(30.1)
or after integration

(30.2)

(30.3)
When the logarithm of the number of surviving organisms is plotted against sterilisation time in a semi-logarithmic graphic, a straight line is obtained (see Fig. 30.1).
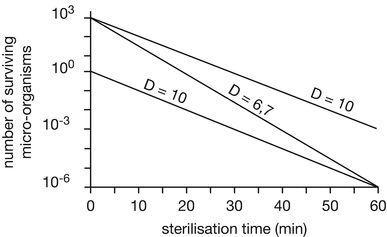
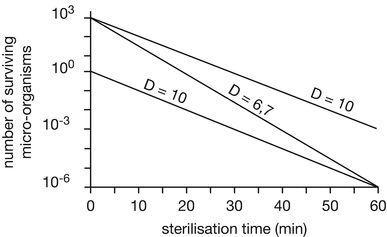
Fig. 30.1
Elimination curves of two types of micro-organisms with different D-values resp. micro-organisms with the same D-value but different initial contamination level at 100 °C heating temperature. Source: Recepteerkunde 2009, ©KNMP
When the number of surviving micro-organisms is plotted directly (not logarithmic) against time, a curve is obtained which approaches the X-axis asymptotically with time. This means that theoretically the zero value is never reached. In time, the chance of a residual contamination with micro-organisms becomes smaller and smaller. Therefore, sterility, if defined as the absolute absence of micro-organisms, can never be guaranteed nor proved. The description sterility assurance level (SAL) is used instead [1]. It is the probability of a single unit being non-sterile after it has been subjected to sterilisation. It is important to note that when stating “1 container in 106 may not be sterile” this does not mean that it is acceptable for 1 container to be unsterile.
SAL also describes the killing efficacy of a sterilisation process. A very effective sterilisation process has a very low SAL. A SAL of 10−6 corresponds to the probability of not more than one micro-organism present in 106 (one million) sterilised units of the product.
As for pharmacokinetics and reaction kinetics, rate parameters can be defined for sterilisation processes, such as the first-order reaction rate constant (k) with the dimension reciprocal time and the half-life (t1/2). However, more commonly the term decimal reduction time or D-value is used. The D-value is the time required to inactivate 90 % of the present micro-organisms.
The D-value, half-life, and k are related as follows:

The D-value is thus, like k and t1/2, a measure for the resistance of the micro-organism to the sterilisation process that is applied. D is influenced by environmental factors and the state the micro-organism is in, especially the hydration state.

(30.4)
The D-value is always defined for a certain temperature and for a defined process. Table 30.1 presents a number of D-values for micro-organisms. Spores of Bacillus and Clostridium strains have the highest heat resistance.
Micro-organism | Medium | T (°C) | D-value (min) |
---|---|---|---|
Geobacillus stearothermophilus a | Water | 121 | 2 |
Bacillus subtilis a | Air | 160 | 5–10 |
Bacillus subtilis a | Water | 100 | 11.3 |
Clostridium sporogenes a | Water | 121 | 0.8–1.4 |
Clostridium botulinum a | Water | 121 | 0.2 |
Non-spore-forming bacteria | Water | 65.5 | 0.5–1 |
Micro-organisms are killed faster at high temperature than at low temperature. In order to quantify the change in resistance of a micro-organism in response to a change in temperature, the Z-value has been introduced. The Z-value is the number of degrees of temperature increase required to decrease the D-value by a factor 10.

In practice, the Z-value of a bacterial strain is calculated from the D-value at different temperatures (see Fig. 30.2). These D values are not absolute but depend on the method of preparation of the spores. For precise calculations the D value must be obtained for each batch made from the supplier. This is particularly important where Geobacillus stearothermophilus is used as biological indicator.
