Fig. 12.1
Proposed model for AFSC-mediated tissue repair. Implanted undifferentiated AFSCs evoke a strong pro-inflammatory and pro-angiogenic response, which accounts for the rapid vascularization of the implantation site. Circulating progenitors are recruited through the neo-formed vessels. At the same time, tissue-resident progenitors can be “activated,” while AFSCs are progressively cleared out. The regeneration is endogenous in nature, meaning that AFSCs only function as an initial burst and host progenitors fulfil the repair
We showed for the first time that human AFSCs recruit host progenitors when implanted in the subcutis of nude mice [56]. In that study, more than two million AFSCs were incorporated in porous ceramic scaffolds with fibrin glue and subcutaneously implanted in mice. Animals were sacrificed 1, 2, 4, and 8 weeks after implantation and the grafts removed and processed for histological analysis and flow cytometric quantification of specific cell populations present in the explanted scaffold. During the first couple of weeks, three basically important events happened: (1) inflammatory cells colonized the scaffold, (2) vessels infiltrated the scaffold, (3) donor AFSCs were not retrieved in the scaffold and part of them reached local and distal sites through systemic circulation. Between 1 and 2 months, different types of tissues of mesoderm origin (adipose, muscle, loose and dense connective tissues) were observed inside the scaffolding structure. Importantly, none of these tissues were of human origin meaning that AFSCs did not directly participate in tissue formation.
Finally, cytofluorimetric analysis of cells harvested from the scaffold offered an interesting insight into the host populations recruited at different time intervals. The first 1–2 weeks are particularly significant in order to study the initial phases of the host response. Murine cells positive for CD14 were detected at 1 week post-implantation and were absent at 2 weeks. Since cells extracted from late implants (2 and 4 weeks) were CD14- and CD45+, the authors speculated that CD14+ mature monocytes of the first inflammatory wave of recruited cells might represent the host immune response (residual in nude mice) versus the xeno-transplanted human cells. On the other hand, CD45+ leukoblasts (minimally differentiated) might be, instead, continually recruited from the systemic circulation, and exert still unclear immunoregulatory and remodeling functions on the tissue homeostasis. Cells positive for CD31 (PECAM-1), which is expressed in monocytes and platelets other than in endothelial cells, were retrieved in high amount during the first week and were progressively decreasing from 2 to 4 weeks post-implantation suggesting that an instant blood-mediated inflammatory reaction may occur or that angiogenic and vasculogenic progenitors were acutely mobilized from the bone marrow [57]. The activation of the host endothelium, proved by the detection of CD106 (VCAM-1) positive murine cells at 2 weeks, is important in order to orchestrate localization and diapedesis of circulating blood cells as well [58]. The authors also observed an increasing amount of host CD146 + cells. Being CD146 a component of endothelial junctions [59] and also a marker of pericyte-like cells that surround the endothelial layers and of MSCs [60–62], the identity of these CD146+ cells remains unclear as it is debatable if they originate from the activation of the pericytes associated to the recruited vessels or from the activation of local (subcutaneous in the proposed model) resident stromal cells or from both. Nevertheless, there are many reports indicating that signals from transplanted stem cells, with bone marrow-derived MSCs being the most well characterized, can induce the mobilization of host reparative cells toward the site of a lesion [63–66].
We have also investigated the capability of AFSC-secreted factors to recruit host progenitors in a rat model of ischemic skin [67]. In this study, the subcutaneous tissue from the perfused part of the skin flap outlining a necrotic region was used to quantify the number of murine CD31, CD34, and VEGFR2 positive cells by cytofluorimetry. Only when the skin flap was treated with the AFSC secretome (see the next part of this chapter), were cells positive for CD34 observed in the subcutis. Again, since CD34 cannot be considered solely a marker of EPCs—on the contrary, CD34 positive cells can be isolated also from the adipose stromal-vascular-fraction (SVF) [68]—it is not understood if these cells come from the local subcutaneous niche or from circulation. Nevertheless, a consistent and well-defined population of cells double positive for CD31 and VEGFR2 was recruited by AFSCs secretome. The cytofluorimetric analysis does not prove the endothelial origin of the CD31+/VEGFR2+ cells and, also, does not exclude the hematopoietic origin of the same cells. However, the isolation in culture of cells growing in colonies, with cobblestone morphology, and positive for the vWF, proved that AFSC secretome had recruited and/or activated endothelial progenitors in the subcutaneous niche.
