Proteins in Plasma and Urine
PLASMA PROTEINS
Plasma albumin and total protein are often included as part of a biochemistry department’s assay profile, and other more specialized plasma proteins such as C-reactive protein (CRP) may also be measured in certain circumstances. There are also a number of clinical conditions that involve abnormalities of plasma and urinary proteins, some of which are discussed in this chapter.
Metabolism of proteins
The amount of protein in the vascular compartment depends on the balance between the rates of synthesis and catabolism or loss. However, also important is the relative distribution between the intravascular and extravascular compartments, as the concentration depends on the relative amounts of protein and water in the vascular compartment. Therefore, abnormal concentrations do not necessarily reflect defects in protein metabolism.
Protein synthesis
Hepatocytes synthesize many plasma proteins; those of the complement system are also made by macrophages. Immunoglobulins are mainly derived from the B lymphocytes of the immune system.
Protein catabolism and loss
Most plasma proteins are taken up by pinocytosis into capillary endothelial cells or mononuclear phagocytes, where they are catabolized. Small-molecular-weight proteins are lost passively through the renal glomeruli and intestinal wall. Some are reabsorbed, either directly by renal tubular cells or after digestion in the intestinal lumen; others are catabolized by renal tubular cells.
Functions of plasma proteins
Peptide hormones and blood clotting factors contribute quantitatively relatively small, but physiologically important, amounts of plasma protein. Some plasma proteins originate from cell breakdown.
Control of extracellular fluid distribution
The distribution of water between the intravascular and extravascular compartments is influenced by the colloid osmotic effect of plasma proteins, predominantly albumin (see Chapter 2).
Transport
Albumin and specific binding proteins transport hormones, vitamins, lipids, bilirubin, calcium, trace metals and drugs. Combination with protein allows poorly water-soluble substances to be transported in plasma. The protein-bound fraction of many of these substances is physiologically inactive, unlike the unbound fraction.
Inflammatory response and control of infection
The immunoglobulins and the complement proteins form part of the immune system and the latter, together with a group of proteins known as acutephase reactants, for example CRP, are involved in the inflammatory response.
Methods of assessing plasma proteins
Plasma proteins may be expressed either as concentrations (for example g/L) or as activities of those proteins that have defined functions, such as clotting times for prothrombin or enzyme activity (see Chapter 18). The distinction is important when abnormal forms of protein are present at normal concentration but with impaired function, such as C1 inhibitor (see Classic pathway). Laboratories are able to measure total serum protein concentration, assay specific proteins and also identity and quantify serum proteins by serum electrophoresis.
Total plasma proteins
Acute changes in proteins are often due to loss from, or gain by, the vascular compartment of protein-free fluid, rather than of protein. Only marked changes of major
constituents, such as albumin and immunoglobulins, are likely to alter total protein concentrations significantly. The acute-phase response can result in rapid albumin extravasation and thus hypoalbuminaemia.
constituents, such as albumin and immunoglobulins, are likely to alter total protein concentrations significantly. The acute-phase response can result in rapid albumin extravasation and thus hypoalbuminaemia.
Plasma total protein concentrations may be misleading for other reasons. They may be normal in the presence of quite marked changes in the constituent proteins. For example, a fall in plasma albumin concentration may be approximately balanced by a rise in immunoglobulin concentrations. Also, most individual proteins apart from albumin contribute little to the total protein concentration; therefore, quite a large percentage change in the concentration of one may not cause a detectable change in the total protein concentration.
Raised plasma total protein concentrations may be due to loss of protein-free fluid, or excessive stasis during venepuncture, or a major increase in the concentration of one or more of the immunoglobulins, including paraproteins. The difference between serum total protein and albumin concentration is termed the serum globulin concentration. Low plasma total protein concentrations may be due to dilution, for example if blood is taken near the site of an intravenous saline infusion, hypoalbuminaemia or severe immunoglobulin deficiency.
Qualitative methods for studying plasma and urinary proteins
Electrophoresis
Electrophoresis is a technique that separates compounds such as proteins according to their different electrical charges. It is usually performed by applying a small amount of serum to a strip of cellulose acetate or agarose and passing a current across it for a standard time. In this way, five main groups of proteins – namely albumin and the α1, α2, β and γ globulins – may be distinguished after protein staining and may be visually compared with those in a normal control serum. Each of the globulin fractions contains several proteins (Fig. 19.1).
The following description applies to the normal appearance, in adults, of the principal bands seen after electrophoresis on cellulose acetate:
Albumin, usually a single protein, makes up the most obvious band.
α1-globulins consist almost entirely of α1-antitrypsin and α1-antichymotrypsin.
α2-globulins consist mainly of α2-macroglobulin and haptoglobin.
β-globulins often separate into two; β1 consists mainly of transferrin with a contribution from lowdensity lipoprotein (LDL), and β2 consists of the C3 component of complement.
γ-globulins are immunoglobulins; some immunoglobulins are also found in the α2 and β regions.
If plasma rather than serum is used, fibrinogen appears as a distinct band in the β-γ region. This may make interpretation difficult. Blood should be allowed to clot and serum used, rather than plasma, if electrophoresis is to be performed.
