Fig. 10.1
The schematic diagram to show the PONT model. The lines represent the axons in the ON and the circles indicate the cell bodies of RGCs: the purple structures locate in the dorsal parts of the retina and ON and the blue structures locate in the ventral parts of the retina and ON. The red line shows the cut site after PONT in the dorsal ON. a The cell bodies and axons of RGCs are intact in normal eye and connect with each other. b After PONT surgery, in addition to the degeneration of the cell bodies of RGCs whose axons are transected, the cell bodies whose axons are reserved after PONT also degenerate
10.2.3 Mechanisms of Secondary Degeneration After PONT
The PONT model has been conducted in three species of rats, including Wistar rats (Levkovitch-Verbin et al. 2003), PVG hooded rats (Fitzgerald et al. 2009a), and Sprague Dawley (SD) rats (Li et al. 2013). After partial injury in the dorsal ON (about one quarter in Wistar rats and SD rats or one third in PVG hooded rats), the axons in the central and the ventral ON would be vulnerable to secondary degeneration (Levkovitch-Verbin et al. 2003; Fitzgerald et al. 2009b, 2010b; Li et al. 2013). In the retinas, the localization of primary and secondary degeneration of the RGC bodies should be based on the topography of the transected axons in the three different strains (Levkovitch-Verbin et al. 2003; Fitzgerald et al. 2009b; Li et al. 2013). The mechanisms underlying both primary and secondary degeneration after PONT were studied simultaneously in some experiments. But the scope of this chapter is mainly limited to the mechanisms of secondary degeneration after PONT. Several mechanisms were assumed to be related with secondary degeneration after PONT, including:
10.2.3.1 Apoptosis, Necrosis, and Autophagy
In the retinas, both apoptosis and necrosis contributed to secondary degeneration of the RGC bodies after PONT (Levkovitch-Verbin et al. 2010; Fitzgerald et al. 2009b). In the ON, mitochondrial autophagic profiles were observed in the areas vulnerable to secondary degeneration (Cummins et al. 2013).
10.2.3.2 Oxidative Stress
Oxidative stress, indicated by increased expression of manganese superoxide dismutase (MnSOD) and decreased catalase activity, occurred as early as 5 min after injury in the ventral ON (area vulnerable to secondary degeneration; Fitzgerald et al. 2010a; Wells et al. 2012). In the retinas, the oxidative stress indicated by increasing expression of MnSOD began at 24 h after PONT in secondary degeneration both in PVG hooded rats and SD rats (Fitzgerald et al. 2010a; Li et al. 2013). Therefore, oxidative stress was involved in the secondary degeneration of both axons and RGC bodies after PONT.
10.2.3.3 Calcium Overload
The result after PONT indicated a redistribution of calcium from internal stores to the cytosol (Wells et al. 2012). It is known that calcium accumulation can lead to the overproduction of reactive oxygen species (ROS) in mitochondria (Lewen et al. 2000; Begemann et al. 2010). In addition, the immunoreactivity of glutamate receptor subunit GluR1, a subunit of functional α-amino-3-hydroxy-5-methyl-4-isoxazolepropionic acid (AMPA) receptors, was increased rapidly in the site of the ON vulnerable to secondary degeneration. Increased plasma membrane expression of GluR1 in astrocytes, resulting in increased calcium flux into and across the linked astrocytic network, is thought to lead to the early spread of oxidative stress in neurons (Fitzgerald et al. 2009a).
10.2.3.4 Mitochondrial Change
10.2.3.5 C-jun
C-jun was shown to be involved in secondary degeneration after PONT. Western blotting showed that the expression of phospho-c-jun (p-c-jun) increased in the inferior retinas (Li et al. 2013). Immunohistochemical (IHC) staining showed that the number of p-c-jun or c-jun positive cells increased significantly from 3 days after PONT in secondary degeneration (Fitzgerald et al. 2010a; Vander and Levkovitch-Verbin 2012).
10.2.3.6 Water Channel Change
Aquaporin 4 (AQP4) is the main water channel of the mammalian nervous system and is indicated to colocalize with the inward potassium (K) channel Kir 4.1, as a water–potassium transport complex. AQP4 immunointensity in glial fibrillary acidic protein (GFAP)-positive astrocytes increased following PONT (Wells et al. 2012). Although no change in Kir 4.1 immunoreactivity or K ions was observed, it is possible that the change in the flux of ions in response to AQP4-mediated astrocytic swelling/hypertrophy results in the spread of altered ionic balances through the astrocytic syncytium and increased secondary degeneration.