As previously mentioned, Asahara and colleagues [11] were the first to describe EPCs, originally reported as circulating angioblasts for their property to home to ischemic tissues and form de novo vessels, obtained from CD34+ peripheral blood mononuclear cells (PBMCs) by using culture conditions favoring endothelial differentiation. Further studies revealed that the majority of EPCs derive from circulating monocytes which can express endothelial markers, form tubes in Matrigel, be integrated into injured endothelium and accelerate re-endothelialization [69–72]. Monocytes were also found to be progenitors for fibroblasts promoting physiologic scar formation and wound repair or pathologic fibrosis [73]. Originally named fibrocytes by Bucala and colleagues [74] and later described as monocyte-derived mesenchymal progenitors (MOMP) [75], these cells are characterized by a fibroblast-like morphology; expression of CD14, CD45, CD34, and type I collagen; potential to differentiate into osteoblasts, chondrocytes, adipocytes, skeletal myoblasts. Zhao et al. reported that a subset of peripheral blood spindle-shaped CD34+ monocytes even have multipotency features, as for their capability of differentiating along several distinct lineages: mature macrophages, T lymphocytes, endothelial cells, neurons, hepatocytes [76]. Thus, blood circulating monocytes are not only precursors for macrophages and dendritic cells; on the contrary, their plasticity degree, as well as their ontogeny, has not been completely explored.
From the information we have to date, it is plausible that macrophages, and circulating, as well as vascular resident, endothelial progenitors are recruited in the site of AFSC implantation. Because of both the intrinsic difficulty of these recruitment studies and the lack of sufficient data, at present, we are not able to unequivocally identify the cell populations (EPCs, monocyte/macrophages, mesenchymal progenitors, or a single progenitor for all of them) which are mobilized by AFSCs, but, it would be useful to know the mechanism underneath these incredible AFSC- proper regenerative properties.
Also, most studies have focused on the capability of AFSCs to migrate in response to tissue injury. Systemically and locally injected AFSCs were found to distribute to the epineuria and neuromuscular junction of a crushed sciatic nerve [77], stably integrate into skeletal muscles in a model of muscular dystrophy [78], or incorporate into the inflamed bowel wall of a model of necrotizing enterocolitis [79]. Despite the copious availability of data about AFSC response to tissue damage, very little is known about the tissue response to exogenous AFSCs. Therefore, AFSC-mediated recruitment and activation of host progenitors should be further investigated, with the perspective of using these cells as regenerative therapeutic agents, rather than simple substitutes of dysfunctional organs.
5 AFSC Secretome as a Cell-Free Treatment for Ischemic Conditions
The term secretome was originally coined by Tjalsma et al. [80]. The secretome is the ensemble of all the products secreted by a cell, tissue, or organism. An increasing amount of studies, largely conducted on acute myocardial infarction, have revealed that only a very small amount of MSCs engraft and are functionally integrated in the tissue during the post-transplantation period [81, 82]. This has prompted cardiovascular scientists to propose a paracrine effect of MSCs (both trophic and immunomodulatory) as the main mechanism of cardiovascular repair. Many studies have investigated the factors that are secreted by human MSCs from different sources, such as bone marrow, adipose tissue, and ESCs [83–85]. The studies by Mirabella et al. were the first reporting pro-angiogenic properties of the AFSC secretome and the creation of a therapeutic cell-free product named ACM—since it is made from AFSC Conditioned Media, capable of recovering and preventing ischemic damage [67, 86]. ACM is a collection of all the soluble factors secreted by AFSCs and represents the total secretome of these cells at least in certain in vitro culture conditions.
