Fig. 16.1
Structural representations of water
Carbohydrates are composed of oxygen, hydrogen, and carbon. There are various types of carbohydrates, the most important of which in biological systems is glucose (C6H12O6) (Fig. 16.2). Even among the monosaccharides, there are several isomers such as fructose, galactose, mannose, and so forth. Oligosaccharides and polysaccharides are composed of monosaccharides connected by glycosidic bonds. Saccharides have versatile roles in living systems and are a huge research topic that incorporates energy storage and molecular recognition in biological processes. Nucleotides are composed of ribose or 2-deoxyribose, a nucleobase (purine or pyrimidine base) (Fig. 16.3), and phosphate groups. Adenosine diphosphate (ADP) and adenosine triphosphate (ATP) are the key molecules in energy transduction in biological systems. The energy evolved in the dissociation reaction of ATP into ADP is used in a wide range of biochemical reactions. There are 20 amino acids that are the subunits of proteins, with peptide linkages connecting the amino acids. It is of great importance to classify the amino acids into those with hydrophilic and hydrophobic groups (Fig. 16.4), more rigorously, into aliphatic amino acids (gly, ala, val, leu, ile), hydroxyl or sulfuric amino acids (ser, cys, thr, met), aromatic amino acids (phe, tyr, trp), cyclic amino acid (pro), basic amino acids (lys, his, arg), and acidic amino acids (asp, glu, asn, gln). The variation of the content of each amino acid and the order of the connections give different proteins; moreover, the solvent properties (pH and ionic strength) as well as the environment (temperature and pressure) affect the molecular structure and functions of proteins.





Fig. 16.2
D-Glucose in Fischer projection and in cyclic form (α-d-glucose)

Fig. 16.3
Nucleobase (adenine [A], guanine [G], cytosine [C], uracil [U], and thymine [T]). R- refers to the position connecting to ribose or deoxyribose in RNA or DNA


Fig. 16.4
Chemical structure of 20 amino acids
16.1.2 Supplement I. Polymer
Polymers are huge molecules consisting of a large number of monomers (subunits). The simplest polymer is linear saturated hydrocarbons containing C–C covalent bonds. The distance between carbon atoms and the angle between successive bonds are 0.154 nm and
, respectively, because of the tetrahedral structure of the carbon atoms (Fig. 16.5). The bonds have a freedom of rotational motion around the C–C linkages (internal rotation of the polymer chain), although the bond length and the angle are fixed. The angle of rotation ϕ is defined by the plane formed by the first three carbon atoms and is measured in the clockwise direction from the plane. When a carbon atom is located at the angle
, i.e., on the reference plane, it is called the trans position (t). For the case of polyethylene, the minimum potential energy is obtained at the angle
. This is called the gauche position (g +, g −). There is an energy difference Δε between the t position and the g + (or g −) position, which determines the average population of carbon atoms in thermal equilibrium. This is related to the flexibility of the polymer chain. The average length λ of a continuous trans sequence is expressed as


where l is the distance of the C–C bond, k B is the Boltzmann constant, and T is the absolute temperature.




Fig. 16.5
Illustration of the internal rotation ϕ in a polymer chain and the potential energy as a function of rotation angle

(16.1)
The frequency of the transitions between different isomeric structures is determined by the potential barrier ΔE. The specific time for the transition from the t position to the g + (g −) position is given by

Here, τ 0 is the microscopic time scale of the torsional vibration of the C–C bond. Polymers take values of Δε ~ 4 kJ mol−1 and ΔE ~ 16 kJ mol−1, although the barrier energy ΔE depends on the side group of the chain, the existence of hydrogen bonds, and other factors. The chemical species of the side chain and the order of connections of subunits vary enormously. Among the polymers produced by biological activity, several biopolymers have been classified into polysaccharides, polynucleotides, proteins, and so forth. The relaxation time of polymers and biopolymers depends on the molecular weight and other factors, which induce slow dynamics [2, 3] .

