1 Rebecca M. Perrett The development of one’s sex comprises ‘sex determination’ – the development of the undifferentiated gonad into testis or ovaries during embryogenesis, followed by ‘sex differentiation’ – the determination of phenotypic sex induced by factors produced by the differentiated gonad. This chapter will highlight the molecular mechanisms underpinning these two processes. During the first 2 weeks of human embryonic development, the only difference between XX and XY embryos is their karyotype. At the two‐cell stage of the XX zygote, X chromosome inactivation occurs, enabling males and females to have equal transcript levels from the X chromosome (Huynh and Lee 2001). In developing germ cells, the X is reactivated in the female, so both X chromosomes contribute to oogenesis (Sugimoto and Abe 2007). During the fourth week of human development, the urogenital ridges develop as a thickening of the mesodermic mesonephros covered by coelomic epithelium (CE); it is from this structure that the urogenital system and adrenal cortex originate. In the fifth week, or mouse embryonic day (E) 9.5–10.5, the urogenital ridge divides into a urinary and adreno‐gonadal ridge the latter of which forms the gonads and adrenal gland (Swain and Lovell‐Badge 1999). Until the sixth week of human development, or mouse E11.5, the undifferentiated gonads of XX and XY individuals are identical and have the potential to form either ovary or testes (bipotential). A number of factors have been shown to be required for the development of the undifferentiated gonad, as illustrated in Figure 1.1. However, due to the limited studies in human development, mouse studies have revealed several more important factors involved in gonadal development, and these are outlined below. Figure 1.1 Simplistic illustration of the molecular determinants for gonadal differentiation. In the presence of SRY, SOX9 is upregulated and is responsible for the regulation for testicular development. In the absence of SRY, pro‐ovarian factors regulate ovarian development (see text for more detail). Emx2 encodes a homeodomain transcription factor expressed in urogenital epithelial cells. Knockout mice completely lack kidneys, gonads, ureters and genital tracts, but the adrenal glands and bladder are normal (Miyamoto et al. 1997), indicating Emx2 acts after division of the urogenital ridge. It may regulate tight junction assembly, allowing migration of the gonadal epithelia to the mesenchyme (Kusaka et al. 2010). Pax2 is a transcriptional regulator expressed within the urogenital system during development, in both ductal and mesenchymal components (Torres et al. 1995). Null mice lack kidneys, ureters, and genital tracts, and the Wolffian and Müllerian tracts degenerate. The POU domain containing Tcf2 gene functions in epithelial differentiation (Coffinier et al. 1999; Kolatsi‐Joannou et al. 2001). It is essential for urogenital development, as patients harbouring mutations exhibit genital malformations (Lindner et al. 1999; Bingham et al. 2002). The transcription factor Sf1 is expressed in the hypothalamus, pituitary, gonads, and adrenal glands (Luo et al. 1994; Val et al. 2003). Null mice lack gonads and adrenal glands (Luo et al. 1994; Shinoda et al. 1995). Sf1 also functions later in testis development. Wt1 encodes multiple isoforms of a zinc finger protein, which act as transcriptional repressors (Menke et al. 1998) or activators (Lee et al. 1999). The –KTS variant promotes cell survival and proliferation in the indifferent gonad, whereas the +KTS isoform functions in testes differentiation (Hammes et al. 2001). The –KTS isoform activates the sex‐determining region Y (Sry) and Sf1 promoters (Hossain and Saunders 2001; Wilhelm and Englert 2002). Wt1 is expressed in urogenital ridges (Pritchard‐Jones et al. 1990) where it maintains the identity of adreno‐gonadal primordium (AGP) the precursor to the gonads and adrenal primordia (Bandiera et al. 2013). Accordingly, null mice lack kidneys and gonads (Kreidberg et al. 1993). Knockout of Lhx9, a homeobox protein, causes failure of gonadal development (Birk et al. 2000) and synergizes with Wt1 to regulate Sf1 expression (Birk et al. 2000; Wilhelm and Englert 2002). Cbx2 is the mouse homologue of the Drosophila polycomb gene and regulates transcription by altering chromatin structure. Knockout XX mice have small or absent ovaries and XY mice show male–female sex reversal (Katoh‐Fukui et al. 1998). Cbx2 may regulate Sf1 expression in the gonad, as it does in the adrenal gland (Katoh‐Fukui et al. 2005), or it may alter Sry expression directly (Katoh‐Fukui et al. 2012). Cited2 is a transcriptional regulator expressed in the AGP, and later in the CE and underlying mesenchyme of the genital ridge (Bhattacharya et al. 1999; Braganca et al. 2003). It cooperates with Wt1 to stimulate Sf1 expression in the AGP (Val et al. 2007; Buaas et al. 2009), and also ensures Sry levels are sufficient to trigger testis determination. Gata4 is a transcription factor first detected at E11.5 in somatic cells of XX and XY gonads; at E13.5 it is upregulated in XY Sertoli cells and downregulated in interstitial cells and XX gonads (Viger et al. 1998). It is required for gonadal ridge formation (Hu et al. 2013), along with later functions in testicular and ovarian development. Primordial germ cells (PGCs), the