva 1998). It is therefore often used to as a marker of neuroepithelial differentiation (Wharton et al. 2002; Blumcke et al. 2004).
2. Cytoplasmic proteins. PGP9.5 and neuron-specific enolase (NSE) are strongly expressed in neurons and can be reliably labeled by commercially available antisera. Unfortunately, they are not specific for neuronal cells, making interpretation tricky. They are best used in the context of a broad antibody panel (Ghobrial & Ross 1986; Wilson et al. 1988).
3. Neuronal nuclear proteins. NeuN is a neuron-specific DNA binding protein, which starts to be expressed around the time of initiation of terminal differentiation of the neuron (Mullen et al. 1992). Antibodies to NeuN therefore label neuronal nuclei, and neuronal components of other tumors (Edgar & Rosenblum 2008). However, in the context of neuro-oncology, it lacks specificity, being expressed to a variable degree in a diverse range of primary brain tumors. Therefore, NeuN is best used as part of a panel of antibodies in the investigation of clear cell primary brain tumors, but is of limited utility for other tumors (Preusser et al. 2006).
4. Proteins associated with neurosecretory granules. Antisera to these proteins can be useful to establish neuronal and neuroendocrine differentiation (Koperek et al. 2004; Takei et al. 2007). Synaptophysin is a membrane glycoprotein component of presynaptic neurosecretory vesicles. The cell body of normal neurons is usually unstained by synaptophysin (Fig. 17.3), resulting in early claims that cell body labeling was a feature of neoplastic neuronal cells that differentiated them from native neurons (Miller et al. 1990). However, it is now evident that a population of normal neurons also show cell body labeling which detracts from the use of this feature as a diagnostic marker (Quinn 1998). Synaptophysin is a useful marker of neuroendocrine differentiation and so also stains cells in metastatic neuroendocrine tumors (Wiedenmann et al. 1987). Chromogranin A is a protein of the dense core matrix of neurosecretory granules, and antibodies to it can be used to identify cells containing dense core vesicles (Nolan et al. 1985). It is therefore used predominantly to elucidate neuroendocrine differentiation in tumors.
Techniques for staining axons and neuronal processes
The annals of neurohistology describe several methods to demonstrate various special structures of the neuron, including axons (viable and degenerate), dendrites, synapses, dendritic trees and peripheral nerve endings. Many of these were block impregnation and free-floating frozen section methods and are rarely performed in time-constrained modern diagnostic laboratories. Immunohistochemistry has largely replaced the old silver preparations for the demonstration of axons as it is reliable and produces adequate results for diagnostic neuropathology with ease. However, for formal quantitation of axons, many still find that Palmgren’s method is superior to neurofilament immunohistochemistry (Chance et al. 1999). This technique has classically been used for staining axons of the peripheral nervous system. However, it is also an excellent preparation for staining central nervous system axons as well. Palmgren’s method uses potassium nitrate to suppress staining of reticulin. It is considered that with the Palmgren method, cresyl violet preparations and immunohistochemistry for neurofilament and MAP-2, there is no longer requirement for older silver preparations such as that of Bielschowsky (Bielschowsky 1902) and Marsland (Marsland et al. 1954).
Modified Palmgren’s method for nerve fibers in paraffin-embedded material (Palmgren 1948)
Preparation of solutions
Acid formalin
Concentrated formaldehyde (40% w/v) | 25 ml |
Distilled water | 75 ml |
1% nitric acid | 0.2 ml |
Silver solution
30% silver nitrate | 25 ml |
20% potassium nitrate | 25 ml |
5% glycine | 0.5 ml |
Method
1. Take sections to distilled water.
2. Treat sections with acid formalin for 5 minutes.
3. Wash in three changes of distilled water for 5 minutes.
4. Leave in filtered silver solution for 15 minutes at room temperature.
5. Without rinsing, drain the slide and flood the section with reducer that has been heated to 40–45°C. Rock the slide gently and add fresh reducer. Leave for 1 minutes. A beaker placed on a hot plate is useful for this stage.
6. Wash in three changes of distilled water. Examine microscopically and, if necessary, repeat from step 4, reducing the time in the silver solution and decreasing the temperature of the reducer to 30°C.