10.2.3.7 Glial Cells
Three days after PONT, the numbers of microglia/macrophages increased significantly in the ventral ON (Fitzgerald et al. 2010a). Astrocytes became hypertrophic after PONT and the immunoreactivity of oxidative stress markers (MnSOD and advanced glycation end product carboxymethyl lysine) increased in the astrocytes of the ventral ON after PONT (Fitzgerald et al. 2010a; Wells et al. 2012). Calcium overload is known to contribute to increased oxidative stress. As mentioned above, the ion channel AMPA receptor subunit GluR1 immunointensity and water channel AQP4 immunointensity in astrocytes was significantly increased in the ventral ON 3 and 24 h following PONT, respectively. Change in AQP4 immunoreactivity may indicate its involvement in altered water balance and flux of ions following PONT. These results indicated astrocytes in the spread of calcium and oxidative stress during secondary degeneration (Fitzgerald et al. 2010a; Wells et al. 2012). Swelling in myelinated axons, but not in unmyelinated axons was detected after PONT in PVG hooded rats. It implied that the dysfunction of oligodendroglia contributed to axon swelling and damaged axonal transport (Payne et al. 2011). It is known that oligodendrocytes are vulnerable to glutamate excitotoxicity, which may be released from the neural tissue after primary injury. But the occurrence of excitotoxicity after PONT has not been investigated yet and the number of oligodendrocytes remains stable after PONT. Therefore, to understand the role of oligodendrocytes in secondary degeneration, study about excitotoxity should be conducted.
10.2.4 Secondary Degeneration Beyond Ganglion Cell Layer After PONT
What should be noticed is that other retinal layers were also affected after PONT in addition to ganglion cell layer. We investigated the activity of other retinal layers after PONT by electroretinogram (ERG), which is a functional recording of the retinal electrical signals in response to a flash of light received at the retina. The multifocal electroretinogram (mfERG) is an advanced ERG technique to examine the retinal response of multiple loci within a short period of time, which is similar to multiple measurements of localized ERGs. The mfERG measurement provides topographical responses of the retina (Sutter and Tran 1992), which can illustrate the retinal changes caused by glaucomatous damage (Chan and Brown 1999, 2000; Chu et al. 2006, 2007). Previous studies showed that the P1 component of mfERG originated from the outer retinas and maybe ON-bipolar cells in porcine and rhesus monkey (Hood et al. 2002; Ng et al. 2008). Our results showed that the P1 component originated from the outer retina because its amplitude remained unchanged after inhibiting the inner retinal activity by tetrodotoxin (TTX) + N-methyl-D-aspartic acid (NMDA). However, its amplitude reduced across the whole retina after PONT in our study indicated that outer retina was also affected. The damage after PONT is assumed to be limited to the RGC layer, but not other layers. The overall reduction of the P1 component indicates that the secondary degeneration could adversely influence the retinal layers beyond RGC level (Chu et al. 2013). We also noticed, after PONT, the decrease of the mfERG photopic negative response (PhNR) component, which is an electroretinal response related to the inner retina as well as RGC. The PhNR reduction was originally assumed to be found at the site with ON transection, but the general diminish of PhNR of the whole retina illustrates that the secondary degeneration also damages the inner retina not related to the part of ON without transection. This kind of transsynaptic degeneration has been found in glaucoma patients and glaucoma models. For example, the decrease of cone opsin messenger RNA (mRNA) occurred in glaucomatous patients and monkey models (Pelzel et al. 2006), swelling and patchy loss of cone photoreceptors in the macular region (Nork et al. 2000; Calkins 2012). In DBA mice, the best-characterized chronic glaucoma model, detection with retinal histology showed the attenuation of scotopic a- and b-wave amplitude concurrent with thinning of the inner plexiform layer and of the outer retina layer, respectively (Bayer et al. 2001). In C57 mice, the numbers of GABAergic types of amacrine cells decreased by about the same percentages to RGCs (Moon et al. 2005). These data indicated the possible degeneration of photoreceptors and amacrine cells in glaucoma. The mechanisms of this transsynaptic degeneration were not clear, but the degeneration of other layers than the ganglion cell layer should be checked when assessing the effects of a neuroprotective agent.