founder cells of the germ cell lineage, are typically established early during embryonic development. Germ cell specification can either occur through the inheritance of germ cell determinants already present in the egg (preformation), as in Drosophila melanogaster and Caenorhabditis elegans, or in response to inductive signals, as for mice and probably all mammals (epigenesis) (Extavour and Akam 2003; Saitou and Yamaji 2012). Mouse PGCs (mPGCs) originate in the pluripotent proximal epiblast at about E6.0 when they respond to signals from extraembryonic tissues and express Fragilis/Interferon‐induced transmembrane protein 3 (Ifitm3) (Saitou et al. 2002). Bone morphogenetic protein 4 (Bmp4) and 8b from the extraembryonic ectoderm and Bmp2 and wingless‐type MMTV integration site family, member 3 (Wnt3) from the visceral endoderm are critical for specification (Lawson et al. 1999; Ying et al. 2000; Ying and Zhao, 2001; Ohinata et al. 2009). At E6.25, about six of these cells express B‐lymphocyte‐induced maturation protein 1 (Blimp1, also known as PR domain‐containing 1, Prdm1): these cells are PGC precursors (Ohinata et al. 2005), although further cells are recruited to become PGCs before E7.25 (Saitou et al. 2002; McLaren and Lawson 2005; Ohinata et al. 2005). Wnt3 acts via β‐catenin to activate the mesodermal factor T (brachyury), which in turn induces Blimp1 and Prdm14 expression (Aramaki et al. 2013); these are transcriptional repressors which suppress the somatic program while allowing establishment of germ cell character (Saitou et al. 2002; Saitou et al. 2005; Ohinata et al. 2005; Vincent et al. 2005; Yabuta et al. 2006; Seki et al. 2007; Kurimoto et al. 2008; Yamaji et al. 2008). The expression of genes which establish/maintain pluripotency are retained via the epiblast, including Sox2, Nanog, Oct4, and Embryonal stem cell gene 1 (Esg1) (Scholer et al. 1990; Ohinata et al. 2005; Western et al. 2005; Yamaguchi et al. 2005; Yabuta et al. 2006; Chambers et al. 2007). Following establishment of the germ cell lineage, extensive reprogramming of the genome occurs, i.e. erasure of epigenetic marks such as DNA methylation and establishment of new marks (Surani 2001; Hajkova et al. 2002). Imprinting must be reprogrammed in the germ line, as a maternal allele in one generation may be a paternal allele in the next. PGCs do initially acquire genome wide de novo methylation; however, following entry into the gonadal ridge, there is rapid demethylation, simultaneously in male and females, prior to their sex‐specific differentiation. The timing of erasure in humans is not known, but in mice it begins between E10.5 and E11.5, i.e. after arrival in the gonadal ridge (Lee et al. 2002). Remethylation occurs in XY germ cells once they have committed to the spermatogenic fate, and in XX germ cells just before ovulation (Hajkova 2011). Human PGCs (hPGCs) are first identified in the wall of the yolk sac at 23–26 days postfertilization (Witschi 1946). The process of hPGC specification is thought to be similar to that in mPGCs, given the conserved expression of key regulatory genes, including that of OCT4, NANOG, BLIMP1, TFAP2C and cKIT (Anderson et al. 2007; Eckert et al. 2008; Kerr et al. 2008a; Kerr et al. 2008b). Human PGCs also undergo extensive epigenetic reprogramming (Gkountela et al. 2013). However, in contrast to mPGCs, hPGCs do not express the key pluripotency transcription factor SOX2 (Perrett et al. 2008), hinting towards fundamental differences between human and mouse PGC specification. At approximately E10.5 in the mouse and between weeks 5 and 8 of human gestation, PGCs actively migrate from the allantois through the gut mesentery to the genital ridges of the developing gonad (Figure 1.2), exhibiting polarized morphology and extending cytoplasmic protrusions (Fujimoto et al. 1977; Anderson et al. 2000; Molyneaux et al. 2001). Again, studies in the mouse have revealed the involvement of a number of key molecules, which are also implicated hPGC migration. Thus, the c‐Kit receptor tyrosine kinase on PGCs, and its ligand, stem cell factor (Scf), expressed by somatic cells along the migratory route, are required (McCoshen and McCallion 1975; Buehr et al. 1993b; Merkwitz et al. 2011) as well as the chemokine stromal‐cell derived factor 1 (Sdf1) which is released from somatic cells and acts on the chemokine (C‐X‐C motif), receptor 4b (Cxcr4b), on the PGC surface (Ara et al. 2003; Molyneaux et al. 2003). Figure 1.2 Migration of human primordial germ cells. Representation of human primordial germ cell (PGC) migration from the allantois to the gonadal ridge in the intact embryo (a) and through the gut mesentery within the dissected abdomen (b) at approximately 6 weeks after conception. The gonadal ridge (G) has developed on the medial surface of the mesonephros (M) adjacent to the adrenal gland (A) and superior to the kidney (K). (c) Human embryo section corresponding to (b) showing PGCs darkly stained for alkaline phosphatase activity in the gonad (G) and throughout the folds of the gut mesentery (arrow). Bar = 250 µm. Reproduced with permission of Wiley. In addition, the following all interfere with PGC migration: knockout/mutation of β1 integrin (Anderson et al. 1999), E‐cadherin (Bendel‐Stenzel et al. 2000; Di Carlo and De Felici, 2000), Fgf8 (Sun et al. 1999), Forkhead box c1 (Foxc1) (Mattiske et al. 2006), Lhx1 (Tanaka et al. 2010), Wnt5a (Chawengsaksophak et al. 2012), Receptor tyrosine kinase‐like orphan receptor 2 (Ror2) (Laird et al. 2011), and the germ cell deficient (GCD) locus (Pellas et al. 1991). Extracellular matrix (ECM) proteins, including fibronectin and laminin, also play a role (Ffrench‐Constant et al. 1991; Garcia‐Castro et al. 1997). Inhibition of 3‐hydroxy‐3‐methylglutaryl‐coenzyme A reductase (HMGCR), involved in cholesterol synthesis, reduces PGC migration (Ding et al. 2008). Hindgut endoderm expansion is essential for mPGC migration (Hara et al. 2009), and the long and narrow genital ridge structure helps capture migrating germ cells (Harikae et al. 2013a). Human PGC migration is less well understood. During the fifth week of human embryonic development, PGCs are apparent in the genital ridges and gut mesentery. They migrate along nerve fibres and Schwann cells to reach the gonadal ridge, indicating that these nerve/Schwann cells release germ cell chemoattractants (Mollgard et al. 2010). Upon arrival in the genital ridge, germ cells (now termed gonocytes) lose their motility and polarized morphology and associate with somatic cells (Baillie 1964; Donovan et al. 1986). Studies in Drosophila and zebrafish indicate that PGCs stop migrating at the site of highest chemoattractant expression (Van Doren et al. 1998; Reichman‐Fried et al. 2004), and that somatic–germ cell interactions are also required (Jenkins et al. 2003; Van Doren et al. 2003; Mathews et al. 2006). A number of factors are involved in mPGC proliferation, which when ablated, cause PGC loss. Bcl‐x, an anti‐apoptotic B‐cell leukemia/lymphoma 2 (Bcl2) family member maintains the survival of mPGCs (Rucker et al. 2000), Fgf2 and Fgf4 promote mPGC proliferation in vitro (Matsui et al. 1992, Resnick et al. 1998, Kawase et al. 2004), and PGC numbers are reduced in Fgfr2‐IIIb knockout embryos (Takeuchi et al. 2005). As well as being required for migration, Kit signalling is required for germ cell growth, maturation and survival (Merkwitz et al. 2011). Loss of β‐catenin, a member of the Wnt signalling pathway, and follistatin (Fst), a Tgfβ family member, cause germ cell loss in the ovary (Yao et al. 2004; Liu et al. 2009). In addition, co‐expression of Wnt4 and Rspo1 is required for proliferation in the undifferentiated gonad (Chassot et al. 2012), as well as being involved later in ovarian development. As well as forming the gonad, the mesonephros and CE also give rise to components of the internal reproductive tract and urinary system, including the Wolffian ducts (WDs) which form the epididymides, vasa deferentia, and seminal vesicles in the male, and the Müllerian ducts (MDs) which generate the Fallopian tubes, uterus, and upper vagina in the female (Hashimoto 2003). In the human embryo, the internal reproductive tract is similar in both sexes up to 8 weeks postconception (WPC) (the indifferent stage). The precursor of the WD (also known as the mesonephric duct) is the pronephric duct (Jirasek 1971; Hashimoto 2003), which regresses at 4 WPC and is replaced by the mesonephros (Seville et al. 2002). The precursor of the MD, the paramesonephric duct, develops in parallel (Sobel et al. 2004). The WD first appears as a single uteric bud, and then develops as a continuous tube along the urogenital ridge, which reaches the caudal part of the hindgut, the cloaca. The WD develops by mesenchymal cell rearrangement, rather than by cell proliferation (Keller et al. 1985), involving Gata3 (Grote et al. 2006), Ret signalling (a receptor tyrosine kinase involved in glial derived neurotrophic factor signalling) (Hoshi et al. 2012), Gremlin1, Bmp4 and Bmp7 (Goncalves and Zeller 2011). The mature WD drains the primitive kidney, the mesonephros, to the cloaca. In males and females it develops into the trigone of the bladder, part of the bladder wall (Figure 1.3). Figure 1.3 Development of gonadal and internal reproductive system in males and females. Illustrated by Phoebe Ingram. The MDs are paired ducts, which run down the side of the urogenital ridge. They arise as a thickening of CE cells (Zhan et al. 2006; Arango et al. 2008), which migrate to the WD, proliferate and elongate at the tip (Guioli et al. 2007; Orvis and Behringer 2007). The Wolffian epithelium secretes Wnt9b, required for Müllerian growth (Carroll et al. 2005). MD development also requires retinoic acid (RA) (Mendelsohn et al. 1994), Wnt4 (Vainio et al. 1999; Heikkila et al. 2005), and Wnt7 which induces anti‐Müllerian receptor‐II (Amhr‐II) expression (Parr and McMahon 1998). Lim1 and Wnt7 expression is regulated by members of the Dachsung gene family (Davis et al. 2008). Knockout of Discs large homolog 1, involved in epithelial polarization and adhesion, causes defective MD development (Iizuka‐Kogo et al. 2007). During the third week of human development, the cloacal membrane is formed; this shifts caudally during the fourth week, and by the fifth week cloacal folds form on either side, joining at the anterior end, the genital tubercle. At 7 WPC, the urorectal septum divides the cloacal membrane, forming the urogenital membrane and urethral folds at the ventral section (urogenital sinus), and anal membrane and folds at the dorsal section. The urogenital membrane then dissolves leaving the urogenital sinus opening (ostium) surrounded by labioscrotal swellings (Figure 1.4). Figure 1.4 Development of external genitalia in both males and females. Illustrated by Phoebe Ingram. A number of signalling molecules are involved in the early patterning of the indifferent external genitalia. Fgf8, activated in the urethra by β‐catenin, and Bmps are required for genital tubercle growth and differentiation (Suzuki et al. 2003, Lin et al. 2008, Haraguchi et al. 2000), while Fgf10 is required for glans penis and clitoridis development (Haraguchi et al. 2000). Sonic hedgehog (Shh) signalling plays a central role (Perriton et al. 2002; Klonisch et al. 2004), as do various homeotic (Hox) genes (Mortlock and Innis 1997; Warot et al. 1997; Post and Innis 1999). Testis development is triggered by Sry (Lovell‐Badge and Robertson 1990; Koopman et al. 1991), a member of the SOX gene family of HMG transcription factors encoded on the Y chromosome. Sry is first detected in supporting cell precursors in the XY gonad from E10.75 (Albrecht and Eicher 2001; Bullejos and Koopman 2001; Sekido et al. 2004; Wilhelm et al. 2005). Expression is transient (approximately 4 h in each cell precursor), reaching a peak at E11.5 and being extinguished shortly after E12.5 (Koopman et al. 1990; Lee and Taketo 1994; Hacker et al. 1995; Jeske et al. 1995; Sekido et al. 2004), its function being to activate transcription of Sox9, the so‐called master regulator of testis determination, approximately 10 h after Sry expression (Bullejos and Koopman 2005). Gata4 activates the mouse, but not the human, Sry promoter (Miyamoto et al. 2008); this activation is enhanced via mitogen‐activated protein 3 kinase 4 (Map3k4) activation of p38 kinase, which phosphorylates Gata4 (Gierl et al. 2012; Warr et al. 2012). This activation requires interaction of Gata4 with its cofactor, Friend of Gata4 (Fog2) (Tevosian et al. 2002; Manuylov et al. 2011). Fog2 expression is in turn regulated by the transcription factors Six homeobox 1 (Six1) and Six4 (Fujimoto et al. 2013), which also regulate Sf1 expression. Map3k4 is activated by Growth arrest and DNA‐damage‐inducible protein (Gadd45g) (Miyake et al. 2007; Gierl et al. 2012) with null XY mice showing sex reversal (Warr et al. 2012; Johnen et al. 2013). Gata6 is co‐expressed with Gata4 in the testis (Ketola et al. 1999), with double knockout mice having smaller testes (Padua et al. 2015). Mouse Gata6 has 85% homology with Gata4 (Molkentin 2000) and the two are postulated to carry overlapping functions (Robert et al. 2006; Bennett et al. 2012). Sf1 activates the Sry promoter (de Santa Barbara et al. 2001; Pilon et al. 2003) and null XY gonads degenerate with due to lack of Sry expression (Luo et al. 1994). Lhx9 and Cbx2 regulate Sry expression indirectly via Sf1 upregulation, and Cited2 interacts with Sf1 and Wt1 to increase Sry expression to initiate testis development. Additionally, Wt1 directly activates the Sry promoter (Shimamura et al. 1997; Hossain and Saunders 2001; Miyamoto et al. 2008), synergizes with Gata4 on the Sry promoter (Miyamoto et al. 2008) and may stabilize Sry mRNA (Polanco and Koopman 2007). The transcription factor Sp1 also transactivates the Sry promoter (Desclozeaux et al. 1998; Assumpcao et al. 2005). Methylation of lysine 9 of histone H3 on the Sry promoter represses gene transcription (Barski et al. 2007), and is demethylated by lysine‐specific demethylase 3A (encoded by the Jmjd1a gene) (Kuroki et al. 2013). Jmjd1a‐null mice show XY sex reversal. A threshold level of Sry expression is required to activate Sox9 expression in Sertoli cells (SCs), but only within a specific window; if this does not occur, either ovotestes or ovaries form (Hiramatsu et al. 2009; Wilhelm et al. 2009). In addition, the level of Sox9 expression must also reach a threshold level. Once expression is initiated within SCs, however, it remains throughout their lifetime. Sry and Sf1 bind directly to several sites within the Sox9 promoter, within a 3.2 kb testis‐specific enhancer (TES) or 1.4 kb of its core element (TESCO), present approximately 14 kb upstream (Sekido and Lovell‐Badge 2008). Sox9 also binds to this region with Sf1 to maintain its own expression. Sox9 is the only critical direct target of Sry, as Sox9 expression in the XX gonad leads to male sex reversal (Bishop et al. 2000; Vidal et al. 2001). Deletion of Sox9 interferes with sex cord development and the activation of male specific markers (Chaboissier et al. 2004). In humans, heterozygous mutations cause campomelic dysplasia, with XY sex reversal (Foster et al. 1994; Wagner et al. 1994), and gain of function mutations cause XX sex reversal (Huang et al. 1999). There are also more distal regulatory regions of Sox9 (Bagheri‐Fam et al. 2006), mutation of which cause XY gonadal dysgenesis (White et al. 2011). Interestingly, mouse Sry can activate Sox9 directly, through its C terminal polyglutamine tract, but this has been lost in the human, which relies on Sry partner protein(s) to activate Sox9 transcription (Zhao et al. 2014). The promoter of Pod1, a basic helix‐loop‐helix (bHLH) transcription factor, contains Sry binding sites, and Pod1 promotes sex reversal of ovarian cells to Sertoli precursors (Bhandari et al. 2011). Null XY mice demonstrate defects in testis formation (Cui et al. 2004), indicating that Pod1 might be an Sry target; however