Sense Organs
SENSORY RECEPTORS
Sense organs called sensory receptors make it possible for the body to respond to stimuli caused by changes occurring in our external or internal environment. This function is crucial to survival. The abilities to see and hear, for example, may provide the necessary warning to help us avoid injury from dangers in our external environment. Internal sensations ranging from pain and pressure to hunger and thirst help us maintain homeostasis of our internal environment.
Receptor Response
The general function of receptors is to respond to stimuli by converting them to nerve impulses. Receptors are often described as the sensitive dendritic endings or “end organs” of sensory neurons. As a rule, different types of receptors respond to different types of stimuli. Heat receptors, for example, do not respond to light or stretch stimuli.
When an adequate stimulus acts on a receptor, a local potential develops in the receptor’s membrane. This receptor potential is a graded response, graded to the strength of the stimulus (see Chapter 13, p. 395). When a receptor potential reaches a certain threshold, it triggers an action potential in the sensory neuron’s axon. These impulses then travel over sensory pathways to the brain and spinal cord, where they are interpreted as a particular sensation, such as heat or cold, or they initiate some type of reflex action, such as withdrawal of a limb from a painful stimulus.
Certain sensory impulses terminating in the brainstem may affect so-called “vital sign” reflexes that help regulate heart or respiratory rate. Others may end in the thalamus or cerebral cortex where they trigger imprecise or “crude” sensation awareness (thalamus) or very precise and specific awareness of not only a specific type of sensation but also its exact location and level of intensity (cerebral cortex).
Receptors often exhibit a functional characteristic known as adaptation. Adaptation refers to the process by which the magnitude of the receptor potential decreases over time in response to a continuous stimulus (Figure 17-1). As a result, the rate of impulse conduction by the sensory neuron’s axon also decreases. So too does the intensity of the resulting sensation. A familiar example of adaptation is feeling the touch of your clothing when you first put it on and then soon not sensing it at all. Touch receptors adapt rapidly. In contrast, the proprioceptors in our muscles, tendons, and joints adapt slowly. As long as stimulation of them continues, they continue sending impulses to the brain.
If we not only remain aware of a particular sensation over time, but also interpret what that sensation means in a larger context, the process is called perception. Although we may have no conscious perception of certain sensory inputs, they often play a critical role in maintaining homeostasis. Examples might include our ability to sense and respond to changing levels of blood glucose and carbon dioxide—but not at a conscious level.
Distribution of Receptors
Receptors responsible for the special senses of smell, taste, vision, hearing, and equilibrium are grouped into localized areas (such as nasal mucosa or the tongue) or into complex organs such as the eye and ear. The general sense organs, on the other hand, consist of microscopic receptors widely distributed throughout the body in the skin, mucosa, connective tissues, muscles, tendons, joints, and viscera.
Sensations produced by the receptors of general sense organs are often called the somatic senses. The distribution of general sense receptors is not uniform in all areas. In some, it is very dense; in others, it is sparse. The skin covering the fingertips, for instance, contains many more receptors to touch than does the skin on the back. A simple procedure, the two-point discrimination test, demonstrates this fact. A subject reports the number of touch points felt when an investigator touches the skin simultaneously with two points of a compass. If the skin on the fingertip is touched with the compass points barely ⅛ inch apart, the subject senses them as two points. If the skin on the back is touched with the compass points this close together, they will be felt as only one point. Unless they are 1 inch or more apart, they cannot be discriminated as two points. Why this difference? Because touch receptors are so densely distributed in the fingertips that two points very close to each other still stimulate two different receptors—they are sensed as two points.
The situation is quite different in the skin on the back. There, touch receptors are so widely scattered that two points have to be at least 1 inch apart to stimulate two receptors and be felt as two points.
CLASSIFICATION OF RECEPTORS
Receptors can be classified according to (1) their location in the body, (2) the particular stimulus that causes them to respond, and (3) their structure.