(16.2)
16.1.3 Supplement I. Colloid
Colloids refer to the dispersed phase of two-component systems in which particles in the dispersed phase are usually smaller than micrometer order. Because of the small particle (or droplet) size in the dispersed phase, the motion of particles is affected by thermal forces. Their properties are also strongly affected by the chemical environment at the interface between the particles and the medium. Particles interact with each other throughout the dispersion or at the surface, owing to the presence of depletion and hydrodynamic forces. Hydrodynamic interactions are caused by a disturbance of the field of fluid flow by the presence of particles. The depletion force arises from the polymer or other substances and even from ions surrounding the particles in the medium and leads to attraction between particles. Therefore, colloidal dispersions provide a rich field of research in rheology from both fundamental and industrial aspects. Colloids are considered to be an interesting system for modeling atoms in physics, since the micrometer size of colloidal particles makes them large enough to be observed by optical techniques. For example, Brownian motion and the related studies by Einstein and Smoluchowski were employed to prove the existence of atoms. Furthermore, colloids have been utilized as model systems to study diffusion, aggregation, sedimentation, and other phenomena.
16.1.4 Supplement I. Micelle, Lipid Bilayer, Emulsion, and Microemulsion
Molecules interact with each other in condensed matter to make various types of stable and quasi-stable assemblies or aggregated states in biological systems. When the process yielding these complexes is based on the principle of the second law of thermodynamics, i.e., the principle of the lowest free energy, the process is called self-organization or self-assembly. Micelles are formed by amphiphilic molecules such as surface active agents and block copolymers.
The self-assembly of amphiphilic molecules such as surfactants, lipids, and copolymers provides various types of ordered structures in water. Micellar structures develop spontaneously from surfactants in aqueous solutions above the critical micelle concentration (CMC) (Fig. 16.6). The biological membranes in cells are formed by phospholipids, and cellular membranes are known to have a lipid bilayer structure (Fig. 16.7). Mixtures of two immiscible liquids often form emulsions owing to the effect of surface active agents called emulsifiers (or surfactants). Normally, emulsions are not formed spontaneously because they are unstable energetically. Thus, some energy must be input to form emulsions, such as by stirring or homogenizing, so that the droplets of a liquid can be dispersed in the continuous phase of another liquid. When the size of the droplets is of nanometer or micrometer orders, the emulsions are called nanoemulsions and microemulsions, respectively. When oil droplets are dispersed in a water phase, the emulsion is called an oil-in-water emulsion and vice versa. Emulsions are often observed in foods (butter and mayonnaise), cosmetics, and pharmaceuticals (waxes, creams, and lotions).



Fig. 16.6
Schematic illustration of surfactant and micelle structure

Fig. 16.7
Schematic illustration of lipid bilayer
16.1.5 Supplement I. Gel, Microcapsule, and Liquid Crystal
Gels are a solid three-dimensional network consisting of polymers and colloids, which include solvent molecules inside the network. The connections in the network are made of functional groups and associative groups, which are called cross-links. Usually, gels show solid-like mechanical properties, although they are mainly composed of liquid in terms of their volume and mass. Networks formed by the cross-linking of primary polymers are called the polymeric gels, while gels made from colloidal particles or aggregated particles are called particulate gels. When the primary molecules consist of biopolymers, they are called biopolymer gels. Chemical gels have covalent bonds at the cross-links; thus, the connections cannot easily be broken, and the cross-links have a permanent structure throughout their lifetime. In contrast, in physical gels, the cross-links comprise physical interactions. In physical gels, even in equilibrium, the connected and disconnected states of cross-links have an average lifetime. Therefore, the time window in experiments is an important factor when studying the mechanical and rheological properties of gels (Fig. 16.8) [4].


Fig. 16.8
Schematic illustrations of gel network made of covalent bonds and physical association as cross-linker
Microcapsules are spherical entities whose diameter is typically micrometer or submillimeter that have a core (internal phase) with a thin surrounding wall membrane. Thus, the core is isolated or partially isolated from its surrounding environment. Microcapsules are utilized to control the rate of release of the internal phase through the wall membrane and are thus used as drug delivery systems. The wall is normally composed of polymers, the cross-linking of which is proceeded in the preparation process. Therefore, the polymer network is more or less swollen with the solvent. This means that the thin wall membrane of microcapsules is mostly in the gel state.
Liquid crystals are also in an intermediate state between liquids and solids. The molecules of a liquid crystal are oriented in an ordered structure similarly to those in a crystal, while they flow similarly to a liquid. There are various types of liquid crystals consisted of biological and synthetic materials. Electronic displays are widely used as an application of liquid crystals. From a biological viewpoint, lipids, carbohydrates, nucleic acids, and proteins often form a liquid crystal and change their state according to changes in the thermodynamic environment. For example, spider silk has low viscosity, allowing it to flow in the liquid crystalline phase, although it becomes a fiber to form a web after extrusion.
16.1.6 Supplement I. Tissue and Organ
Cells are the building blocks of living organisms. Most cells are visible under an optical microscope, have micrometer-order size, and consist of a protoplasm enclosed in a plasma membrane. An ensemble of similar cells from a tissue has a specific function. Organs are constructed from multiple tissues. Organs have a specific and complicated structure in which numerous molecules aggregate to form cells, tissues, and organs.
16.2 Supplement II. Brief Review of the Classical Theory in Relation to Rheological Measurements
Rheology at the nano- and microscale is associated with the transport, diffusion, aggregation, and relaxation behavior of molecules. The rheological behavior of each system is unique when it undergoes a phase transition or glass transition. The methodologies for studying rheological properties applied to nano-/micro-science and technology need to be understood in relation to the force and molecular motion in systems together with the principles of statistical physics and equilibrium/nonequilibrium thermodynamics. In this Sect. 16.2, a general survey of classical theory together with experimental principles and related phenomena is described.
16.2.1 Supplement II. Force
The structural formation and biological functions of molecules are induced by various forces in biological systems. In most experiments, a stimulus is given to a system to obtain physical quantities as a response. The forces used in experiments include mechanic, electronic, magnetic, thermal force, and so forth.
A fundamental definition of the van der Waals interaction between two molecules is the attractive force formulated in quantum mechanics. In practice, the potential V 12(r), which describes the dispersive force between molecules 1 and 2, is a function of the distance r between two molecules,