it is expressed prior to Sry, and Pod1 knockout increases apoptosis. Its sex reversal effect may therefore occur because it represses Sf1 expression leading to Leydig cell and SC differentiation (Luo et al. 1994; Tamura et al. 2001). In the mouse testis, Sertoli‐secreted Ntf3 acts on its receptor Tropomyosin receptor kinase C (TrkC) to promote mesonephric cell migration (Cupp et al. 2003), and Sry activates the Ntf3 promoter (Clement et al. 2011). TrkC‐null mice show defective testis cord formation (Cupp et al. 2002). Another direct target of Sry and Sox9 is Cbln4 (Bradford et al. 2009), although the function of this secreted protein is unknown. As well as acting on its own promoter, Sox9 upregulates the expression of Fgf9 and prostaglandin D2 (Pgd2) synthase, creating feedforward loops which also maintain Sox9 expression. Pgd2 induces Sox9 expression and nuclear import in neighbouring cells (Wilhelm et al. 2005; Malki et al. 2005; Wilhelm et al. 2007; Moniot et al. 2009). Via interaction with its receptor Fgfr2, Fgf9 maintains Sox9 and downregulates Wnt4 expression (Kim et al. 2006; Kim et al. 2007). Fgf9‐ or Fgfr2‐null XY mice demonstrate complete or partial sex reversal, respectively (Colvin et al. 2001; Kim et al. 2007; Bagheri‐Fam et al. 2008), and Fgf9 causes proliferation of SC precursors (Schmahl et al. 2004; Kim et al. 2006). Sox9 upregulates the expression of AMH, secreted from SCs and involved in the development of the internal reproductive tract (Arango et al. 1999; Lasala et al. 2011). Sox8 is upregulated by Sox9 (Chaboissier et al. 2004) and cooperates with Sf1 to activate AMH transcription (Schepers et al. 2003). In addition, Sox3 and Sox10 are expressed in the mouse testis, and all three Sox proteins interact with Sf1 to maintain Sox9 expression (Sutton et al. 2011; Sekido and Lovell‐Badge, 2013). Later in development Sox8 and Sox9 synergize to promote basal lamina integrity of testis cords and suppress Forkhead box L2 (Foxl2) expression (Georg et al. 2012). The chromatin remodeler ATRX (α‐thalassemia and mental retardation associated with the X chromosome) functions in human sexual differentiation (Tang et al. 2004), with mutations causing gonadal and urogenital defects (Reardon et al. 1995). Mutations in testis‐specific protein Y‐like‐1 (TSPYL1), another chromatin modifier, cause sudden infant death with dysgenesis of the testis in males (SIDDT) (Puffenberger et al. 2004), along with other disorders of testicular development (Vinci et al. 2009). The transcription factor Mamld1 (Mastermind‐Like Domain‐Containing Protein 1) activates the transcription of a noncanonical Notch target gene hairy/enhancer of split 3 (Hes3) and augments testosterone production, likely regulated by Sf1 (Fukami et al. 2008). Dmrt1 (Doublesex and mab‐3 related transcription factor 1) maintains mammalian testis differentiation throughout development and postnatally (Matson et al. 2011; Minkina et al. 2014). It determines sex in a number of nonmammalian vertebrates (Matsuda et al. 2002; Yoshimoto et al. 2008; Smith et al. 2009), but is dispensable in mammals, in which it has been replaced by Sry (Raymond et al. 2000). However, overexpression in mouse XX gonads causes sex reversal (Zhao et al. 2015), indicating it has retained its ability to trigger testis differentiation. Duplication of the dosage sensitive sex reversal region on the X chromosome, encoding the transcription factor Dax1, causes sex reversal in XY patients (Bardoni et al. 1994; Swain et al. 1998). However, mutation in XX gonads does not prevent ovary development (Yu et al. 1998), and further investigations indicate that Dax1 is required for development of both the ovary and testis (Ludbrook and Harley 2004). As well as transcription factors, signalling molecules are involved in initiating the early stages of testis differentiation. Loss of function mutations in the insulin receptor, the insulin‐like growth factor receptor (Igf1r), and the insulin‐related receptor result in reduced Sry expression (Nef et al. 2003; Pitetti et al. 2013). However, these factors affect cell proliferation, which can cause XY ovary formation (Schmahl and Capel 2003). Male Desert Hedgehog (Dhh)‐null mice are sterile with reduced spermatogenesis (Bitgood and McMahon 1995), possibly due to decreased germ cell survival (Makela et al. 2011; Sahin et al. 2014). Dhh is secreted by SCs and its receptor Patched homologue 1 (Ptch1) is expressed by the interstitium, and also positively regulates fetal Leydig cell differentiation (Yao et al. 2002). In addition, palmitoyl‐transferase hedgehog acyl‐transferase (Hhat) is involved in testis cord formation and fetal Leydig cell differentiation (Callier et al. 2014). Testis cord formation is initiated by the clustering of pre‐SCs around germ cells, first evident as ‘proto‐cords’ in the mouse testis at 12 days postconception (DPC) and 7 WPC in the human (Francavilla et al. 1990; Heyn et al. 2001). Within 24 h in the mouse, definitive cords have formed (Nel‐Themaat et al. 2009; Combes et al. 2009a), made up of germ cells surrounded by epithelialized SCs (Nel‐Themaat et al. 2011), encased by peritubular myoid cells (PMCs, smooth muscle) and ECM (Maekawa et al. 1996; Skinner et al. 1985). This boundary tissue is termed lamina propria, and also contains myofibroblasts in humans (Davidoff et al. 1990; Dym 1994; Holstein