Classification by Location
Three groups or classes of receptors can be identified by their location:
Exteroceptors, as the name implies, are located on or very near the body surface and respond most frequently to stimuli that arise external to the body itself. Receptors in this group are sometimes called cutaneous receptors because of their placement in the skin. However, the special sense organs, which are described later in the chapter, are also classified as exteroceptors. Examples of exteroceptors include those that detect pressure, touch, pain, and temperature.
Visceroceptors (interoceptors) are located internally, often within the substance of body organs (viscera), and when stimulated provide information about the internal environment. They are activated by stimuli such as pressure, stretching, and chemical changes that may originate in diverse internal organs, such as the major blood vessels, intestines, and urinary bladder. Visceroceptors are also involved in mediating sensations such as hunger and thirst.
Proprioceptors are a special type of visceroceptor. They are less numerous and generally more specialized than other internally placed receptors, and their location is limited to skeletal muscle, joint capsules, and tendons. Proprioceptors provide us with information about body movement, orientation in space, and muscle stretch.
Activation of two types of proprioceptors, called tonic and phasic proprioceptors, allows us to orient our body in space and provides us with positional information about specific body parts while at rest or during movement. The firing of the nonadapting tonic proprioceptors allows us to locate, for example, our arm, hand, or foot at rest without having to look. Phasic proprioceptors are rapidly adapting receptors, so they are triggered only when there is a change in position. Phasic proprioceptors therefore permit us to feel the changing position of our body parts during continuous movement.
Classification by Stimulus Detected
Receptors are frequently classified into six categories based on the types of stimuli that activate them:
Classification by Structure
Regardless of their location or how they are activated, the general (somatic) sensory receptors may be classified anatomically as either of the following:
Refer often to Table 17-1 as you read about the general sensory receptors that are described in the following paragraphs.
TABLE 17-1
Classification of Somatic Sensory Receptors
BY STRUCTURE | BY LOCATION AND TYPE | BY ACTIVATION STIMULUS | BY SENSATION OR FUNCTION |
Free Nerve Endings | |||
![]() | Either exteroceptor or visceroceptor–most body tissues | Almost any noxious stimulus; temperature change; mechanical | Pain; temperature; itch; tickle |
![]() | Exteroceptor | Light pressure; mechanical | Discriminative touch |
![]() | Exteroceptor | Hair movement; mechanical | Sense of “deflection” type of movement of hair |
Encapsulated Nerve Endings | |||
Touch and Pressure Receptors | |||
![]() | Exteroceptor; epidermis, hairless skin | Light pressure, mechanical | Touch; low-frequency vibration |
![]() | Exteroceptor; mucous membranes | Mechanical | Touch; low-frequency vibration; textural sensation |
![]() | Exteroceptor; dermis of skin | Mechanical | Crude and persistent touch |
![]() | Exteroceptor; dermis of skin, joint capsules | Deep pressure, mechanical | Deep pressure; high-frequency vibration; stretch |
Stretch Receptors | |||
![]() | Interoceptor; skeletal muscle | Stretch; mechanical | Sense of muscle length |
![]() | Interoceptor; tendon (near muscle tissue) | Force of contraction and tendon stretch; mechanical | Sense of muscle tension |
FREE NERVE ENDINGS
Free nerve endings are the simplest, most common, and most widely distributed sensory receptors. They are located both on the surface of the body (exteroceptors) and in the deep visceral organs (visceroceptors). These slender sensory fibers often terminate in small swellings called dendritic knobs.
Pain Sensations
The term nociceptor is used to describe the free nerve endings that serve as the primary sensory receptors for pain. Nerve fibers that carry pain impulses from nociceptors to the brain can be divided into two types—acute or fast (A) pain fibers and chronic or slow (B) pain fibers.
A fibers are concentrated in the skin, mucous membranes, and other superficial areas. Fast pain is sometimes described as a sharp “take your breath away” type of pain associated with superficial injury or trauma. If you have ever slammed your finger while closing a car door you have experienced this type of fast or somatic pain.
The type of deep or visceral pain that develops more slowly over time and travels over B fibers is often described as dull or aching. It originates in deeper body (visceral) structures and can be severe if caused by conditions such as intestinal obstruction or passage of a kidney stone or gallstone.