10.3 L. barbarum
10.3.1 A Brief Introduction of L. barbarum
In Chinese medicine, L. barbarum has been used as an “upper class herb” for many years, and it is believed to be good for the vision sight, nourishing the “kidney” and protecting the “liver” (Junlin and Aicheng 2002). It can be eaten directly, and also can be used for making tea, tonic soup, and wine in daily life. L. barbarum is made up of many components, for example, polysaccharides (Chang and So 2008; Ho et al. 2009), taurine (Song et al. 2011), betaine (Xie et al. 2001; Lee et al. 2004), zeaxanthin (Kim et al. 2002), beta-carotene (Inbaraj et al. 2008), beta-sitosterol (Xie et al. 2001), flavonoids (Lee et al. 2004), vitamins (Zhang et al. 2011), amino acids (Wang et al. 2012), fatty acids (Honglin et al. 2009), and trace minerals (Yang et al. 2012b).
The biological effects of L. barbarum have been reported in different animal models. These studies showed that it had potential benefits against overweight (Amagase and Nance 2011), liver injury (Cui et al. 2011; Xiao et al. 2012), cardiovascular diseases (Xin et al. 2011), inflammatory diseases (Tang et al. 2011), and cancer (Tang et al. 2011; Zhu and Zhang 2012). It could also modulate immunity (Vidal et al. 2012) and sex behavior (Lau et al. 2012). In addition, L. barbarum has neuroprotective roles (Yu et al. 2005, 2006; Ho et al. 2007; Chang and So 2008; Ho et al. 2010; Li et al. 2011; Mi et al. 2012b; Chu et al. 2013; Li et al. 2013; He et al. 2014).
10.3.2 The Neuroprotective Effects of L. barbarum
L. barbarum is also known as wolfberry or Gouqizi. The neuroprotective effects of L. barbarum have been demonstrated both in vitro and in vivo. L. barbarum polysaccharides (LBP) could protect primary cultured hippocampal neurons of neonatal rats from the damage induced by oxygen–glucose deprivation and reperfusion (Rui et al. 2012). In another study, it elucidated the neuroprotective effects of wolfberry against homocysteine-induced neuronal damage (Ho et al. 2010). Glutamate excitotoxicity takes part in many neurodegenerative diseases. The experiments from Chang’s group showed that L. barbarum could inhibit glutamate neurotoxicity in primary cultures of cortical neurons (Ho et al. 2009). The fruit of L. barbarum also elicited an effective protection for neurons against ß-amyloid peptides-induced apoptosis by reducing the activity of both caspase-3 and -2 (Ho et al. 2007). In vivo, research indicated that L. barbarum extracts protected the brain from blood-brain barrier disruption and cerebral edema in experimental stroke (Yang et al. 2012a). L. barbarum also showed neuroprotective effects on eyes (Chan et al. 2007; Li et al. 2011; Mi et al. 2012b; Chu et al. 2013; Li et al. 2013; He et al. 2014).
10.3.3 The Neuroprotective Effects of L. barbarum on Eyes
L. barbarum has also been studied extensively in eye diseases including diabetic retinopathy, ischemia model, and glaucoma. L. barbarum extract enhanced cell viability of a spontaneously arising retinal pigment epithelia line (ARPE-19, a model of diabetic retinopathy) exposed to high glucose injury. This cytoprotective effect was achieved by the reduction of high-glucose-induced apoptosis and downregulation of caspase-3 protein expression (Song et al. 2012). The results from cell culture study have shown that LBP could increase the survival of RGCs from neonatal SD rats (Yang et al. 2011). In animal studies, L. barbarum could improve the function of retinas in rats with diabetic retinopathy evaluated by using electroretinogram (Hu et al. 2012). In retinal ischemia/reperfusion model, LBP attenuated neuronal damage, blood-retinal barrier disruption, and oxidative stress (Li et al. 2011). L. barbarum could rescue photoreceptors of rd1 mice (Miranda et al. 2010). The human study showed that L. barbarum could reduce hypopigmentation and soft drusen accumulation in the macula of elderly subjects (Bucheli et al. 2011b). LBP could decrease the expression of endothelin-1 and modulate the expression of its receptors in a rat OH model (Mi et al. 2012a). It could increase the expression of crystallins and modulate microglia and then protected RGCs in rat hypertension models (Chan et al. 2007; Chiu et al. 2009, 2010).
The protective mechanisms of L. barbarum involve antioxidation (Li 2007; Cheng and Kong 2011; Li et al. 2011; Shan et al. 2011; Xiao et al. 2012), anti-excitotoxicity (Ho et al. 2009), anti-inflammation (Wu et al. 2011; Xiao et al. 2012), and anti-apoptosis (Ho et al. 2010; Li et al. 2011; Song et al. 2012).