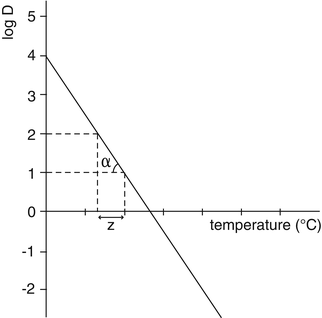

(30.5)
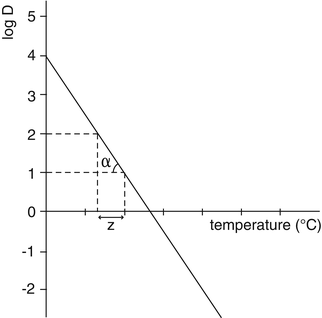
Fig. 30.2
Calculation of the Z-value from the relationship between the decimal reduction time D and the temperature. Source: Recepteerkunde 2009, ©KNMP
30.3 Sterilisation Time
The sterilisation time that is required to obtain sterility at a certain temperature is dependent on the number and the resistance of the micro-organisms present in or on the product. An initial contamination with 106 of a certain micro-organism requires twelve decimal reduction times to obtain the SAL of 10−6, see equation 30.3.
In theory two sterilisation processes can be distinguished: the overkill process and the bioburden process.
The overkill process is often used in daily practice and is based on the principle that the process should be sufficiently powerful to reduce a severe contamination with a very thermo-resistant organism, i.e. circa 106 spores of Geobacillus stearothermophilus, to the SAL 10−6. The standard process for steam or hot water sterilisation in the Ph.Eur. is 121 oC for 15 min and complies with this requirement. The overkill method is used when the product can withstand excessive heat treatment such as an Fo ≥ 12 without adverse effects. Bioburden and resistance data are not required to determine the required ‘Fo’ values.
The bioburden process is based on the initial contamination before sterilisation, and thus it is a tailored sterilisation process. Bioburden based sterilisation cycles are not commonly used in Europe. Prerequisite is that the number and thermo-resistance of the micro-organisms in the product are documented, based on which the customised sterilisation time can be calculated. An example can be found for parenteral solutions (for injection, for infusion) in reference [4]. In this example, N0 is 1 (rounded up) and the D-value at 100 °C of the most resistant micro-organism is approximately 2 min. Using equation 30.3, it can be calculated that a SAL of 10−6 will be obtained after 12 min.
There are basically two possibilities for determination of the sterilisation time of the overkill procedure:
The autoclave computer software waits for the temperature in the Pt-100 in the product on the coldest spot having reached the set maximum process temperature (for example 121 oC) and keeps the load at this temperature for a specified duration (15 min); after that the cooling phase starts.
The autoclave computer software takes into account the elimination of micro-organisms during the heating and cooling phases and adds the time that the product is kept at the maximum process temperature. The total lethality (the elimination effect) is not only obtained during the sterilisation phase, but during the heating and cooling phase germs die as well. The phase of maximum process temperature can be shorter in that case (but shortening of the sterilisation time at maximum temperature is not used when not necessary). The contribution of these three phases and subsequently the total lethality of the sterilisation process are dependent on the Z-value of the micro-organism(s) concerned. Autoclaves are equipped with computer software that can easily calculate these parameters.
For both the calculation of the lethality during the heating and cooling phase and the comparison of sterilisation processes at various temperatures, the F-value has been introduced. The F-value of a sterilisation process at a certain specified temperature (not the reference temperature) is the number of minutes that a sterilisation process would need to last at a reference temperature (usually 121 °C) to obtain the same degree of elimination. This is expressed by the equation:

In which
is the time at temperature T1, and
is the time with the same lethality at the reference temperature TR and Z is the Z-value.

(30.6)


For example, if after the calculation of the contribution of heating and cooling a sterilisation is performed that corresponds to 15 min sterilisation at 121 °C, the F-value is 15 (F121 = 15).
The Z-value is a measure for the change in resistance of the micro-organism following the change in temperature. Therefore, the Z-value should always be mentioned in the notation of the F-value. For steam and hot-water sterilisation, usually a Z-value of 10 °C is used.
F121°C 10°C = 15 thus means an F-value of 15 min at 121 °C calculated with a Z-value of 10 °C.
F121°C 10 °C can also be simplified to F0. In general, the F0-value of a sterilisation process stands for the lethality of that process expressed as the time at 121 °C calculated with a Z-value of 10.
For hot air sterilisation, the influence of the temperature on the elimination of the micro-organisms is characterised by the Z-value as well. Moreover, the effectiveness of two hot-air processes can also be compared with the F-value.