It is important to stress that in responding to powerful stimuli of any kind, including chemical or thermal burns, intense light, sound, or pressure, the generation of a receptor potential in free nerve endings most often results in the sensation of pain—often the first indication of injury or disease.
Brain tissue is unique in that it lacks the type of nociceptors that transmit sensations of pain and is therefore incapable of sensing painful stimuli. Just the opposite is true of many deep visceral organs, in which the presence of free nerve endings makes pain one of the few sensations that can be evoked.
Pain is experienced differently by different people. Many factors cause this—many of which are unknown. Certainly the brain’s filtering and processing of sensory information plays a large part. Box 17-1 describes a common phenomenon in which visceral pain is perceived as external pain.
Pain is a “bad news/good news” type of sensation. The bad news, of course, is its relationship to disease and injury. The good news is the important role pain plays in alerting us to threats in our environment. Pain expert Paul Brand often called pain “the gift that nobody wants.” Individuals who are unable to adequately sense pain on the body surface tend to have other types of sensory loss as well. They are very much at risk for injury because they lack the ability to sense the “warning signs” that a normally functioning sensory system can provide.
Individuals with uncontrolled or poorly controlled diabetes often lose their ability to sense pain on certain areas of the body surface—especially on the skin of the feet. Other sensations are also frequently diminished or lost over time in diabetics because of nerve damage called diabetic neuropathy. For this reason physicians closely monitor the adequacy of cutaneous sensation on the bottom of the foot (Figure 17-2, A). Recognizing early signs of diabetic neuropathy can help prevent later injury. Figure 17-2, B, shows a foreign body (a piece of wire) protruding from the tip of the third toe in a diabetic patient. The individual lacked sensation in the feet and only after visually noticing it sought medical attention. This is just one example, but a common one, of a failure of “the gift of pain” to give an appropriate warning of an injury.
In a syndrome called fibromyalgia (FM), chronic and widespread musculoskeletal pain is usually accompanied by distress and a variety of other symptoms. The primary mechanism of FM seems to be an abnormal amplification of pain information processed in the central nervous system. However, other mechanisms are also being investigated as contributing factors. The drug pregabalin (Lyrica) reduces the pain of FM mainly by blocking calcium channels in the pain-pathway neurons of the spinal cord. Reducing calcium influx, as you probably recall (see Figure 13-27 on p. 402), inhibits the release of pain neurotransmitters.
Temperature Sensations
Free nerve endings called thermoreceptors mediate sensations of heat and cold. When small cold or warm probes are used to “map” the skin’s sensitivity to temperature, small areas called “receptive fields” about 1 mm across can be identified on the skin surface as being sensitive to either cold or warmth, but not both. Research has shown that these receptive fields represent separate warm receptors and cold receptors that respond to different thermal sensations and are sensitive to a range of relatively hot or cold temperatures. Thermal maps have also shown that thermoreceptors, like other types of cutaneous receptors, are not spread uniformly across the skin surface.
Both types of thermoreceptors undergo rapid adaptation. As a result, the intensity of the warm or cold sensations they initially produce when activated soon fade. We adapt to the heat of a sauna or a cool shower in a short time.
Warm receptors, which are located in the dermis, are activated above about 25° C (77° F) and increase their firing rate until the temperature reaches about 46° C (114° F). Figure 17-3 shows the range of temperature receptor sensitivity. Beyond a temperature of about 48° C (118° F) a sensation of burning pain begins.