10.3.4 The Effects of L. barbarum on Secondary Degeneration of RGCs After PONT
In our previous study using the PONT model of SD rats, it was shown that LBP could reduce secondary degeneration but not primary degeneration of the cell bodies of the RGCs after PONT (Li et al. 2013). This protective effect might be related with the antioxidant effect of LBP and the inhibition of the JNK pathway (Li et al. 2013). In addition to the primary and secondary degeneration of RGCs, the mfERG study showed that outer retinas (photoreceptors and bipolar cells) could also be affected subsequently after PONT, and L. barbarum could reverse the functional deterioration caused by primary and secondary degeneration of the outer and inner retina (Chu et al. 2013). Therefore, L. barbarum could delay secondary degeneration caused by PONT including RGCs and outer retinas. The results from our group are summarized in Fig. 10.2.


Fig. 10.2
The summary of the results from our group about the neuroprotection of LBP on secondary degeneration of retinas after PONT. After PONT, secondary degeneration of RGCs in the inferior retinas is caused by apoptosis attributed to the activation of the JNK pathway, and oxidative stress occurs. The retinal function is damaged by the degeneration of RGCs and secondary functional injury of outer retinas. The administration of LBP can reverse these changes both in structure and function
10.3.5 The Possible Mechanisms Involved in the Neuroprotection of L. barbarum in Secondary Degeneration
10.3.5.1 Antioxidant Property
MnSOD is an antioxidant enzyme by converting toxic superoxide into hydrogen peroxide and diatomic oxygen. In our study, LBP could increase the production of MnSOD in the retinas after PONT (Li et al. 2013). The study from He et al. showed that LBP could also activate the transcription factor nuclear factor erythroid 2-related factor (Nrf2)/Heme oxygenase-1(HO-1) pathway to exert the antioxidant role in the retina after ischemia/reperfusion damage (He et al. 2014). Both RGCs and amacrine cells lost after ischemia/reperfusion and the degeneration of amacrine cells also occurred in glaucoma (Moon et al. 2005). Therefore, LBP may protect the amacrince cells from secondary degeneration via the antioxidant property.
10.3.5.2 Inhibition of Apoptosis
Apoptosis is involved in the secondary degeneration of RGCs after PONT (Levkovitch-Verbin et al. 2010; Li et al. 2013). JNKs can bind and phosphorylate c-jun. Three kinds of JNKs, including JNK1, JNK2, and JNK3, are all involved in apoptosis (Yang et al. 1997; Tournier et al. 2000). JNK3 is found mainly in the neural tissues. LBP could reduce the phosphorylation of JNK1 in rat cortical neurons, which were induced toxicity by homocysteine and glutamate (Ho et al. 2009, 2010). Our study showed that LBP could decrease the phosphorylation of JNK3 and c-jun after PONT (Li et al. 2013). Therefore, LBP may inhibit apoptosis via the inhibition of the JNK pathway.
10.3.5.3 Immunomodulatory Effects
10.3.5.4 Preserving Synapses
Our study has shown the transsynaptic cell degeneration in retinas after PONT by multifocal ERG testing (Chu et al. 2013). In our group, LBP restored the decreased spine density and the reduced expression of postsynaptic density protein 95 (PSD-95) in the hippocampus (Zhang et al. 2012). PSD-95 exists in the postsynaptic component of synapses and is important for the maintaining of synapses. Therefore, it is possible that LBP can preserve the structure and function of synapses after PONT, which needs further investigation in the future.
10.3.5.5 Modulating Autophagy
Autophagy is involved in secondary degeneration of axons in the ON (Cummins et al. 2013). LBP could inhibit autophagy after transection of sciatic nerve in rats (Fan et al. 2010). Although the effects of LBP on autophagy have not been investigated after PONT, it is a possible mechanism of the neuroprotection of LBP.
10.3.5.6 Modulating Signal Processing
The LBP was found to restore and to enhance the mfERG responses of both outer and inner retina (Chu et al. 2013). This phenomenon was not observed in the eyes without PONT under the supplement of LBP. The protective effect of LBP is believed to be activated in the presence of PONT or other insults. Without any delay of the mfERG components, the recovery of the mfERG may be caused by changing the electrical characteristics of the retinal cells, altering the blood flow and improving the synaptic transmission. Most of the RGCs in the superior retinas degenerated and they are not likely to involve in the restoring of the PhNR after LBP supplement. The influence to the electro-retinal activity may be contributed by other cells in the inner retina, for example, amacrine cells (Kielczewski et al. 2005). Therefore, it seems that after PONT the LBP activate the amacrine cells, which provide an effect of compensation reflex to rescue the signal disturbance from inner retina (or RGCs) by enhancing the outer retinal responses.