8. Fix in 5% sodium thiosulfate, 5 minutes.
Examination of axons in peripheral nerve in diagnostic neuropathology now relies largely on toluidine blue-stained semi-thin resin-embedded tissue. Capricious techniques such as Eager’s method for detecting degenerating axons are no longer in use (Eager et al. 1971).
The Golgi preparation and its variants are excellent for the visualization of the three-dimensional nature of the neuron and its dendritic processes. However, modern diagnostic neuropathology practice has no requirement for this. Golgi techniques are occasionally used in research (e.g. Garey 2010) although new antisera are increasingly allowing immunohistochemical indices of these aspects of cell morphology. It is suggested that the interested reader consider the Pugh and Rossi modification for use on paraffin-embedded tissue (Pugh & Rossi 1993) if a Golgi stain is to be attempted.
Myelin
Modern tinctorial stains for myelin are simple and reliable and can be performed on formalin-fixed paraffin-processed tissue. Many can be combined with a Nissl stain to demonstrate neuronal localization. Older methods may give more even and consistent staining, but are considerably more time consuming and have fallen from use (Weigert 1904; Loyez 1910; Weil 1928). Both luxol fast blue and solochrome cyanine preparations are now favored.
Luxol fast blue is a copper phthalocyanine dye which is employed in myelin staining of paraffin-processed tissue (Kluver & Barrera 1953). This can be combined with cresyl violet or hematoxylin to outline cellular architecture (Fig. 17.4) or with periodic acid-Schiff (PAS) to demonstrate myelin degradation products in demyelinating disease. It can be used on central nervous system tissue only.
Luxol fast blue stain for myelin with cresyl violet counterstain (Kluver & Barrera 1953)
Preparation of solutions
Cresyl violet stock solution
Cresyl violet | 0.5 g |
Distilled water | 100 ml |
Method
1. Take sections on slides to 95% alcohol (not water).
2. Stain in luxol fast blue solution, 2 hours at 60°C, or 37°C overnight.
5. Differentiate in 0.1% lithium carbonate solution until the gray and white matter are distinguished. This may be more easily controlled by using 0.05% lithium carbonate followed by 70–95% alcohol instead.
7. Check differentiation under the microscope. Repeat step 5 if necessary.
8. Stain in cresyl violet solution, 10–20 minutes.
9. Drain sections and transfer to 70% alcohol. Avoid placing the section in water at this stage as the cresyl violet staining loses some of its intensity. Gently agitate the sections; the cresyl violet dye will flood out. The 70% alcohol differentiates the cresyl violet stain. Optimally, the cresyl violet should be removed, leaving the cell bodies and Nissl clearly visible. Do not over-differentiate; the 70% alcohol will take out the cresyl violet and to a certain extent the luxol fast blue. The cresyl violet counterstain will deepen the color of the luxol fast blue stained myelin from turquoise to a deep blue.
Notes
a. If the section is over-differentiated with lithium carbonate/alcohol, the section can be restained with the luxol fast blue and then differentiated to obtain the optimum staining result. This may apply to tissues which have very low amounts of myelin (e.g. baby/neonatal brains). These tissues can be very challenging in achieving optimum staining. Unfortunately, once the cresyl violet counterstain has been applied the over-differentiation cannot be rectified.
b. Some histologists prefer to differentiate the cresyl violet using 0.25% acetic acid in 100% alcohol.
c. The use of thick sections is important for the visualization of myelin tracts.
d. Other counterstains may be used such as neutral red. This will result in the myelin appearing purple/blue due to a slightly different color balance, and is a matter of personal preference.
Page’s solochrome cyanine technique for myelin in paraffin sections
Preparation of solution
Solochrome cyanine RS | 0.2 g |
Distilled water | 96 ml |
10% iron alum | 4 ml |
Concentrated sulfuric acid | 0.5 ml |
Method
Immunohistochemistry for S-100 is useful in the diagnosis of tumors derived from Schwann cells both in the central nervous system and peripherally (Hirose et al. 1986; Winek et al. 1989). Many antisera are used as markers of myelination, the most useful being myelin basic protein and myelin associated glycoprotein (Itoyama et al. 1980a, 1980b; Ludwin & Sternberger 1984; Lindner et al. 2008). However, given the reliability and simplicity of the tinctorial stains, immunohistochemical myelin makers are largely unused in routine diagnostic neuropathology and remain the preserve of research laboratories.