First, in a hind limb ischemia murine model [86], Mirabella and colleagues ligated the right femoral artery immediately distal to the origin of the deep femoral branch. Intramuscular treatment with ACM, or PBS for the control group, was started 10 days after the surgery. It was shown by the authors that ACM prevented atrophy and gangrene of the ischemic limb. ACM-treated mice showed a normal hind limb functionality already 1 week after the first injection and their walking time was comparable to the non-ischemic animals. ACM treatment also stimulated arteriogenesis as evaluated from counting the number of arterioles in the semimembranosus (SM) muscle. The density of endomysial arterioles in ACM-treated mice was significantly higher than in PBS-treated and non-ischemic mice. In the PBS group, the arteriole density was comparable to the non-ischemic group, but the arterioles were enlarged, had thin mural walls and low deposition of collagen fibers in adventitia. On the contrary, in the ACM group, the arteriole showed a regular lumen, a thick tunica media and a remodeled tunica adventitia with hyper-deposition of collagen fibers and fibrocyte infiltration/proliferation. Distal angiogenesis and muscular degeneration, generally occurring in the muscles below the knee, were evaluated on the gastrocnemius (GC) muscle. In the PBS group, distal capillaries and muscle fibers were degenerated. No significant differences in the capillary density and in the distribution of muscle fiber diameter were observed between the ACM group and the non-ischemic group indicating that ACM prevents ischemia-mediated distal tissues damage.
In a second rat model of ischemic fasciocutaneous flap, Mirabella and colleagues [67] elevated a 7 × 7 cm2, abdominally located, full-thick skin flap, and ligated one inferior epigastric neurovascular bundle with the contralateral bundle remaining pervious to perfuse half of the flap (vital part). At the time of the surgery, right before the flap was sutured back, a gelatin membrane, previously embedded with ACM or saline (CTRL), was locally positioned beneath the skin flap. Blood perfusion through the flap was evaluated by laser doppler imaging (LDI) through the week following the surgery. ACM treatment was shown to mediate vessel growth and sprouting from the vital part to the ischemic region of the flap. In the ischemic zone of the ACM-group, the perfusion level at day 7 was 50 % higher than the initial baseline (perfusion before ligation), while the initial perfusion was never recovered in the CTRL group. Since the survival of the flap is linked to the blood supply, the ensuing necrosis development was progressively increasing in the CTRL group, while it was delayed and significantly less pronounced in the ACM group. The histological analysis showed a very thick, dead cutaneous layer in the CTRL group while the ACM group showed normal arrangement of epidermal and dermal structures (glands, follicles, collagen fibers) and a high density of vessels in subcutaneous tissues.
The human AFSC conditioned media was then used by Bollini et al. in an experimental setting of myocardial ischemia (30 min)/reperfusion (2 h) damage [87]. The systemic injection of AFSCs, as well as their conditioned medium, was shown to be cardioprotective. The infarct size and the myocardial cell survival were improved compared to the PBS group and no significant difference between the cellular (AFSC)-group and the relative secretome (ACM)-group was reported.
Other groups have used conditioned media as a surrogate of the secretome from MSCs to achieve repair in vivo.
For instance, human embryonic MSC-derived conditioned medium (CM) was found to reduce progression of chronic kidney disease (CKD) in a rat model: twice daily intravenous injections of CM for four consecutive days after the experimental induction of the CKD increased the glomerular filtration rate and the renal plasma flow in 6 weeks [88].
Concentrated, conditioned medium from cultured (both in normoxia and hypoxia conditions) human bone marrow-derived MSCs was intravenously administered in rats subjected to traumatic brain injury (TBI). Media treated rats performed significantly better than the controls in both motor and cognitive functional test, and the increased neurogenesis accounted for less brain damage [89].