30.4 Initial Contamination
The initial contamination, also referred to as pre-sterilisation bioburden, is the contamination of a starting material or product just before sterilisation or before a disinfection procedure is performed. The initial contamination depends on the degree of contamination of the starting materials (including water), the packaging material, the storage conditions, the storage time, the preparation processes, and the circumstances during production (hygiene). When filtration is not performed as a final sterilisation process but as part of the preparation (removal of particles and lowering of the bioburden by membrane filtration), the initial contamination is determined after filtration and measured in the filled container, ready for sterilisation.
Bacteria may negatively influence product quality even after thermal destruction, for example when pyrogens are formed from the destruction of bacteria (see Sect. 19.3.4). Therefore, it is essential to reduce the initial contamination as much as possible before sterilisation. This can be done by choosing starting materials with a low level of contamination, such as containers with sterilised water for injection, hot or freshly cooled water for injections in bulk or sterilised base solutions for the preparation of eye drops. Furthermore, the equipment and primary packaging should be clean, sterile, and pyrogen free and direct contact should be avoided between the product, critical unsterile spots on the primary packaging and the hands of the operator. To reduce the growth of micro-organisms and the development of pyrogens in the product that is to be sterilised, the time between filling the primary packaging and sterilisation should be kept short, usually maximal four hours. However this time period may be somewhat longer in special cases, according to a risk assessment.
Final filtration through a sterile 0.2 μm membrane filter is not only a sterilisation method, but also an effective way to reduce the initial level of contamination. A pre-filtration (often in-line) with a coarser pre-filter (for example 1.2 μm) is necessary to reduce coarser foreign particles and contamination and to prevent early clogging of the 0.2 μm membrane filter.
Because products that require sterilisation are often aqueous solutions, the initial microbiological contamination can easily be determined in the quality control laboratory by the membrane filtration method (see Sect. 19.6.3).
30.5 Terminal Sterilisation Methods
The sterilisation methods (methods of preparation of sterile products) described in the Ph. Eur. are terminal sterilisation methods and filtration [1]. See for filtration Sect. 30.6. In this section an overview of terminal sterilisation methods is given.
Terminal sterilisation methods are:
Steam sterilisation (heating in an autoclave): steam sterilisation or sterilisation with hot water in a closed container, minimum 15 min at 121 °C (F0 = 15). The humid heat denatures proteins and DNA of micro-organisms.
Dry heat sterilisation: hot air sterilisation at least 2 h at a minimum of 160 °C. Dry heat at temperatures higher than 220 °C is used for sterilisation and depyrogenisation of glassware. Sterilisation by dry heat is mainly caused by oxidation of micro-organisms.
Ionising radiation sterilisation: an absorbed radiation dose of at least 25 kGy. This method leads to breaks in the DNA of micro-organisms and, in presence of water, the formation of free radicals.
Gas sterilisation including gas plasma sterilisation: Gas, penetrated with moisture, enters the material to be sterilised, which is followed by the elimination of the gas. The gas (ethylene oxide or hydrogen peroxide) alkylates the purine and pyrimidine bases in the RNA and DNA of micro-organisms.
All mentioned sterilisation methods allow for different conditions, as long as the procedures and precautions are chosen as such that a SAL of 10−6 is obtained [1]. However broad sterilisation experience, good knowledge of GMP and process validation are necessary to work with different conditions. In many pharmacies and industries the standard methods are used in practice.
30.5.1 Steam (and Hot Water) Sterilisation
The principle of steam sterilisation for medical devices, pharmaceutical products and utensils is based on heat transfer by hot condensing steam under pressure. The steam condenses in the autoclave to pure water, releasing at that moment its heat content. This is a very effective means of heat transfer. Furthermore, the mechanism of inactivation by saturated steam (denaturation of proteins) is also very effective. Therefore, steam sterilisation in an autoclave is the preferred method for medical devices, utensils and some pharmaceutical products. It is of critical importance that the steam in a steam autoclave is completely saturated and not superheated, because only then the sterilisation is effective. For the details of steam sterilisation reference is made to other textbooks and guidance, such as [4, 5]. The pressure of saturated steam at different temperatures is shown in Table 30.2.