Myelin loss may occur in a region of brain damaged by any of a number of processes such as trauma, neoplasia, multiple sclerosis or toxic insult. It may also occur secondary to the loss of axons emanating from a lesioned brain region. In modern practice, degeneration of myelinated tracts is most commonly demonstrated by showing loss of normal myelin staining by either luxol fast blue or solochrome cyanine preparations, or by showing a microglial reaction using CD68 immunohistochemistry (e.g., Ince et al. 2008). Historically, the Marchi technique (Swank & Davenport 1935) and neutral lipid stains have been used to detect early and late myelin degeneration products, respectively. However, the Marchi technique requires block staining or free-floating sections and lipid stains cannot be performed on paraffin-embedded material. Given these issues, the sensitivity of CD68 immunohistochemistry and the ease of the tinctorial preparations for normal myelin (see above), the Marchi and neutral lipid techniques have been rendered obsolete.
The neuroglia
Ependymal cells
Ependymal cells are epithelioid and line the ventricles of the brain and the central canal of the spinal cord. They are easily located with conventional stains such as H&E and immunohistochemistry for GFAP, vimentin and S-100. Immunohistochemistry for epithelial membrane antigen (Uematsu et al. 1989; Hasselblatt & Paulus 2003) labels both normal and neoplastic ependymal cells, whilst cytokeratin markers are negative.
Astrocytes
Astrocytes have multiple, fine processes and (in their reactive state) are ‘star-shaped’ (hence the name). On standard H&E sections, only the nucleus of resting astrocytes is distinct, as the cell body cannot be discerned from background neuropil. These nuclei are slightly larger with more open granular chromatin than that of the more compact oligodendrocyte. Modern neuropathology relies most heavily on GFAP (Fig. 17.5) immunohistochemistry for demonstration of astrocytes, although antibodies to S-100, αB-crystallin and glutamine synthetase may also be used. Metal-based and tinctorial methods such as Cajal’s gold sublimate, PTAH (Chan & Lowe 2002) and Holzer (1921) are no longer in use as these are variously more expensive, more technically demanding or less specific than their immunohistochemical equivalents.
In neuro-oncology, astrocytic differentiation is best demonstrated by GFAP immunohistochemistry. GFAP immunoreactivity is also seen in other tumors including ependymoma, oligodendroglial tumors and choroid plexus tumors (Eng & Rubinstein 1978; Velasco et al. 1980; Eng 1983; Doglioni et al. 1987). Astrocytic tumors also label with vimentin and S-100, but these are also seen in many other tumor types, rendering them of little use for differential diagnosis. Astrocytes occasionally show cross-reactivity as seen for the pan cytokeratin AE1/AE3 (Cosgrove et al. 1989). Therefore, it is expedient to use other cytokeratin markers such as CAM5.2 or MNF116 to exclude the diagnosis of epithelial cell tumors, such as metastatic adenocarcinoma.
An emerging and potentially more powerful tool for the diagnosis of diffuse oligodendroglial and astrocytic neoplasms is the use of antibodies to isocitrate dehydrogenase 1 (IDH1) carrying the R132H mutation. This is the most frequent mutation in diffuse gliomas (Hartmann et al. 2009). There is an emerging literature that appears to demonstrate that antisera to this mutant protein may be used to differentiate reactive gliosis from grade II and III astrocytomas (Camelo-Piragua et al. 2010; Capper et al. 2010) and oligodendrogliomas from lesions with similar morphologic appearances (Capper et al. 2011).