The conditioned medium generated from human amniotic mesenchymal tissue cells (AMTC) was administered to mice with bleomycin-induced lung fibrosis. Two weeks later AMTC-CM-treated mice showed significantly lower fibrosis, as indicated from fibroblast proliferation, collagen deposition, and alveolar obliteration scores [90].
Taken together, both the observations regarding the limited presence/integration of transplanted stem cells in host tissues, and the studies regarding the beneficial effect of the administered conditioned media, strongly suggest that the mechanisms by which stem cells promote the tissue repair are paracrine in their nature.
Is ACM’s capability to attract vessels an ACM prerogative? Of course, the probability that any cell-conditioned medium would provoke an anti-angiogenic reaction, when compared to PBS or saline, is very unlikely. Anyway, it is essential to understand if AFSC pro-angiogenic properties are specific, or at least superior to other cell types.
Mirabella et al. compared the AFSC conditioned medium (ACM) with the medium conditioned by an equal number of Bone Marrow Stromal Cells (BMCM) in a Matrigel plug assay and found that ACM is almost 50 % more angiogenic than BMCM [86]. The same authors compared AFSC- and bone marrow stromal cells (BMSC)-mediated recruitment of host cells after 3 days from implantation and found a significant increment of murine CD31+, CD14+, and VEGFR2+ cells in AFSC constructs compared to BMSC constructs suggesting that AFSCs trigger a strong pro-angiogenic and pro-inflammatory response in the host [53].
In a study by Roubelakis and colleagues, conditioned media (CM) from human BMSCs, dermal fibroblasts (DFs) and AFSCs (isolated by plastic adhesion criteria from fibroblastoid colonies), were analyzed with proteome profiler arrays for angiogenesis growth factors [91]. Although AFSCs and DFs shared about 95 % of the secreted proteins analyzed by the authors, some factors secreted by AFSCs were not detected in CM from DFs, such as EGF and PDGF-AB/BB. More interestingly, the BMSC-CM lacked many of the inflammatory response molecules found in AFSC-CM and DF-CM (IL-8, MCP-1, MIP-1α, GM-CSF, IL-1β), molecules involved in vessel stabilization (angiostatin, endostatin, thrombospondin 2, TGFβ-1, PDGF-AB/BB), enzymes involved in tissue remodeling (MMP-8, MMP-9), growth factors important for tissue repair (EGF, HGF, FGF4), and peculiar pro-angiogenic factors (EGVEGF, PD-ECGF). Although the technique adopted by the authors is semi-quantitative and not very sensitive for detecting low abundant proteins, it offers an important panoramic view on the secretome of different cell types.
Previously, Mirabella et al. used Luminex’s xMAP Technology to detect the amount (pg/ml) of some AFSC-secreted factors, normalized by the DNA content of the secreting cells [86]. The authors found that 1 μg of DNA (meaning about 170,000 AFSCs) secreted about 1 ng of VEGF-A, IL-8 and SDF-1α, about 0.5 ng of IL-6 and MCP-1, about 0.2 ng of TGFβ-1. The same authors also demonstrated an up-regulation of the cited molecules after TNF-α stimulation of AFSCs [53].
Between the soluble factors detected at high level in the ACM [86], VEGF-A, one of the main factors that prime endothelium development, would be considered a possible candidate causative of the in vivo pro-angiogenic reaction. But CM from BMSCs releases almost double the amount of VEGF-A compared to ACM [91]. Thus, the factors released by AFSCs must act in combination to achieve the strong pro-angiogenic response so far reported. It is known that SDF-1α is chemoattractive for EPCs [92]. It could be conceivable that VEGF-A and SDF-1α act in synergy to promote angiogenesis, as this has been proven in tumors [93]. Another powerful chemokine secreted by AFSCs at higher levels than BMSCs is IL-8 [86, 91], which directly enhances endothelial cell survival and matrix metalloproteinases production which is important for capillary sprouting [94, 95].