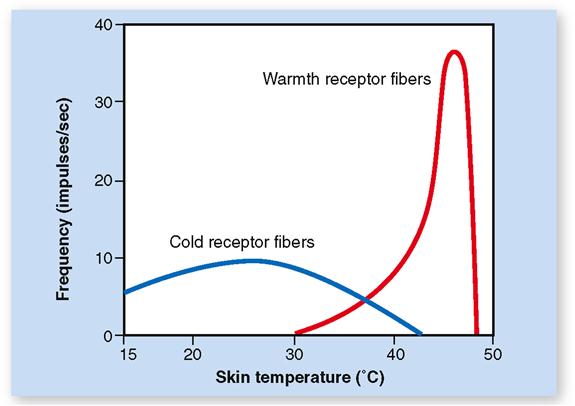
Cold receptors, which are located in the deepest layer of the epidermis, have a broader temperature response than warm receptors (see Figure 17-3). They are most active between about 10° C (50° F) and 40° C (104° F). Below 10° C the firing of cold receptors decreases dramatically. The falling temperature first acts as a local anesthetic and then activates nociceptors resulting in a sensation of freezing pain. Between temperature extremes the brain senses a particular temperature sensation by integrating sensory inputs from both receptor types.
Tactile Sensations
In addition to their role in mediation of pain and temperature, free nerve endings and slightly modified free nerve endings are also involved in certain tactile sensations.
Skin Movement
Root hair plexuses are rapidly adapting free nerve endings that are activated when very slight skin movement bends or deforms a hair shaft or follicle surrounded by the receptor. When you feel a mosquito “bite,” it may not be caused by the piercing of the skin by the mouth of the insect. Instead, it may be the movement of your own skin caused by the mosquito’s activity that triggers or stimulates a root hair plexus. In any event, it’s a good idea to swat the mosquito!
Itch
The term “itch” is used to describe several different tactile sensations mediated by free nerve endings. Most people would describe it as a sensation that makes you want to scratch. It can vary in intensity from almost imperceptible to intense and disabling. The cause is generally chemical irritation of free nerve endings by inflammatory chemicals, such as bradykinin or histamine. These types of chemicals are often released by injured tissue following insect bites, or during allergic reactions. But itches can also be induced by suggestion, like a yawn. Scientists are just now working out the types of itch that occur in humans and how these itch mechanisms work.
Tickle
Tickle is a unique sensation in that it most often results from tactile stimulation of the skin, not by you, but by someone else. It is mediated by free nerve endings and is a good but somewhat baffling example of how perception can alter the conscious interpretation of a nervous impulse when it reaches the brain. We know that neural pathways in tickle involve both the thalamus and cerebellum before the impulses reach the cerebral cortex. However, the circuits involved in the cerebral cortex and how these circuits interact with neurons in other areas of the brain are not well understood. Obviously, knowing consciously that a particular tactile sensation generally occurs only when you are touched by someone else requires complex nervous system involvement. What begins as a simple receptor potential in a free nerve ending ends in a complex, consciously interpreted tactile sensation.
Discriminative Touch
Discriminative touch, or “light touch,” refers to an often very subtle sensation that can be located exactly in certain areas of the skin (Figure 17-4). It is mediated by a flattened or disk-shaped variation of a free nerve ending called a tactile disk or Merkel disk. It may also be called a tactile meniscus. This structure was first described by the German anatomist Friedrich Merkel (1885-1919) when he studied delicate nervous elements near the skin surface.
Structurally, the tactile receptor unit is made up of two cells. One is an epithelial cell called the tactile epithelial cell, located in the epidermis of the skin (see Figure 6-4, p. 138). The other cell is a sensory neuron called the tactile disk. The tactile epithelial cell is rather stiff and transmits compression of the outer layer of the skin to the tactile disk neuron. The tactile disk is a delicate mechanoreceptor that is not encapsulated and has a superficial placement in the epidermis of the skin (Figure 17-5, A). It is therefore easily “deformed” when pressed by the stiff tactile cell and capable of generating an action potential when exposed to very minimal stimulation. Tactile disks adapt slowly, thus maintaining information flow to the central nervous system for some time.
