the ventral tegmental area, which communicates with the nucleus accumbens via the medial forebrain bundle,
The reward pathway is activated by impulses arising in the ventral tegmental area of the brain. These impulses are relayed through the medial forebrain bundle, via the nucleus accumbens, to the prefrontal cortex (Fig. 54.1). Activation of the ventral tegmental area results in dopamine release in the nucleus accumbens and stimulation of postsynaptic D2 receptors (acting via inhibitory Gi proteins to inhibit the generation of intracellular cAMP). This is thought to be central to the processes of motivation and reward.
Fig. 54.1 The role of dopamine and cAMP in reward pathways in the mesolimbic system and the relevance of these pathways to substance abuse.
This diagram illustrates only a small part of the complex mechanisms involved in the processes of reward and the ways that substances of abuse influence these pathways, leading to dependence and withdrawal effects. Dopaminergic neurons in the ventral tegmental area release dopamine (DA) at the nucleus accumbens, which decreases cAMP and its activation of the transcription factor, cAMP response element-binding protein (CREB). Many substances of abuse when administered acutely increase DA and consequently inhibit cAMP, providing the pleasurable and rewarding effects of the drug. However, chronic persistent intake eventually increases CREB and dynorphin (DYN), which will dampen reward mechanisms in the nucleus accumbens, providing a possible mechanism for drug dependence and tolerance (see further explanations in the text). GABA, γ-aminobutyric acid; GLU, glutamate.
Occasional and limited administration of most drugs of potential abuse directly or indirectly releases dopamine in the nucleus accumbens (Fig. 54.1). For example, morphine enhances dopaminergic input to the nucleus accumbens by stimulating opioid receptors in the ventral tegmental area. This effect may eventually drive the processes, resulting in drug dependence, because with repeated use, the drug-related dopamine release becomes essential to maintain a ‘normal’ level of pleasure.
Chronic stimulation of the mesolimbic dopamine reward pathways
The complexities of the changes involved are daunting and only a limited description of these events is given here. Chronic exposure of the mesolimbic system to drugs such as opioids, alcohol and cocaine eventually leads to neuro-adaptive changes and sensitisation of the mesolimbic system to further drug administration. Upregulation of neuronal cAMP in the nucleus accumbens results from chronic exposure to many drugs of abuse, with increased intraneuronal cAMP response element-binding protein (CREB). Increased CREB in the nucleus accumbens is probably important for the development of tolerance and dependence. CREB also activates dysphoria-inducing κ-opioid receptors that bind the opioid peptide dynorphin (Ch. 19) on dopamine- and glutamate-releasing neurons in the prefrontal cortex. The long-term actions of dependence-inducing drugs also affect plasticity in the neural circuits of the reward pathway. Upregulation of transcription factors such as CREB and ΔFosB leads to long-term changes in brain-derived neurotrophic factor (BDNF), which regulates the number of dendrites on various neurons in the pathway. Therefore, the changes in the reward and stress systems in the brain that arise with addiction may become ‘imprinted’ even if the causative drug is stopped for long periods. This would explain the vulnerability to relapse after detoxification. There are probably genetic influences on the neurochemical events involved in the reward pathways and stress systems that also increase susceptibility to addiction.
Drug craving is also influenced by neural inputs to the ‘reward’ pathway from the amygdala, which are involved in emotion and conditioned responses. In particular, the amygdala is central to the reinforcing effects of drug binges and also the anxiety and negative effect involved in acute withdrawal. Conditioned responses provide powerful cues to drug-taking in specific social circumstances, and the conditioning is reinforced by aspects of the drug-taking process. Eventually, learning that a drug withdrawal can produce adverse effects that are relieved by the drug may lead to any source of stress or frustration becoming a cue for drug use. Dependence is associated with recruitment of stress systems in the brain on drug withdrawal, probably in an attempt to restore normal neuronal function. There is an elevation of corticotropin-releasing hormone (CRH) and noradrenaline, with suppression of the anti-stress neuropeptide Y.
In contrast, physical dependence on a drug is unrelated to activity in the mesolimbic system and arises from excessive noradrenergic output from the locus ceruleus, a structure in the base of the brain that is involved in arousal and vigilance.
This chapter covers drugs that are encountered in clinical practice primarily because of their abuse, such as ecstasy and cannabis, or because of their potential to cause dependence, such as nicotine and ethanol (Box 54.1).
Drugs of abuse
Several drugs that have central stimulant properties are abused and produce dependence. Those more commonly encountered are considered here.
Cocaine
Cocaine is an alkaloid obtained from the leaves of the coca plant. It is usually taken as the hydrochloride salt. ‘Crack’ cocaine is the free-base form, named after the crackling sound produced when it is smoked.
Mechanism of action and effects: The psychomotor effects of cocaine are due to inhibition of reuptake transporters for monamines in presynaptic nerve terminals, particularly inhibiting the dopamine reuptake transporter (DAT), and to a lesser extent those for noradrenaline (NET) and serotonin (SERT). This in turn may activate opioid systems in the brain, with upregulation of µ-receptors (Ch. 19). Increased dopaminergic activity in the reward pathway promotes dependence. Changes in various pituitary neuroendocrine functions occur with more prolonged use; in particular, the release of corticotropin and luteinizing hormone (LH) are enhanced. Tolerance to the psychomotor effects of cocaine is limited. One of the metabolites of cocaine, norcocaine, has direct vasoconstrictor activity.