et al. 1996). The cords elongate, causing expansion of the gonad, eventually forming the ‘spaghetti’‐like network of tubules seen in the mature adult testes (Combes et al. 2009a; Nel‐Themaat et al. 2009). A protective layer of fibrous tissue surrounds the testes. The main component is the tunica albuginea, formed due to basement membrane deposition just beneath the CE (Carmona et al. 2009), plus smooth muscle (Langford and Heller 1973) and contractile cells (Middendorff et al. 2002). Its rhythmic contractions regulate blood flow, sperm movement, and intertesticular pressure (Ohanian et al. 1979; Banks et al. 2006). Pre‐SCs originate from the CE and express Sry, which induces their differentiation to primitive SCs (Karl and Capel 1998; Albrecht and Eicher 2001; Bullejos and Koopman 2001), which are Sox9, Amh and Dhh positive (Josso et al. 1993; Morais da Silva et al. 1996; Park et al. 2005). The molecular mechanisms underpinning cord formation are not clearly understood, but involve Sertoli‐derived nerve growth factor 3 and its receptors Ntrk1 and Ntrk3 (Russo et al. 1999; Cupp et al. 2000; Levine et al. 2000), involved in forming adhesive cell contacts (Cupp et al. 2002,; Gassei et al. 2008), and Fgf9 diffusion (Hiramatsu et al. 2010). Tgfβ, activin, and inhibin b signalling also play a role (Yao et al. 2006; Memon et al. 2008; Sarraj et al. 2010; Liu et al. 2010; Miles et al. 2013). Germ cells themselves do not provide the trigger for cord formation, as cords develop normally in XY gonads without germ cells (Merchant 1975; McCoshen 1982; McCoshen 1983; Escalante‐Alcalde and Merchant‐Larios 1992). In contrast, germ cell progression through meiosis is essential for ovarian development (Adams and McLaren 2002). Vascularization of the gonadal ridge, the formation of the major coelomic vessel and interstitial microvasculature, is crucial for cord formation (Cool et al. 2008; Coveney et al. 2008; Brennan et al. 2002; Combes et al. 2009b). Migration of vascular endothelial cells requires endothelial expressed platelet‐derived growth factor B (PDGF‐B) and mesenchymal expressed vascular endothelial growth factor A (VEGF‐A) (Brennan et al. 2003; Bott et al. 2006; Cool et al. 2011). Yolk sac derived macrophages also mediate vascular reorganization (DeFalco et al. 2014). Mesonephric cell migration into the gonad is also necessary for cord formation (Buehr et al. 1993a; Martineau et al. 1997; Tilmann and Capel 1999), the cells of which contribute to the Leydig and endothelial cell populations (Martineau et al. 1997; Merchant‐Larios and Moreno‐Mendoza 1998). Cell migration requires pre‐SCs lying beneath the CE (Tilmann and Capel 2002) and Fgf9 (Colvin et al. 2001). Human PGC number increases rapidly in the testis from ~3000 at 6 WPC to ~30 000 at 9 WPC (Bendsen et al. 2003). At around 41–44 days postconception, between E12.5 and E14.5 in the mouse, PGCs begin to enter mitotic arrest in G0/G1 as prospermatogonia, associated with SC differentiation and testicular cord formation (Gondos and Hobel 1971; Western et al. 2008), resuming mitosis after birth (McLaren 1984), with meiosis delayed until well after birth (McLaren 1988). Mitotic arrest is induced by expression of the cell cycle regulator retinoblastoma 1 (Spiller et al. 2010). The chromosomal make‐up of germ cells does not influence their sex differentiation, XX germ cells in the testis will differentiate to spermatogonia, whereas XY germ cells in the ovary develop into oogonia (McLaren 2000), demonstrating that somatic secreted factors play a determining role. RA is secreted from the mesonephros in XX and XY gonads (Bowles et al. 2006), and somatic cells in the testis (Bowles et al. 2009) due to expression of retinaldehyde dehydrogenases (ALDHs). Meiosis in the fetal testis is antagonized by Fgf9 expression, which reduces the responsiveness of germ cells to RA (Barrios et al. 2010; Bowles et al. 2010) and Sox9/Sry‐induced expression of Cyp26b1 (Bowles et al. 2006; MacLean et al. 2007; Kashimada et al. 2011b), a P450 enzyme that degrades RA. The human fetal testis, however, may respond to RA, as RA receptors are present but CYP26B1 is absent (Cupp et al. 1999; Childs et al. 2011). Fgf9 also prolongs germ cell pluripotency by stimulating the expression of the Nodal coreceptor Cripto (Bowles and Koopman 2010; Spiller et al. 2012). Fsh promotes the survival of germ cells (Meachem et al. 2005), and Tgfβ and Activin A regulate quiescence (Moreno et al. 2010; Mendis et al. 2011). The androgen producing cells of the testis, the Leydig cells, are found within the interstitial compartment at around 8 WPC in the human testis (Codesal et al. 1990). However, their origin is unknown (Griswold and Behringer 2009; DeFalco et al. 2011; Barsoum et al. 2013). Their initial differentiation is induced by SC‐derived PDGF binding to the PDGFRα (Brennan et al. 2003), the paracrine action of Dhh (Barsoum et al. 2009; Huang and Yao 2010) and Notch signalling (Tang et al. 2008). Human fetal testosterone production is detectable by 9 weeks, peaks between weeks 15 and 16, before dropping sharply (Reyes et al. 1974). Initial androgen production does not require gonadotrophin stimulation (Word et al. 1989) but placental‐derived human chorionic gonadotrophin (hCG) and, in the third trimester, fetal pituitary luteinizing hormone (LH), regulate final