In general, angiogenesis is a complex biological process:
It is driven by many factors and each of them can be necessary but not sufficient to the process.
The removal of one factor can result in no phenotype changes because of biological redundancy.
The over-supply of a non-necessary factor can result in pronounced phenotypic changes.
Indeed, angiogenic growth factors (GFs) have been used for therapeutic angiogenesis. Few studies have compared and combined different GFs to identify which could be critical, since in most of the cases a single factor is not sufficient to promote angiogenesis and two or more GFs can act in synergy [3, 96–99].
AFSC-derived secretome, thus ACM, has been proposed as a cell-free cocktail of different growth factors and chemokines capable of stimulating the migration and proliferation of vessel progenitors as well as maturation of neo-formed vessels. In vitro studies have demonstrated that when HUVEC were cultured for 16 h with ACM, the expression level of CD105 (endoglin) was doubled and the level of CD146 significantly upregulated as well [86]. The glycoprotein CD105, a part of the TGFβ-receptor complex, is required for the development of the cardiovascular system and the neo-formation of blood vessels [100]. It also antagonizes the inhibitory effects of TGFβ-1 on endothelial cell growth [101]. The glycoprotein CD146 is an adhesion marker of endothelial cells, which has also been identified on other cell types, such as pericytes and MSCs, and it is involved in the control of cell–cell cohesion and vessel integrity [59]. Taken together, these results suggest that ACM can promote both vessel growth and stabilization. Unfortunately, all the studies published to date are descriptive and mechanisms by which AFSCs stimulate angiogenesis have not been elucidated. However, it is clear that AFSC secretome does contain the paracrine signals needed to stimulate therapeutic angiogenesis, and a successful use of ACM in clinical protocols for therapeutic angiogenesis would shift the current tissue engineering (TE) paradigm of implanting exogenous bioengineered vessels to a newer regenerative medicine (RM) breakthrough of inducing endogenous vessel growth (Fig. 12.2).


Fig. 12.2
Proposed model to switch from a tissue engineering (TE)—to a regenerative medicine (RM)-based therapeutic angiogenesis. In the TE approach, AFSCs, as other stem cells expressing mesenchymal markers (CD44, CD29, CD90, CD105, CD73, etc.) in culture, have to be differentiated or reprogrammed to express endothelial cell markers (CD31, vWF, eNOS, VE-Cadh, VEGFR2); subsequently, the engineered cells or vessels will be transplanted in the patient. In the RM approach, the release of angiogenic factors from AFSCs, as well as the simple use of ACM, is sufficient to drive the vascularization of ischemic tissues in the patient
6 Remarks and Future Challenges
There is stronger and stronger evidence that the stem cell-based therapy could serve as a “chemotactic” recruitment of host progenitor cells. To engineer a tissue in vitro is a very difficult operation both theoretically and practically. Since we do not possess the complete knowledge of developmental biology for each cell type, it is at least compelling to recreate the same identical conditions of a developing organism through the large availability of grow factors, scaffolds, and bioreactors. The in vivo cell therapy approach may consist of systemic infusions or topic implantations of cells. In both cases, after the eventual migration toward the injured site, cells should be retained in the compromised tissue, at least for a certain time, in order to establish a bidirectional, communicating network with the host microenvironment which consists in:
Receiving stimuli from healthy and damaged resident cells and from inflammatory infiltrating cells.
Giving a feedback by juxtacrine (contact-dependent) interactions with cells and by the release of cytokines and grow factors that exert a short distance (paracrine) activation of the tissue niche and/or a long distance (“endocrine”) recruitment of circulating progenitors or progenitors settled in anatomically separated sites.
The focus of this chapter was to discuss the emerging evidence that:
1.