Pharmacokinetics: Cocaine, as the hydrochloride salt, is used orally, intranasally (cocaine snuff) or by intravenous injection; the intravenous route gives an intense and rapid onset of effect. ‘Crack’ cocaine is prepared by mixing cocaine hydrochloride with sodium bicarbonate or ammonia and water, then heating to volatilise the free base. The product is smoked and produces intense effects similar to intravenous use. Cocaine is metabolised by plasma and liver esterases and its half-life is very short.
Amfetamine and derivatives
Amfetamine, dexamfetamine, methamfetamine and 3,4-methylenedioxymetamfetamine (MDMA, ‘ecstasy’) have little medical value. Dexamfetamine is sometimes used as a treatment for attention deficit hyperactivity disorder (ADHD) (Ch. 22).
Mechanism of action and effects: Amfetamine and related drugs have indirect sympathomimetic effects, releasing monoamines from central nervous system (CNS) neurons (Ch. 4). They are taken up into presynaptic nerve terminals where they block vesicular uptake of dopamine, serotonin and noradrenaline by the vesicular monamine transporters (VMATs), increasing their concentrations in the cytoplasm. This induces release of the monoamines into the synapse by reversing the respective reuptake transporters for dopamine (DAT), serotonin (SERT) and noradrenaline (NET) in the neuronal membrane. CNS stimulation by amfetamine is most marked in the reticular formation although it also occurs in many other areas of the brain including the reward pathway. The D-isomer (dexamfetamine) is twice as potent as the L-isomer of amfetamine in its central stimulant activity. Effects of amfetamine include:




MDMA (ecstasy) is more selective than amfetamine for serotonin release, and produces euphoria similar to that of amfetamine but with less stimulant activity. Direct agonism of serotonin (5-hydroxytryptamine) 5-HT1 or 5-HT2 receptors may contribute to its effects, including release of oxytocin associated with the euphoric action. Disturbance of thermoregulatory homeostasis occurs, leading to a syndrome resembling heat stroke with hyperthermia and dehydration, usually after exertion in hot environments. Stimulation of antidiuretic hormone release can cause thirst and water retention with subsequent water intoxication and hyponatraemia. The toxic effects of MDMA include cardiac arrhythmias, seizures, muscle damage and severe metabolic acidosis, which may be fatal. The long-term toxicity may include memory impairment, anxiety or depression.
Pharmacokinetics: Although amfetamine is sometimes used intravenously or via nasal inhalation, absorption from the gut is rapid and complete. Amfetamine readily crosses the blood–brain barrier. About half is excreted unchanged in the urine and the rest is metabolised in the liver. Amfetamine is a basic drug and its half-life varies according to urine flow and pH; at low urine pH, greater ionisation of amfetamine increases its excretion, producing a shorter half-life, whereas at high urine pH the half-life is longer because of greater reabsorption of the non-ionised drug in the renal tubule. Metabolites of amfetamine are believed to contribute to the psychotic effects seen with long-term use.
Ecstasy is usually taken orally. It undergoes hepatic metabolism via CYP2D6, and polymorphism of this enzyme may explain some of the serious intoxication that occurs with the drug, although the half-life does not differ much between poor and extensive metabolisers (about 5 h in both).
Cathinone stimulants
Mechanism of action: Cathinone and cathine are derived from the khat plant, and there are several similar synthetic compounds such as mephedrone, methylone and flephedrone that are used recreationally. They probably stimulate release of monoamines from neuronal vesicles and inhibit their reuptake. Their psychoactive and physical effects resemble those of amfetamines. They are used intranasally or orally, although they can be injected, smoked or taken rectally.
Nicotine and tobacco
Mechanism of action: Over 300 chemical compounds are present in tobacco smoke, but the actions of nicotine are central to the addictive pharmacological effects of smoking. Nicotine has dose-related peripheral actions. At low doses, stimulation of aortic and carotid chemoreceptors enhances sympathetic nervous system activity, and at higher doses there is direct stimulation of the nicotinic N1 receptors in autonomic ganglia (Ch. 4). At even higher doses, nicotine acts as a ganglion-blocking agent. Initial stimulation of autonomic nervous tissue is therefore followed by depression. Effects on the CNS are mediated by presynaptic nicotinic receptors structurally distinct from those in the periphery. Stimulation of CNS nicotinic receptors increases neuronal permeability to Na+ or Ca2+ and enhances the release of glutamate, which promotes dopamine release. With prolonged use nicotine inhibits the release of γ-aminobutyric acid (GABA). Nicotinic receptors are found in the mesocortical and mesolimbic dopaminergic systems, in projections from the ventral forebrain to the cortex that mediate arousal and in hippocampal projections where stimulation enhances learning and short-term memory. Tolerance to the CNS effects of nicotine is rapid due to receptor desensitisation.
Effects of nicotine and tobacco: Tobacco components, including nicotine, have effects on a number of organ systems.
Respiratory effects: The lungs are the first area to be in contact with the chemical components of tobacco smoke and are also exposed to particles and gases. Tars and other irritants, rather than nicotine, are responsible for the chronic damage to the lungs.