Table 30.2
Temperature-pressure relation of saturated steam
Temperature (°C) | Pressure (kPa) |
---|---|
100 | 101 |
115 | 170 |
121 | 204 |
134 | 304 |
It is important that either the steam can penetrate into the object to be sterilised (medical devices or utensils in dedicated steam sterilisation packaging), or that the object in a closed container contains water (content of an ampoule, bottle, vial or plastic bag with aqueous solution).
Also ‘empty’ sterile bottles must contain some water for injections (and be closed under vacuum) before they can be sterilised by steam or hot water. Without water inside only radiation or heat sterilisation is effective to sterilise an empty, washed and dried container.
30.5.1.1 Steam Autoclave or Steam Steriliser
The steam steriliser is commonly referred to as an autoclave. The simplest steam steriliser, however, is a pressure cooker. In a pressure cooker, the added distilled or deionised water is heated and eventually begins to boil. The steam that is formed pushes the air out through the vent valve in the lid. This is not a very effective process because the vent valve is in the upper part of the steriliser and it takes time to remove the air just by continuous boiling. Air is heavier than steam, so these gases are slowly removed by upwards displacement to the upper part of the pressure cooker.
In a steam autoclave air is more effectively removed by downward displacement which makes loading with more and differently shaped objects possible as well. After removal of the air, the valve is closed and the steam pressure gradually increases. A pressure control valve is used to set the desired pressure, and thereby the desired temperature, since these parameters have a fixed relation in the absence of air (see Table 30.2). For control of the temperature and duration of the sterilisation process a timer or Programmable Logic Controller (PLC) can be used. A PLC is part of the computer of the autoclave. In a PLC the routine programs are factory set and fixed, so for the process operator it is not possible to change them. The temperature-time course inside the steam steriliser is shown in Fig. 30.3.
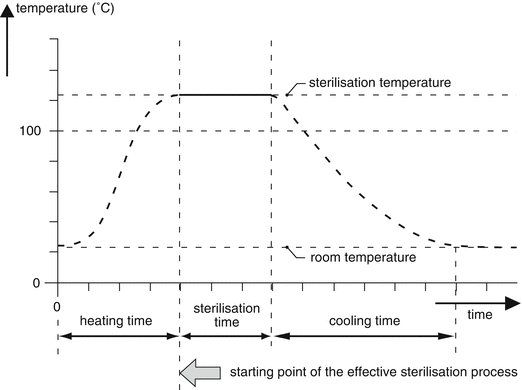
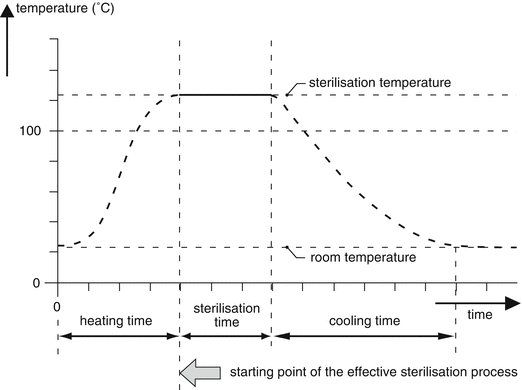
Fig. 30.3
Temperature-time course (temperature profile) during a sterilisation process in an autoclave (Reprinted with permission of the publisher [9])
Manual control of a pressure cooker is not realistic and GMP compliant in practice because it does not result in a reproducible process. Moreover incidental manual control by an operator is only allowed with the authorisation of a competent person. GMP compliant autoclaves are entirely computer-controlled using multiple pressure and temperature sensors. Processes for control and operation administration are validated.
30.5.1.2 Process Description of Steam Sterilisation for Medical Devices
In contrast to the sterilisation of liquids in closed containers, medical devices, such as surgical instruments, require the sterilising steam to penetrate through the packaging material to reach the surface of the device. The steam condenses on the cold surface, thereby transferring heat to the medical device, which results in heating up to the sterilisation temperature. The result is that both the medical device and the packaging become wet. Since wet packaging is not a barrier to micro-organisms, the packaging should be dried during and at the end of the sterilisation process. Moreover, the sterilisation agent (steam) should be dry and saturated, which means that the steam should not contain any air.