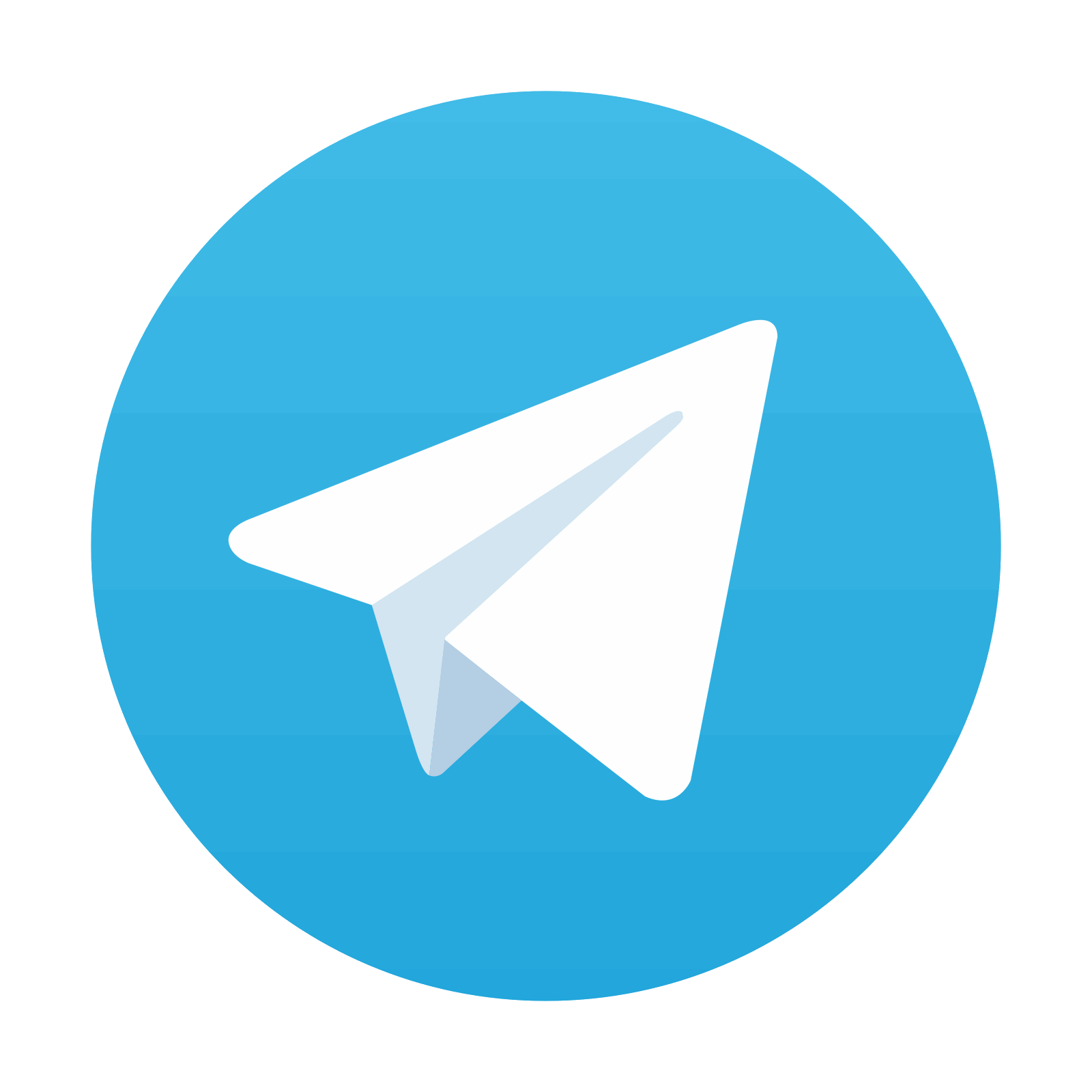
Stay updated, free articles. Join our Telegram channel

Full access? Get Clinical Tree
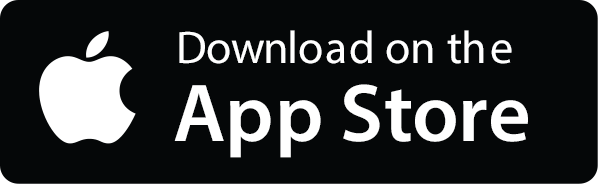
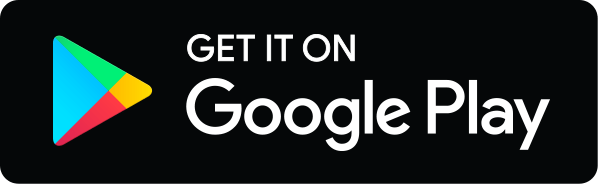