Psychological effects: The psychological effects of smoking are substantial, as indicated by the difficulties experienced by those attempting to quit.
Other effects: Nicotine and smoking have a number of other effects.

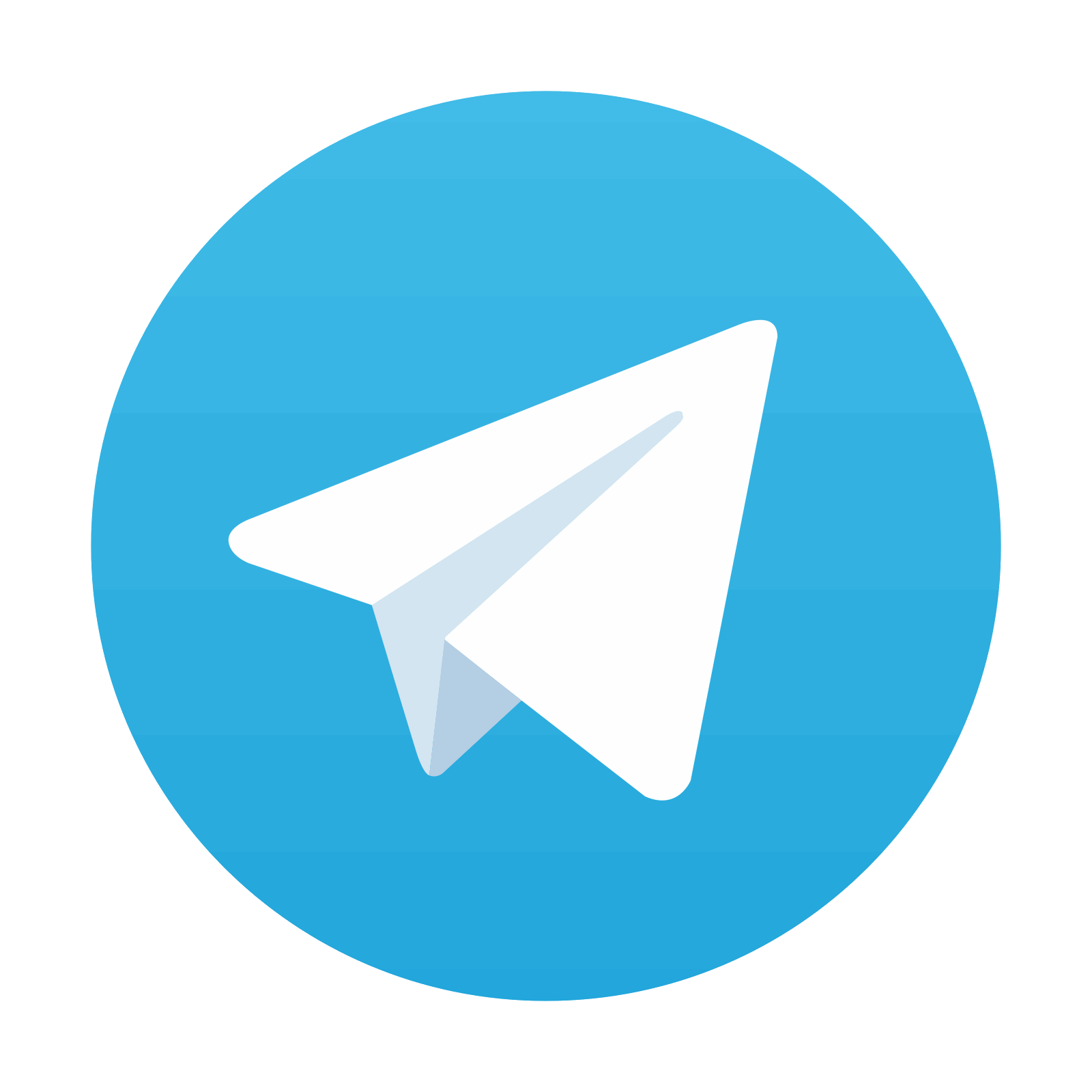
Stay updated, free articles. Join our Telegram channel

Full access? Get Clinical Tree
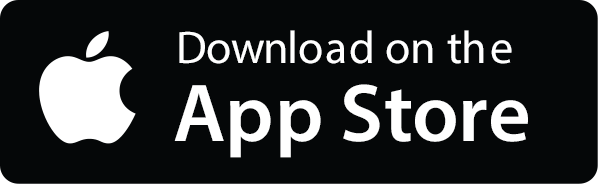
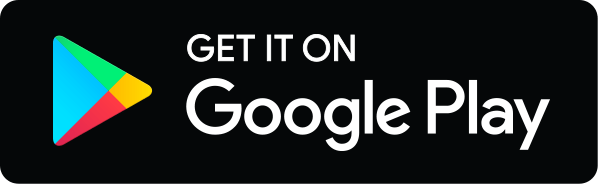