Here, A 12 is the Hamaker constant. This implies that there is a characteristic length scale that decays as 1/r 6 relative to the potential with the constant of proportionality, A 12. The Hamaker constant A 12 is a system-dependent quantity. Typically, the strength of van der Waals forces is on the order of 1 kJ mol−1, which is relatively weak compared with other forces. The Lennard-Jones potential is often used for molecular dynamics simulations as the energy of the interaction,
![$$ U(r)=4\varepsilon \left[{\left(\frac{\sigma }{r}\right)}^{12}-{\left(\frac{\sigma }{r}\right)}^6\right], $$](https://i0.wp.com/basicmedicalkey.com/wp-content/uploads/2018/03/A314557_1_En_16_Chapter_Equ4.gif?w=960)
where r is the distance between the particles and ε and σ are characteristic constants.

(16.3)
![$$ U(r)=4\varepsilon \left[{\left(\frac{\sigma }{r}\right)}^{12}-{\left(\frac{\sigma }{r}\right)}^6\right], $$](https://i0.wp.com/basicmedicalkey.com/wp-content/uploads/2018/03/A314557_1_En_16_Chapter_Equ4.gif?w=960)
(16.4)
Hydrogen bonds are stronger than van der Waals force, and their energy is in the range of 10–40 kJ mol−1, although they are still weaker than ionic interactions and covalent bonds by an order of magnitude. Hydrogen bonds are formed between the proton-donating groups in molecules and the proton-accepting atoms. The donor group is a polar group such as O–H, N–H, or S–H, whereas the acceptor is an electronegative atom such as oxygen, nitrogen, or sulfur. Hydrogen bonding plays a key role in the molecular dynamics of aqueous systems such as micelles, membrane formation in biological cells, and in the higher-order conformation of proteins and polynucleotides.
Coulomb’s law is the fundamental formula used to consider the electrostatic interactions in systems. The interaction energy E between two point charges at a distance r is

where q 1 and q 2 are the magnitudes of the point charges and ε r and ε 0 are the relative dielectric permittivity of materials and the dielectric permittivity in vacuum, respectively. The electrostatic force propagates over a long distance and is often referred to as a long-range interaction, and the strength of ionic bonds between molecules is typically on the order of 100 kJ mol−1.

(16.5)
The screening effect of the Coulombic force is also an important factor for aqueous solutions. An electric double layer forms around a charged group, which screens the Coulombic interactions of electrolytes in aqueous solutions. The screening effect is considered using the characteristic length scale (
) defined by the Debye screening length,

Here, z is the valence of the counterion, e is the electronic charge, and ρ is the charge on the surface.


(16.6)
16.2.2 Supplement II. Molecular Motion and Diffusion
The Arrhenius equation, which is equivalent to the Eyring equation, is widely accepted as an empirical relationship holding in various physical and chemical phenomena such as the temperature variation of the relaxation of molecular motion, the diffusion, mechanical relaxation of materials, common chemical reactions, and other thermally induced processes/reactions. It gives a fundamental means of describing the relationship among the rate constant k of various processes/reactions, the activation energy E a, and the absolute temperature T,

Here, A is the prefactor, and k B is the Boltzmann constant. The rate constant k indicates the number of collisions resulting in a process or reaction per second, A is the total number of collisions per second, and the term
gives the probability of observing collisions in the reaction or process.