30.5.1.3 Packaging Medical Devices
The packaging of medical devices after cleaning and before sterilisation should protect the content (against contamination) as well as (during sterilisation) allow for removal of air and penetration of steam. The classical and most often used packaging material is sterlisation paper. Instrumentation kits are wrapped in sheets of sterilisation paper or non-woven materials that are subsequently sealed with sterilisation tape, which usually has a sterilisation indicator.
Smaller instruments are packed in sterilisation bags, which are made of sterilisation paper and transparent plastic. Sterilisation bags are sealed with equipment designed for the purpose.
The sealed packaging has a defined shelf life during which it should protect the sterilised content against contamination [5]. The user should be able to open the packaging aseptically.
30.5.1.4 Process Description of Steam Sterilisation of Aqueous Pharmaceutical Products
The steam sterilisation process is pressure-controlled and temperature-monitored. The most important steps in the steam sterilisation process are (see also Fig. 30.4):
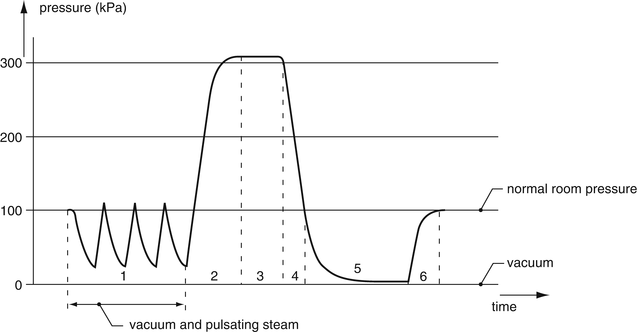
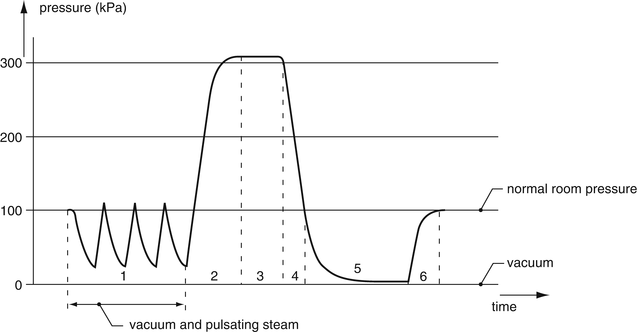
Fig. 30.4
Sterilisation process for medical devices (Reprinted with permission of the publisher [9])
1.
Removal of air by repeated pre-vacuum
2.
Heating/building up pressure by steam inlet
3.
Sterilisation in dry saturated steam
4.
Removal of the steam
5.
Drying by post-vacuum
6.
Letting in air until atmospheric pressure
During the steam sterilisation of aqueous liquids in closed containers (infusion bottle, bag, injection vial, ampoule, etc.), saturated steam has to heat the container and product inside the container. Steam condenses first on the colder surface of the container, which is the method by which heat is transferred to the aqueous content. In this way, every water containing container becomes a unique steriliser itself.
Sterilisation of aqueous liquids in closed containers by the traditional steam sterilisation process has some advantages and some important disadvantages:
The advantage of steam is that closed glass ampoules, glass injection vials and glass infusion bottles can be sterilised effectively. Filled and closed ampoules for injection are sterilised upside down in perforated stainless steel cassettes in streaming saturated steam. After sterilisation the steam autoclave is brought to vacuum. The vacuum dries the outside of the ampoules. This is used as leak test as well, because leaking glass ampoules will lose their content in the vacuum phase. The result is an empty container, which will be detected in the visual quality control process after sterilisation.