differentiation and androgen production (Rabinovici and Jaffe 1990; Mendis‐Handagama 1997). Leydig cell‐derived testosterone and insulin‐like growth factor 3 (Insl3) cause WD stabilization and differentiation into the epididymis, vas deferens, and seminal vesicle, masculization of the external genitalia, and testicular descent (Nef and Parada 1999; Klonisch et al. 2004; Hannema and Hughes 2007; Feng et al. 2009; Ivell and Anand‐Ivell 2011). Insl3 controls the first, transabdominal phase of testicular descent, by stimulating gubernaculums testis development (Kumagai et al. 2002). Testosterone is converted to dihydrotestosterone (DHT) by 5α‐reductase, which has a higher affinity for the androgen receptor (AR) and thus is a more potent driver of external genitalia and prostate development (Wilson et al. 1981; Imperato‐McGinley and Zhu 2002). In addition, the testis itself produces DHT via a testosterone‐independent pathway (Wilson et al. 2002). The AR translocates to the nucleus upon stimulation and binds to androgen response elements to regulate gene transcription (Roche et al. 1992; Jenster et al. 1993). androgens are responsible for the inguinoscrotal phase of testicular descent (Su et al. 2012) and the disappearance of the cranial suspensory ligament (van der Schoot and Elger 1992). The MDs regress at around 8 WPC due to apoptosis (Roberts et al. 1999; Allard et al. 2000), in turn, due to Sertoli‐derived AMH (Josso et al. 2006, Josso et al. 2012). AMH is a member of the Tgfβ family (Cate et al. 1986), whose expression is triggered by Sox9 (Arango et al. 1999), increased by Sf1, Gata4, and Wt1 (Watanabe et al. 2000; Hossain and Saunders 2003; Viger et al. 2008), and stimulated by Fsh postnatally (Al‐Attar et al. 1997; Lukas‐Croisier et al. 2003; Young et al. 2005). Up to around 9 WPC, the external genitalia remain undifferentiated (Jirasek 1977). The genital tubercle elongates to form the penis in males, beginning around 9 WPC by lengthening of the angogenital distance (Jirasek 1977). Part of the cloacal folds form the urogenital folds, which surround the urogenital ostium laterally. Fusion of the labioscrotal folds occurs, forming the epithelial seam of the scrotum (Baskin et al. 2001). The proximal urethra forms by fusion of the urethral folds around the urethral plate, and the distal urethra arises from an invagination of the apical ectoderm. The urethral folds fuse in the midline converting the urethral groove into the penile urethra, which is formed by 14 WPC; however, there is no difference in penile and clitoral size until 14 WPC (Feldman and Smith 1975; Zalel et al. 2001). The third trimester sees maximal phallic growth, curiously at a time when testosterone levels are declining (Winter et al. 1977; O’Shaughnessy et al. 2007) The urogenital sinus is the precursor to the bladder, urethra, and prostate and is formed in response to androgens on E13.5 (approx. 6 WPC) as cylindrical gut endoderm surrounded by mesenchyme (Goldstein and Wilson 1975; Cunha and Lung 1978). Up to around 9 WPC, it remains undifferentiated. Solid epithelial outgrowths (prostatic buds) form by E16.5 in the mouse, or 10 WPC in the human (Cunha et al. 1987). There is a period of quiescence in the human, until puberty, when increased androgen levels promote prostatic growth, forming the complex ductal network of the prostatic gland (Glenister 1962; Berry et al. 1984). The prostatic utricle forms – the male equivalent of the vagina, as an epithelial‐lined diverticulum of the prostatic urethra – it serves no function (Glenister 1962). Ovarian development is generally considered to be the default pathway (Jost 1947; Burgoyne 1988; Goodfellow and Darling 1988), occurring in the absence of Sry expression and the presence of Wnt4, Fst, and Foxl2 (Tevosian 2013). Ovarian development involves germ cell meiosis and apoptosis, granulosa cell differentiation, and primordial follicle formation. The RNA‐binding protein Dazl is one of the first factors expressed by PGCs required for ovarian development. It is detected shortly after PGC migration (Cooke et al. 1996) and knockout causes oocyte loss at the time of meiotic entry (McNeilly et al. 2000). It is thought to enable the gonads to respond to ovarian cues (Gill et al. 2011). Figlα is a transcription factor expressed by oocytes from E13 (Liang et al. 1997), required for germ cell survival and primordial follicle formation (Soyal et al. 2000; Lei et al. 2006). In the absence of Sry, Wnt4 is expressed in the female gonad from E12.5 (Heikkila et al. 2005) and represses Fgf9 and Sox9 expression and stabilizes β‐catenin (Kim et al. 2006), as well as upregulating Dax1, which antagonizes Sf1 and thereby inhibits steroidogenic enzymes (Jordan et al. 2001). Wnt4 is required for female germ cell survival (Yao et al. 2004) and null XX embryos exhibit masculinized gonads (Vainio et al. 1999). It prevents the production of steroids and the formation of the male‐specific coelomic blood vessels by preventing the binding of β‐catenin to Sf1 sites on steroidogenic genes (Jeays‐Ward et al. 2003; Jordan et al. 2003). Rspo1 is essential for ovarian development in several vertebrate species, and upregulates Wnt4 in a cooperative manner to increase β‐catenin and Fst levels (Yao et al. 2004; Parma et al. 2006; Chassot et al. 2008; Kim et al. 2008; Smith et al. 2008; Tomizuka et al. 