AFSCs need to be rationally reprogrammed toward the endothelial lineage in order to be functional vessels in vivo. We need to learn more about the biology of blood vessel development to optimize differentiation protocols.
2.
The crosstalk between AFSCs and specific tissue niches has to be elucidated each time a new application of these cells results in a beneficial effect. Mechanisms of repair can be mediated by the recruitment of host reparative progenitors, rather than by a direct integration of AFSCs in the tissue.
3.
The administration of soluble factors secreted by cultured AFSCs may achieve regenerative effects equivalent to cell therapy. The research and development of secretome-based therapy would be free from the limitations of immune rejection and possible tumorigenesis observed in cell transplantation.
The future challenges that the field will encounter, can be summed up as follows:
Which amniotic fluid-derived cell fraction has to be used? Also, is the maintenance of the stem potential essential in order to get a therapeutic effect? Amniotic fluid contains heterogeneous cell types. To date, c-kit-positively selected amniotic fluid cells are considered multipotent stem cells [18]. On the other hand, c-kit negative amniotic cells are considered lineage-committed, phenotypically marked as CD324, CD326, and CD24 positive cells [30]. Finally, Arnhold et al. showed that magnetic c-kit selection does not influence the growth in culture but differentiation capacity through different lineages is affected [102].
What is AFSC fate in vivo? As discussed above, many studies have reported that AFSCs are poorly or not retrieved at the site of implantation. It is known that AFSCs acutely recruit a wave of inflammatory cells, and that they have migratory properties through the circulatory system [51, 53, 56]. Both these processes can account for in vivo AFSC clearance.
Which strategies must be envisioned to force AFSCs to be retained in vivo? Protocols of differentiation and reprogramming, or selection of tissue-committed subpopulations could improve both the engraftment and the functionality of transplanted AFSCs.
AFSC secretome and its most immediately translational surrogate, the ACM, have to be better characterized. Liquid Chromatography with Tandem Mass Spectrometry Detection (LC-MS/MS) can be useful for characterizing the secretome profile, although not very sensitive as to detect low abundant proteins. Antibody-based methods (e.g., ELISA and antibody arrays) are limited by the availability of antibodies to detect secreted proteins. A systematic integrated approach would include LC-MS/MS detection, antibody arrays, microarrays, and bioinformatics [85, 103].
In order to overcome short protein half-lives and rapid diffusion, the delivery of AFSC secretome could be achieved as a sustained and controlled release of ACM factors in a temporal and spatial-controlled manner, or in an environment-responsive manner.
When I search PubMed for “amniotic fluid stem cells,” I get less than 600 hits! We are on a good track, but we need to combine criticism and enthusiasm for a more in depth examination of AFSCs. We have in our hands a cell source with a great potential for many still unexplored future applications.
References
1.
Folkman J. Tumor angiogenesis: therapeutic implications. N Engl J Med. 1971;285(21):1182–6.PubMed
2.