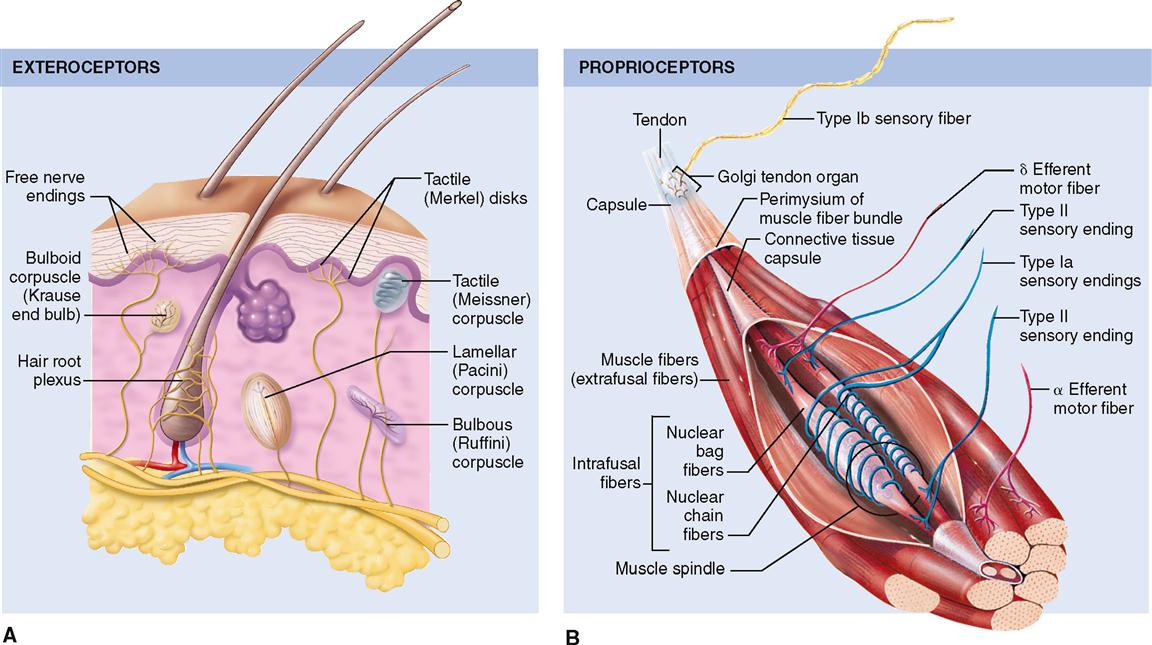
In addition to discriminative or light touch, the tactile receptor unit is also capable of detecting subtle changes in surface form and contours.
ENCAPSULATED NERVE ENDINGS
The six types of encapsulated nerve endings have in common some type of connective tissue capsule that surrounds their terminal or dendritic end. In addition, most of the encapsulated receptors are primary mechanoreceptors. Thus they are most often activated by a mechanical or “deforming” type of stimulus. Encapsulated receptors vary in size and anatomical characteristics, as well as in numbers and distribution throughout the body (see Table 17-1).
Touch and Pressure Receptors
Tactile corpuscles are encapsulated tactile end organs, also called Meissner corpuscles, first described by the German physiologist George Meissner (1829-1905). Note in Figure 17-5, A, and Table 17-1 that they are superficially placed ovoid or egg-shaped mechanoreceptors that are larger than tactile disks. The encapsulated receptor fibers are coiled and enmeshed in connective tissue. They are located in or very close to the dermal papillae in hairless skin areas such as the fingertips, lips, nipples, and genitals. Although their covering capsule requires slightly more of a “deforming” stimulus to generate an action potential than a tactile (Merkel) disk, they do mediate light touch in addition to textural sensations and low-frequency vibration.
Two important anatomical variants of tactile (Meissner) corpuscles also act as mechanoreceptors. One is called the bulboid corpuscle or Krause end bulb. Bulboid corpuscles are egg shaped, like tactile corpuscles, but they are somewhat smaller and have fewer and less tightly coiled dendritic endings within their covering capsule. These receptors are more numerous in mucous membranes than in skin and are sometimes called mucocutaneous corpuscles. They are involved in touch and low-frequency vibration.
The other variant of the tactile (Meissner) corpuscle is the bulbous corpuscle, also known as the Ruffini corpuscle (see Figure 17-5, A). Although considered to be a variant of the tactile corpuscle, it has a more flattened capsule and is more deeply located in the dermis of the skin. These receptors mediate sensations of crude, heavy, and persistent touch. Because they are slow adapting, they permit the skin of the fingers to remain sensitive to deep pressure for long periods. The ability to grasp an object, such as the steering wheel of a car, for long periods of time and still be able to “sense” its presence between the fingers depends on these receptors.
