6
Proteins: Myoglobin & Hemoglobin
Peter J. Kennelly, PhD & Victor W. Rodwell, PhD
OBJECTIVES
After studying this chapter, you should be able to:
Describe the most important structural similarities and differences between myoglobin and hemoglobin.
Sketch binding curves for the oxygenation of myoglobin and hemoglobin.
Identify the covalent linkages and other close associations between heme and globin in oxymyoglobin and oxyhemoglobin.
Explain why the physiologic function of hemoglobin requires that its O2-binding curve be sigmoidal rather than hyperbolic.
Explain the role of a hindered environment on the ability of hemoglobin to bind carbon monoxide.
Define P50 and indicate its significance in oxygen transport and delivery.
Describe the structural and conformational changes in hemoglobin that accompany its oxygenation and subsequent deoxygenation.
Explain the role of 2,3-bisphosphoglycerate (BPG) in oxygen binding and delivery.
Outline the role of hemoglobin in CO2 and proton transport, and describe accompanying changes in the pKa of the relevant imidazolium group.
Describe the structural consequences to HbS of lowering pO2.
Identify the metabolic defect that occurs as a consequence of α- and -β thalassemias.
BIOMEDICAL IMPORTANCE
The heme proteins myoglobin and hemoglobin maintain a supply of oxygen essential for oxidative metabolism. Myoglobin, a monomeric protein of red muscle, stores oxygen as a reserve against oxygen deprivation. Hemoglobin, a tetrameric protein of erythrocytes, transports O2 to the tissues and returns CO2 and protons to the lungs. Cyanide and carbon monoxide kill because they disrupt the physiologic function of the heme proteins cytochrome oxidase and hemoglobin, respectively. The secondary-tertiary structure of the subunits of hemoglobin resembles myoglobin. However, the tetrameric structure of hemoglobin permits cooperative interactions that are central to its function. For example, 2,3-BPG promotes the efficient release of O2 by stabilizing the quaternary structure of deoxyhemoglobin. Hemoglobin and myoglobin illustrate both protein structure–function relationships and the molecular basis of genetic diseases such as sickle cell disease and the thalassemias.
HEME & FERROUS IRON CONFER THE ABILITY TO STORE & TO TRANSPORT OXYGEN
Myoglobin and hemoglobin contain heme, a cyclic tetrapyrrole consisting of four molecules of pyrrole linked by methyne bridges. This planar network of conjugated double bonds absorbs visible light and colors heme deep red. The substituents at the β-positions of heme are methyl (M), vinyl (V), and propionate (Pr) groups arranged in the order M, V, M, V, M, Pr, Pr, M (Figure 6–1). The atom of ferrous iron (Fe2+) resides at the center of the planar tetrapyrrole. Other proteins with metal-containing tetrapyrrole prosthetic groups include the cytochromes (Fe and Cu) and chlorophyll (Mg) (see Chapter 31). Oxidation and reduction of the Fe and Cu atoms of cytochromes are essential to their biologic function as carriers of electrons. By contrast, oxidation of the Fe2+ of myoglobin or hemoglobin to Fe3+ destroys their biologic activity.
FIGURE 6–1 Heme. The pyrrole rings and methyne bridge carbons are coplanar, and the iron atom (Fe2+) resides in almost the same plane. The fifth and sixth coordination positions of Fe2+ are directly perpendicular to—and directly above and below—the plane of the heme ring. Observe the nature of the methyl (blue), vinyl (green), and propionate (orange) substituent groups on the β carbons of the pyrrole rings, the central iron atom (red), and the location of the polar side of the heme ring (at about 7 o’clock) that faces the surface of the myoglobin molecule.
Myoglobin Is Rich in α Helix
Oxygen stored in red muscle myoglobin is released during O2 deprivation (eg, severe exercise) for use in muscle mitochondria for aerobic synthesis of ATP (see Chapter 13). A 153-aminoacyl residue polypeptide (MW 17,000), myoglobin folds into a compact shape that measures 4.5 × 3.5 × 2.5 nm (Figure 6–2). An unusually high proportion, about 75%, of the residues are present in eight right-handed 7–20 residue α helices. Starting at the amino terminal, these are termed helices A–H. Typical of globular proteins, the surface of myoglobin is rich in amino acids bearing polar and potentially charged side chains, while—with only two exceptions—the interior contains only residues such as Leu, Val, Phe, and Met that possess nonpolar R groups. The exceptions are His E7 and His F8, the seventh and eighth residues in helices E and F, which lie close to the heme iron, where they function in O2 binding.