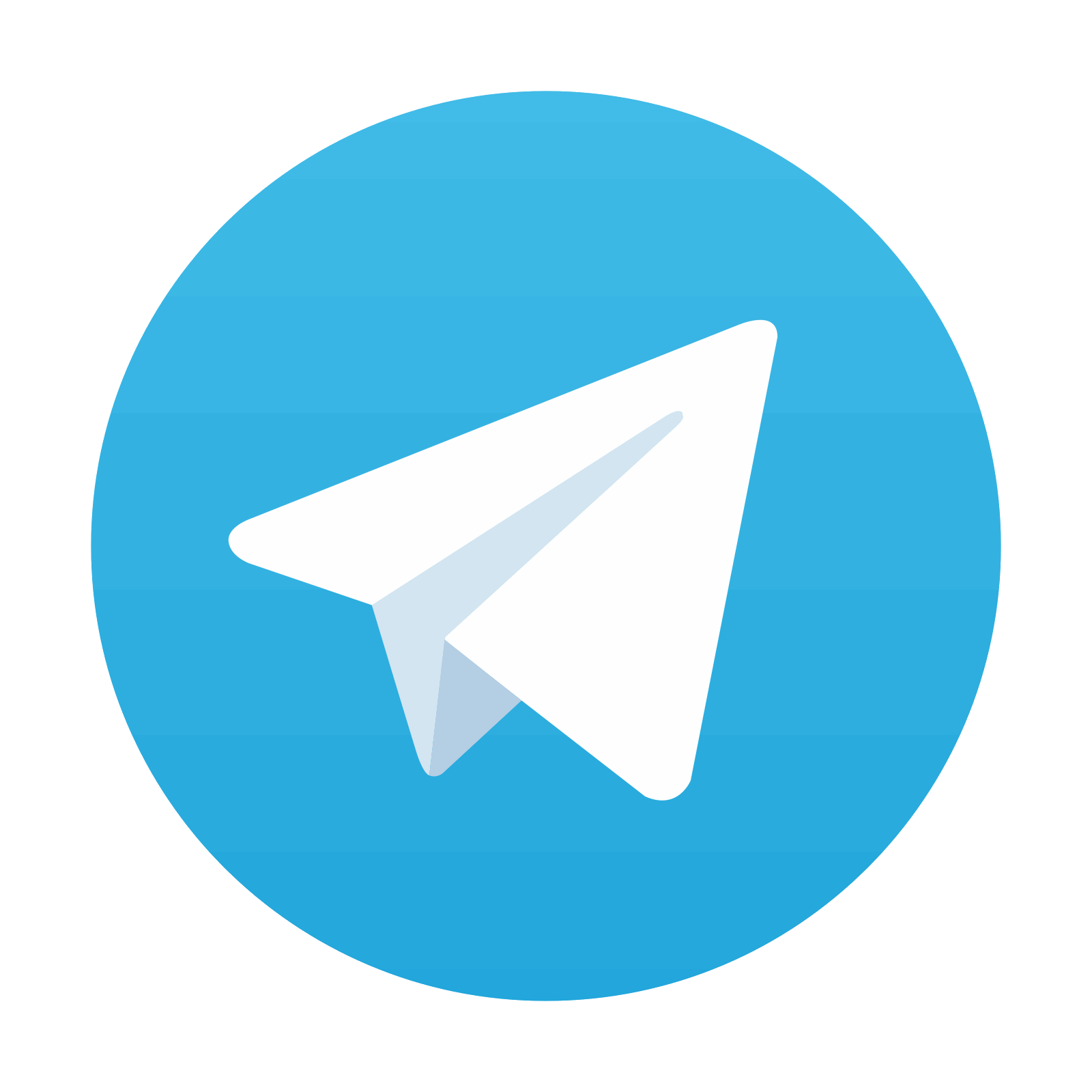
Stay updated, free articles. Join our Telegram channel

Full access? Get Clinical Tree
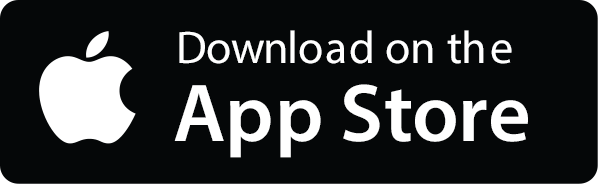
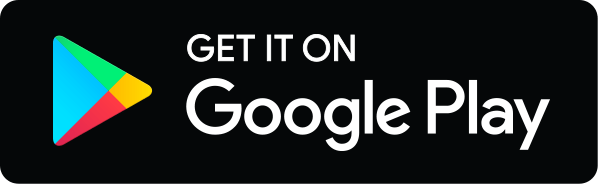