2008). β‐catenin then activates Wnt4 expression in a positive feedback loop (Chang et al. 2008). Rspo1 knockout impairs ovarian development, but does not cause sex reversal (Chassot et al. 2008; Tomizuka et al. 2008), and overexpression does not perturb testis differentiation (Buscara et al. 2009). Rspo1 stimulates germ cell proliferation, and with Wnt4 regulates germ cell entry into meiosis (Naillat et al. 2010; Chassot et al. 2011) and maintains pregranulosa cell quiescence (Maatouk et al. 2013). Human RSPO1 is upregulated between 6 and 9 WPC, and augments β‐catenin signalling (Tomaselli et al. 2011). β‐catenin regulates germ cell fate, possibly by regulating cell–cell adhesion (Fleming et al. 2012) along with Wnt4 (Naillat et al. 2010). It prevents Sf1 binding to the Sox9 TESCO enhancer, inhibiting Sox9 expression and SC differentiation (Bernard et al. 2012). It also induces Fst expression, which represses Activin B thus inhibiting endothelial cell migration and coelomic vessel formation (Yao et al. 2004; Yao et al. 2006). Ablation of Rspo1, Wnt4 and β‐catenin causes development of seminiferous tubules in XX gonads (Chassot et al. 2008), indicating that the three genes together are required to suppress the male pathway. Fst acts downstream of Wnt4 to promote germ cell survival (Yao et al. 2004), and knockout causes infertility (Kimura et al. 2010; Kimura et al. 2011). Wnt4 is required to initiate, but not maintain, Fst expression; this requires Bmp2 and Foxl2 (Kashimada et al. 2011a). Bmp2 is expressed in the gonad at E12.5 (Yao et al. 2004), but its role in ovarian development is unknown. The Gata4 and Fog2 interaction, required for testis formation, is also required for early ovarian differentiation, with knockout resulting in multiple defects including reduced Fst, Wnt4, and Foxl2 expression (expression of Sf1 is not affected) (Manuylov et al. 2008). The Gata4‐Fog2 complex serves as a repressor of Dickkopf Wnt signalling pathway inhibitor 1 (Dkk1), which inhibits β‐catenin signalling. Between 10.5 and 13.5 DPC in the mouse ovary, mitotic germ cells (oogonia) develop as clusters of interconnected cells, termed germ cell cysts (Pepling and Spradling 1998). Cyst formation occurs due to incomplete mitosis, with daughter germ cells remaining connected to one another by intercellular bridges (McKearin and Ohlstein 1995). Whilst within these cysts, germ cells lose expression of Oct4 (Pesce et al. 1998) and enter meiosis, at around E13.5 in the mouse, and approximately 12 WPC in the human (Gondos and Hobel 1971). The intercellular bridges breakdown and the oocytes become enclosed within ovigerous cords, forming ‘pregranulosa cells’ surrounded by a basal lamina (Odor and Blandau 1969; Gondos 1987; Pepling and Spradling 1998). There are two waves of pregranulosa cell recruitment from the surface epithelium; one just before sexual differentiation and the second immediately postbirth during follicle formation (Harikae et al. 2013b). RA binding to its receptor causes meiotic entry of germ cells, stimulated by retinoic acid gene 8 (Stra8) gene expression (Baltus et al. 2006; Koubova et al. 2006; Childs et al. 2011). Stra8 functions in premeiotic DNA replication and chromosome cohesion and synapsis (Baltus et al. 2006). Sycp1 (Synaptonemal complex protein 1) (de Vries et al. 2005), Sycp3 (Di Carlo et al. 2000; Yuan et al. 2002) and Rec8 (yeast meiotic recombination protein Rec8 homologue) (Prieto et al. 2004) are then expressed, which are involved in the formation of the meiotic synaptonemal and cohesion complexes respectively, marking the beginning of prophase I. Germ cells that do not undergo cell death progress through leptonema, zygonema, pachynema, and diplonema, entering a prolonged arrest stage termed dictyate around the time of birth (Borum 1961; Borum 1967; Speed 1982
Sexual Differentiation, Gonadal Development, and Development of the External Genitalia: A Review of The Regulation of Sexual Differentiation
Introduction
The Bipotential Gonad
Molecular Determinants of Gonadal Development
Empty spiracles homeobox 2 (Emx2)
Paired box gene 2 (Pax2)
Transcription factor 2 (Tcf2)
Steroidogenic factor 1 (Sf1)/Nr5a1
Wilms’ tumour 1 (Wt1)
LIM homeobox 9 (Lhx9)
Chromobox homologue 2 (Cbx2)
CBP/p300 interacting transactivator, with glu/asp‐rich c‐terminal domain, 2 (Cited2)
Gata binding protein 4 (Gata4)
Primordial Germ Cells
Specification
Migration
Proliferation
The Internal Reproductive Tract
The External Genitalia
Testis Differentiation
Molecular Determinants of Testis Differentiation
Regulation of Sry Expression
Sry Targets
Sox9
Pod1 (Transcription factor 21, Tcf21)
Neurotrophin 3 (Ntf3)
Cerebellin 4 precursor gene (Cbln4)
Sox9 Targets
Fgf9 and Prostaglandin D2 (Pgd2)
Anti‐Müllerian hormone (AMH)
Sox Family Members
Other Factors Involved in Testis Differentiation
Cord Formation
Germ Cells
Somatic Factors Acting on XY Germ Cells
Leydig Cells
Male Differentiation of the Internal Reproductive Tract
Male Differentiation of the External Genitalia
Ovarian Differentiation
Germ Cells
Survival and Proliferation
Deleted in AZoospermia (Dazl)
Factor in Germ Line α (Figlα)
Wnt4
R‐spondin1 (Rspo1)
Fst
Gata4‐Fog2 interaction
Meiosis
Stay updated, free articles. Join our Telegram channel

Full access? Get Clinical Tree
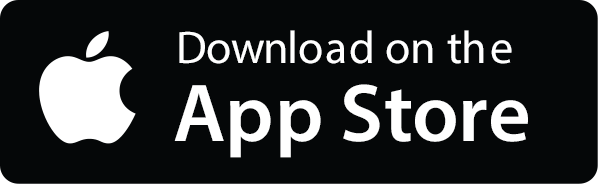
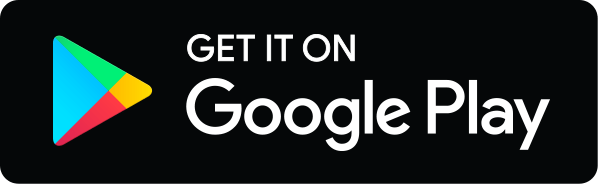