Lamellar corpuscles (Pacini corpuscles) are large mechanoreceptors, which, when sectioned, show thick laminated connective tissue capsules. They are found in the deep dermis of the skin—especially in the hands and feet—and are also numerous in joint capsules throughout the body (see Figure 17-5, A). Pacini, or lamellar, corpuscles respond quickly to sensations of deep pressure, high-frequency vibration, and stretch. Although sensitive and quick to respond, these receptors adapt quickly, and the sensations they evoke seldom last for long.
Stretch Receptors
The most important stretch receptors are associated with muscles and tendons and are classified as proprioceptors. Two types of stretch receptors, called muscle spindles and Golgi tendon receptors, operate to provide the body with information concerning muscle length and the strength of muscle contraction (see Figure 17-5, B).
Anatomically, each muscle spindle consists of a discrete grouping of about 5 to 10 modified muscle fibers called intrafusal fibers, which are surrounded by a delicate capsule. These fibers have striated ends that are capable of contraction but are devoid of contractile filaments in their central areas where, instead, there are several nuclei surrounded by clear cytoplasm and two types of sensory nerve fibers that are described below. The muscle spindles can be found lying between and parallel to the regular muscle fibers called extrafusal fibers. Both ends of each spindle are connected or anchored to connective tissue elements within the muscle mass.
Two types of sensory (afferent) nerve fibers are found encircling the central area of each spindle. Both large-diameter and rapidly conducting type Ia and slower-conducting, small-diameter type II fibers encircle the clear central area of each spindle. When stretching occurs, afferent impulses from these sensory neurons pass to the spinal cord and are relayed to the brain, providing a mechanism to monitor changes in muscle length.
The striated ends of the muscle spindle fibers (intrafusal fibers) are capable of contraction when stimulated by efferent impulses generated in gamma motor neurons, whereas regular muscle fibers (extrafusal fibers) are stimulated to contract by efferent impulses generated in alpha motor neurons. Both types of neurons are located in the anterior gray horn of the spinal cord.
Muscle spindles are stimulated if the length of a muscle is stretched and exceeds a certain limit. The result of stimulation is a stretch reflex that shortens a muscle or muscle group, thus aiding in the maintenance of posture or the positioning of the body or one of its extremities in a way that may be opposed by the force of gravity (see Figure 12-25, p. 366). We do this unconsciously, even though these receptors do contribute to conscious proprioception.
Golgi tendon organs, often called simply tendon organs, like muscle spindles, are proprioceptors. They are located at the point of junction between muscle tissue and tendon. Each Golgi tendon organ consists of dendrites (Golgi tendon receptors) of afferent (sensory) nerves called type Ib nerve fibers, which are associated with bundles of collagen fibers from the tendon and surrounded by a capsule. These sensory organs act in a way opposite that of muscle spindles. Golgi tendon organs are stimulated by excessive stretch of a tendon—as when pulling too great a load for the muscle to bear safely. When the Golgi tendon organ is activated, the skeletal muscle relaxes. This response, called a Golgi tendon reflex, protects muscles from tearing internally or pulling away from their tendinous points of attachment to bone because of excessive contractile force.
Table 17-1 summarizes the different types of somatic sense receptors, their locations, and their functions.