FIGURE 6–2 Three-dimensional structure of myoglobin. Shown is a ribbon diagram tracing the polypeptide backbone of myoglobin. The color of the polypeptide chain is graded along the visible spectrum from blue (N-terminal) to tan (C-terminal). The heme prosthetic group is red. The α-helical regions are designated A through H. The distal (E7) and proximal (F8) histidine residues are highlighted in blue and orange, respectively. Note how the polar propionate substituents (Pr) project out of the heme toward solvent. (Adapted from Protein Data Bank ID no. 1a6n.)
Histidines F8 & E7 Perform Unique Roles in Oxygen Binding
The heme of myoglobin lies in a crevice between helices E and F oriented with its polar propionate groups facing the surface of the globin (Figure 6–2). The remainder resides in the non-polar interior. The fifth coordination position of the iron is occupied by a nitrogen from the imidazole ring of the proximal histidine, His F8. The distal histidine, His E7, lies on the side of the heme ring opposite to His F8.
The Iron Moves Toward the Plane of the Heme when Oxygen Is Bound
The iron of unoxygenated myoglobin lies 0.03 nm (0.3 Å) outside the plane of the heme ring, toward His F8. The heme therefore “puckers” slightly. When O2 occupies the sixth coordination position, the iron moves to within 0.01 nm (0.1 Å) of the plane of the heme ring. Oxygenation of myoglobin thus is accompanied by motion of the iron, of His F8, and of residues linked to His F8.
Apomyoglobin Provides a Hindered Environment for the Heme Iron
When O2 binds to myoglobin, the bond between the first oxygen atom and the Fe2+ is perpendicular to the plane of the heme ring. The bond that links the first and second oxygen atoms lies at an angle of 121° to the plane of the heme, orienting the second oxygen away from the distal histidine (Figure 6–3, left). This permits maximum overlap between the iron and one of the lone pairs of electrons on the sp2 hybridized oxygen atoms, which lie at an angle of roughly 120° to the axis of the O=O double bond (Figure 6–4, left). Isolated heme binds carbon monoxide (CO) 25,000 times more strongly than oxygen. Since CO is present in small quantities in the atmosphere and arises in cells from the catabolism of heme, why is it that CO does not completely displace O2 from heme iron? The accepted explanation is that the apoproteins of myoglobin and hemoglobin create a hindered environment. When CO binds to isolated heme, all the three atoms (Fe, C, and O) lie perpendicular to the plane of the heme. This geometry maximizes the overlap between the lone pair of electrons on the sp hybridized oxygen of the CO molecule and the Fe2+ iron (Figure 6–4, right). However, in myoglobin and hemoglobin the distal histidine sterically precludes this preferred, high-affinity orientation of CO, but not that of O2. Binding at a less favored angle reduces the strength of the heme-CO bond to about 200 times that of the heme-O2 bond (Figure 6–3, right) at which level the great excess of O2 over CO normally present dominates. Nevertheless, about 1% of myoglobin typically is present combined with CO.
FIGURE 6–3 Angles for bonding of oxygen and carbon monoxide (CO) to the heme iron of myoglobin. The distal E7 histidine hinders bonding of CO at the preferred (90°) angle to the plane of the heme ring.
FIGURE 6–4 Orientation of the lone pairs of electrons relative to the and
bonds of oxygen and carbon monoxide. In molecular oxygen, formation of the double bond between the two oxygen atoms is facilitated by the adoption of an sp2 hybridization state by the valence electron of each oxygen atom. As a consequence, the two atoms of the oxygen molecule and each lone pair of electrons are coplanar and separated by an angle of roughly 120° (left). By contrast, the two atoms of carbon monoxide are joined by a triple bond, which requires that the carbon and oxygen atoms adopt an sp hybridization state. In this state the lone pairs of electrons and triple bonds are arranged in a linear fashion, where they are separated by an angle of 180° (right).
THE OXYGEN DISSOCIATION CURVES FOR MYOGLOBIN & HEMOGLOBIN SUIT THEIR PHYSIOLOGIC ROLES
Why is myoglobin unsuitable as an O2 transport protein but well suited for O2 storage? The relationship between the concentration, or partial pressure, of O2 (Po2) and the quantity of O2 bound is expressed as an O2 saturation isotherm (Figure 6–5). The oxygen-binding curve for myoglobin is hyperbolic. Myoglobin therefore loads O2 readily at the Po2 of the lung capillary bed (100 mm Hg). However, since myoglobin releases only a small fraction of its bound O2 at the Po2 values typically encountered in active muscle (20 mm Hg) or other tissues (40 mm Hg), it represents an ineffective vehicle for delivery of O2. When strenuous exercise lowers the Po2 of muscle tissue to about 5 mm Hg, myoglobin releases O2 for mitochondrial synthesis of ATP, permitting continued muscular activity.