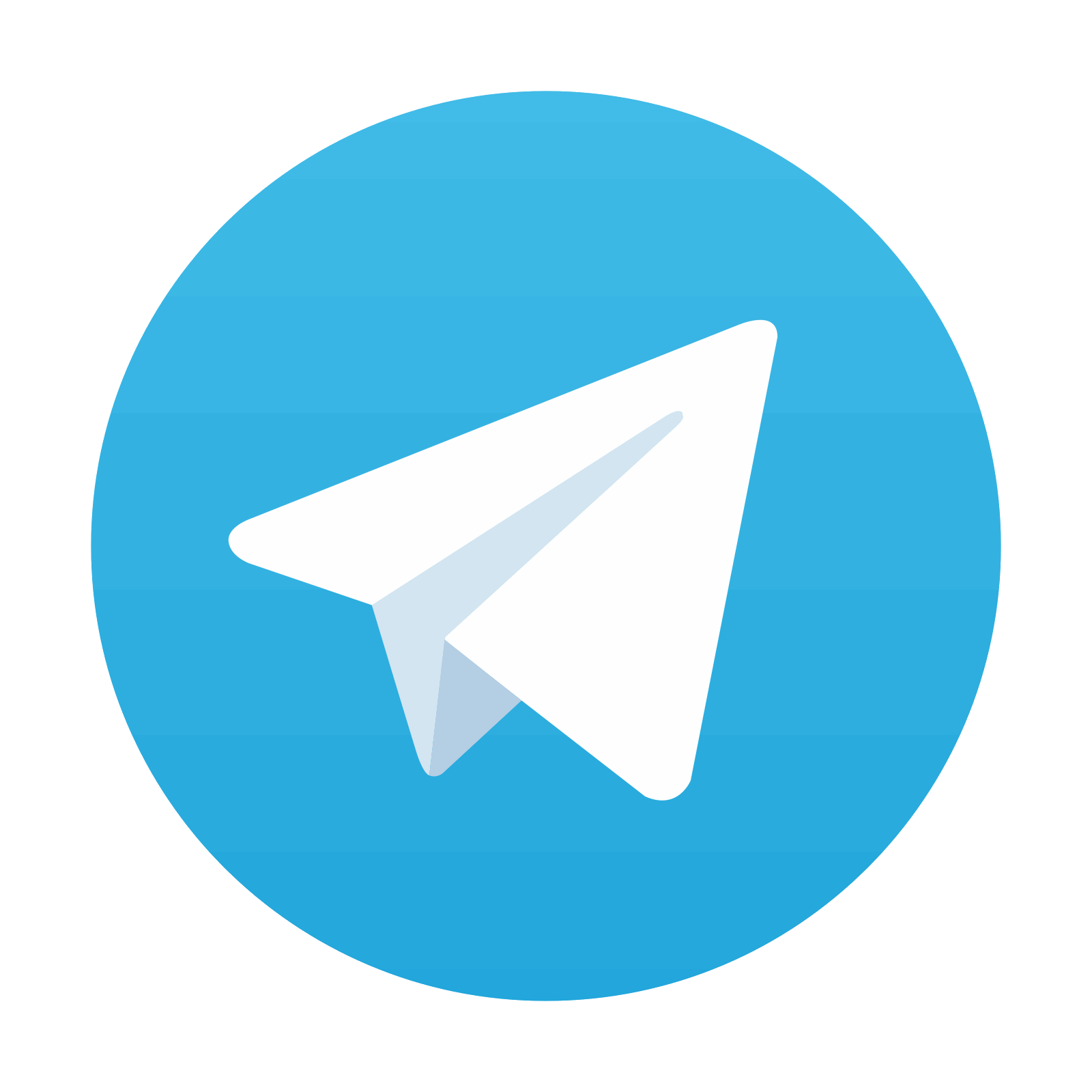
Stay updated, free articles. Join our Telegram channel

Full access? Get Clinical Tree
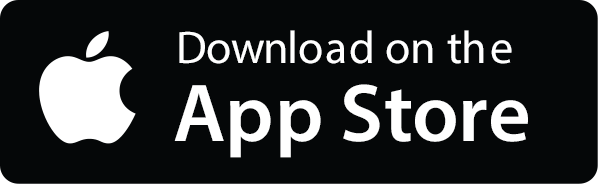
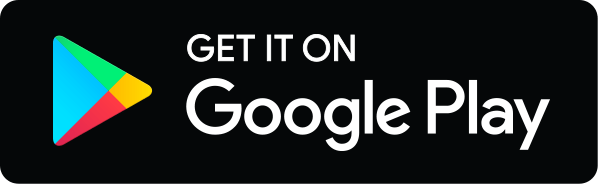