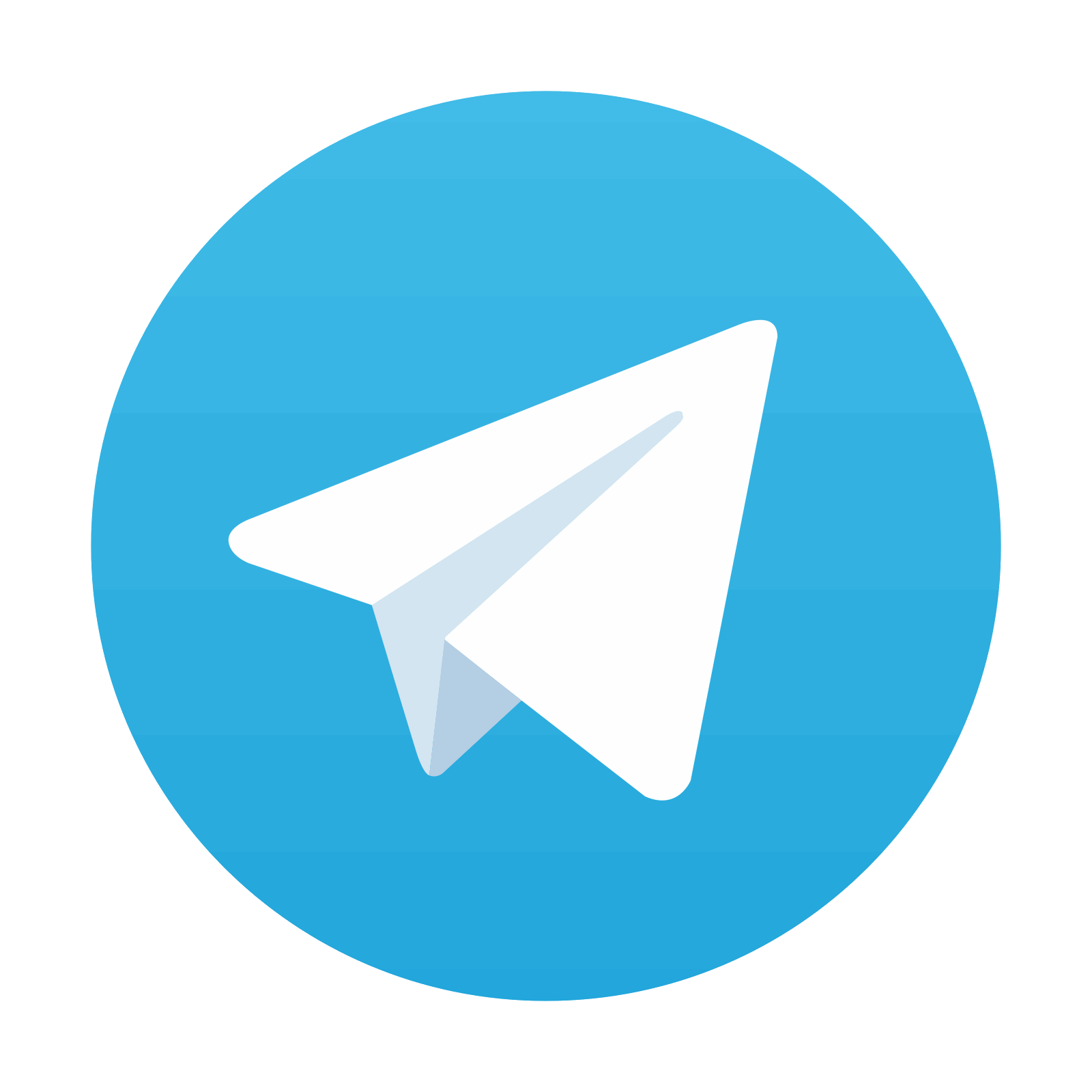
Stay updated, free articles. Join our Telegram channel

Full access? Get Clinical Tree
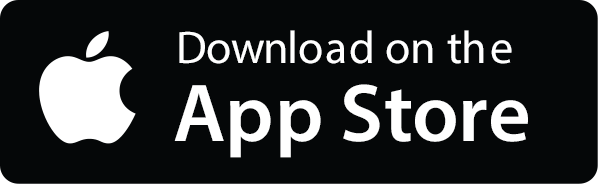
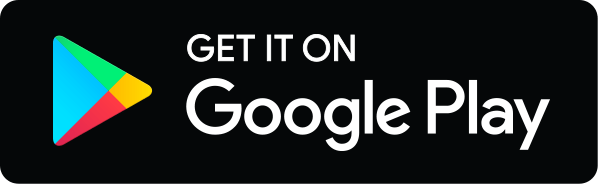