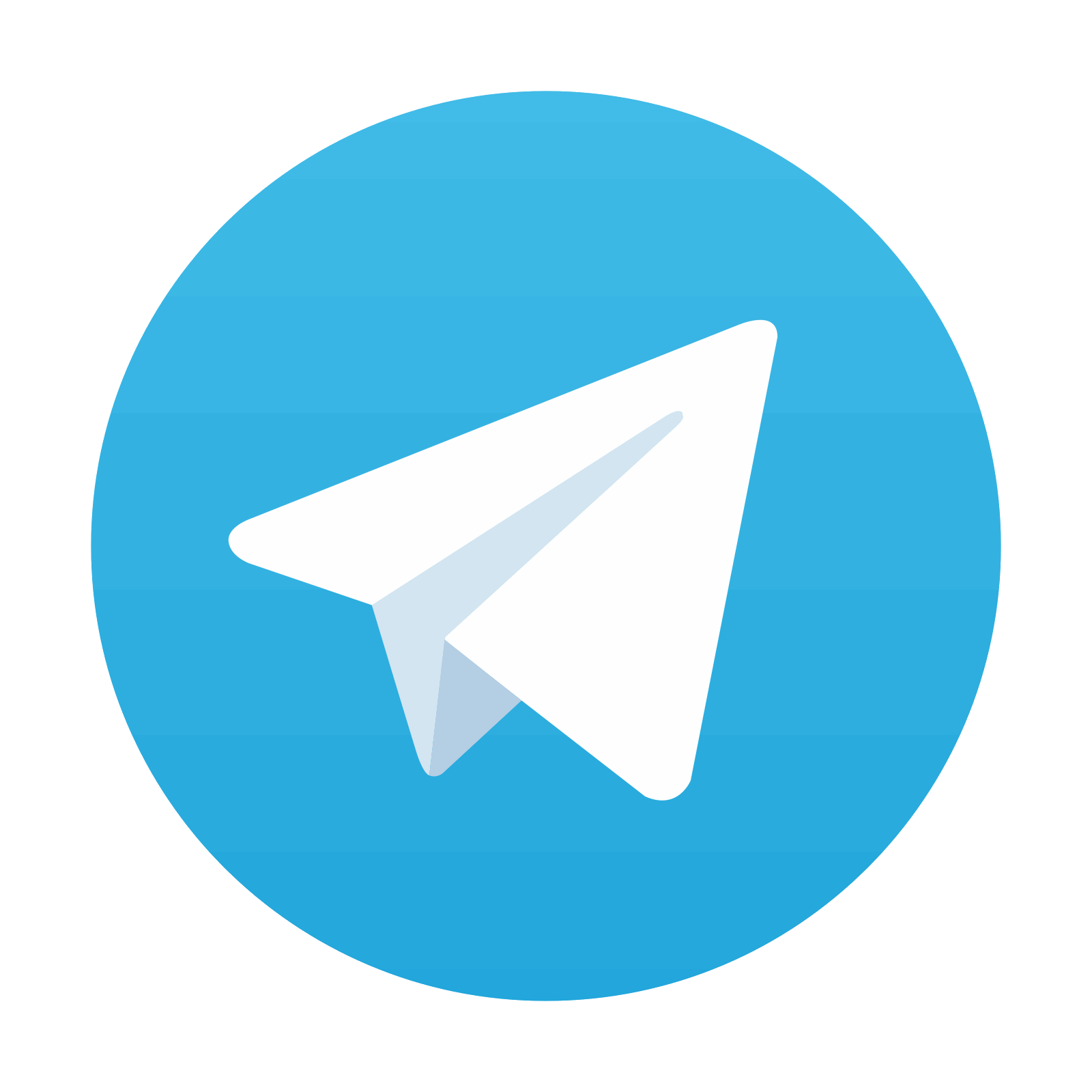
Stay updated, free articles. Join our Telegram channel

Full access? Get Clinical Tree
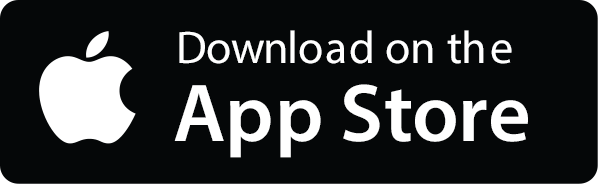
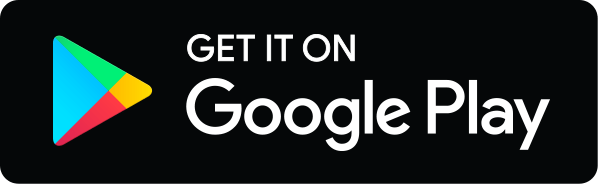