(16.7)

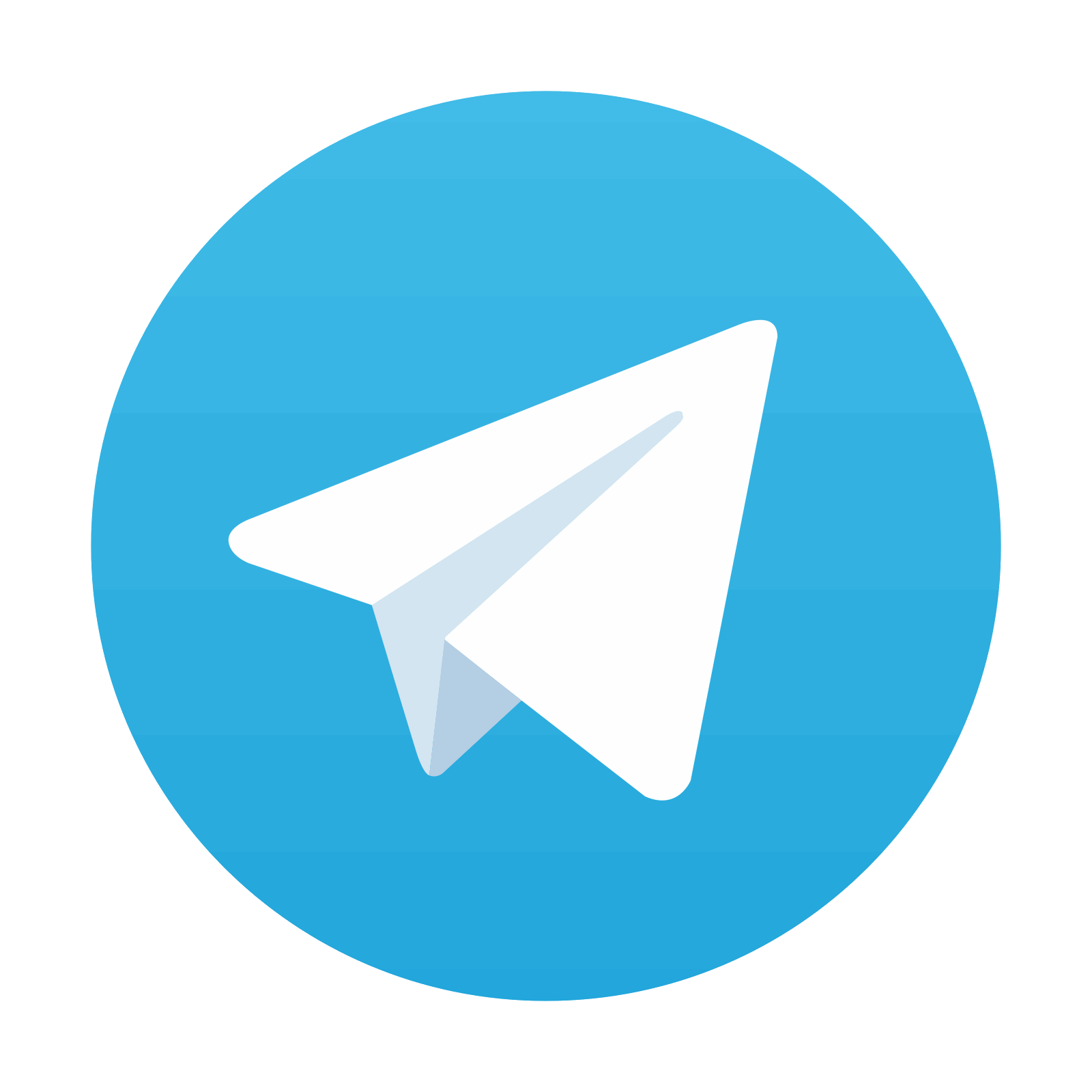
Stay updated, free articles. Join our Telegram channel

Full access? Get Clinical Tree
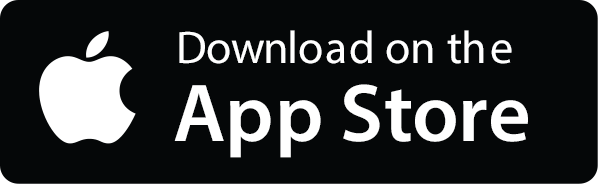
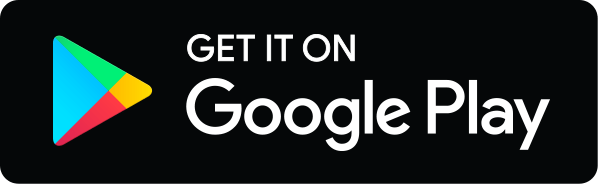