Senger DR, Galli SJ, Dvorak AM, Perruzzi CA, Harvey VS, Dvorak HF. Tumor cells secrete a vascular permeability factor that promotes accumulation of ascites fluid. Science. 1983;219(4587): 983–5.PubMed
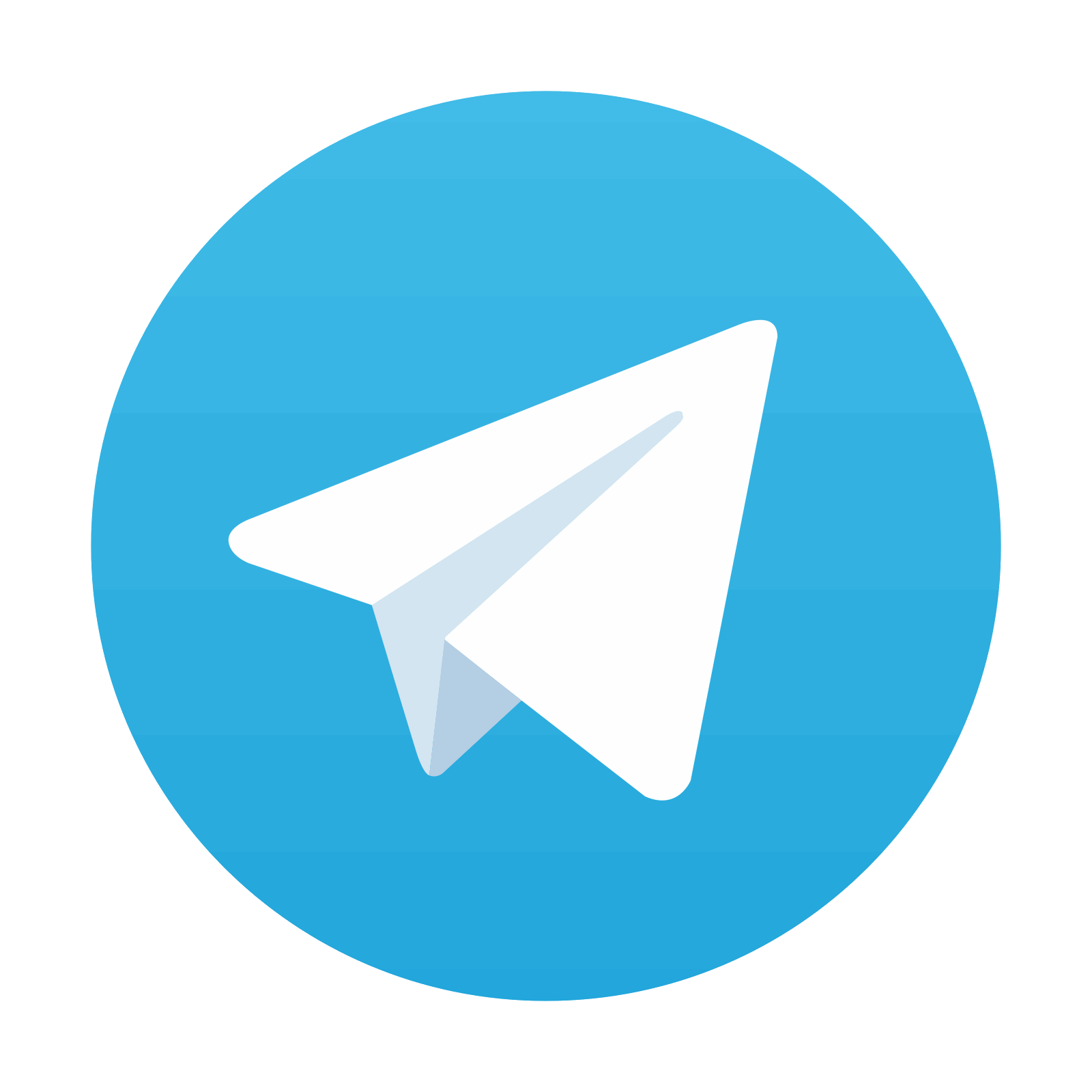
Stay updated, free articles. Join our Telegram channel

Full access? Get Clinical Tree
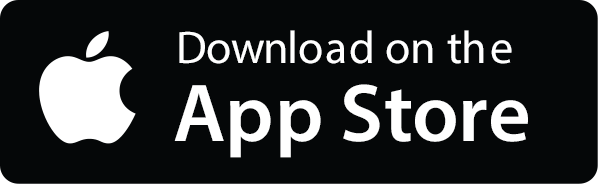
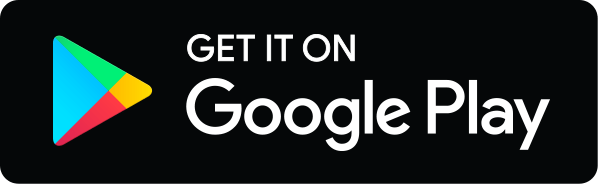