Electrophoretic patterns in disease (Fig. 19.2)
Some abnormal electrophoretic patterns are characteristic of a particular disorder or group of related disorders, while others indicate non-specific pathological processes. For example, the α2 band, which contains haptoglobin, may be reduced if there is in vivo haemolysis and may split into two if in vitro haemolysis has occurred.
Parallel changes in all protein fractions
Reduction may occur in severe undernutrition, sometimes due to malabsorption, unless accompanied by infection and haemodilution. An increase may occur in haemoconcentration.
The acute-phase pattern
Tissue damage usually triggers the sequence of biochemical and cellular events associated with inflammation. The biochemical changes include the stimulation of synthesis of the so-called acute-phase proteins, with a rise in the α1-globulin and α2-globulin fractions. The plasma concentrations of these proteins reflect the activity of the inflammatory response, and their presence is responsible for the rise in the erythrocyte sedimentation rate (ESR) and increased plasma viscosity characteristic of such a response.
Chronic inflammatory states
In chronic inflammation, the usual increase in immunoglobulin synthesis may be visible as a diffuse rise in γ-globulin. If there is an active inflammatory reaction, the increased density in the γ-globulin region is associated with an increase in the α1 and α2 fractions of the acute-phase response.
Cirrhosis of the liver
The changes in the concentrations of plasma proteins in liver disease are discussed in Chapter 17. They are usually ‘non-specific’, but in cirrhosis a characteristic pattern is sometimes seen. Albumin and often α1– globulin concentrations are reduced and the γ-globulin concentration is markedly raised, with apparent fusion or ‘bridging’ of the β and γ bands because of an increase in plasma IgA concentrations.
Nephrotic syndrome
Plasma protein changes depend on the severity of the renal lesion. In early cases, a low plasma albumin concentration may be the only abnormality, but the typical pattern in established cases is reduced albumin, α1-globulin and sometimes γ-globulin bands and an increase in α2-globulin concentration due to a relative or absolute increase in the high-molecular-weight α2-macroglobulin. If the syndrome is due to conditions such as systemic lupus erythematosus (SLE), the γ-globulin concentration may be normal or raised.
α1-Antitrypsin deficiency
Other protein abnormalities
Hypogammaglobulinaemia shows reduced plasma γ-globulins and is discussed in the sections on immunoglobulins.
Multiple myeloma and paraproteinaemia may show a band or bands on the electrophoretic strip and are also discussed later.
Albumin
Albumin, with a molecular weight of about 65 kDa, is synthesized by the liver. It has a normal plasma biological half-life of about 20 days. About 60 per cent in the extracellular fluid is in the interstitial compartment. However, the concentration of albumin in the smaller intravascular compartment is much higher because of the relative impermeability of the blood vessel wall. This concentration gradient across the capillary membrane is important in maintaining plasma volume (see Chapter 2).
There are several inherited abnormalities of albumin synthesis:
the bisalbuminaemias, in which two forms of albumin are present; there are usually no clinical consequences,
analbuminaemia, in which there is deficient synthesis of the protein; clinical consequences are slight, and oedema, although present, is surprisingly mild.
An abnormally high plasma albumin concentration is found only artefactually in a sample taken with prolonged venous stasis or after loss of protein-free fluid.
Causes of hypoalbuminaemia
A low plasma albumin concentration may be due to dilution or to redistribution. True albumin deficiency may be caused by a decreased rate of synthesis, or by an increased rate of catabolism or loss from the body.
Dilutional hypoalbuminaemia
Dilutional hypoalbuminaemia may, as in the case of total protein, result from artefactual changes due to taking blood from the arm into which an infusion is flowing, administration of an excess of protein-free fluid or fluid retention, usually in oedematous states or during late pregnancy.
Redistribution of albumin
Redistribution of albumin from plasma into the interstitial fluid space results from:
Recumbency: plasma albumin concentrations may be 5-10 g/L lower in the recumbent than in the upright position because of the redistribution of fluid.
Increased capillary membrane permeability: this is the usual cause of the rapid fall in plasma concentration found in many circumstances, for example postoperatively and in most illnesses.
The slight fall in plasma albumin concentration found in mild illness may be due to a combination of the above two factors.
Decreased synthesis of albumin
Normally, about 4 per cent of the body albumin is replaced each day. Hepatic impairment, such as cirrhosis, causes hypoalbuminaemia if the rate of synthesis of new amino acids is inadequate to replace those deaminated during metabolism; most of the amino nitrogen is then lost as urinary urea. Hypoalbuminaemia may therefore be due to:
undernutrition, resulting in an inadequate supply of dietary nitrogen,
malabsorption, resulting in impaired absorption of dietary peptides and amino acids,
impairment of synthesis, due to chronic liver dysfunction, for example cirrhosis.
Increased loss of albumin from the body
Because of its relatively low molecular weight, significant amounts of albumin and other low-molecular-weight proteins are lost in conditions associated with increased membrane permeability. The plasma concentration of albumin is higher than that of the other proteins and its loss is therefore more obvious. Albumin loss may occur through:
the skin, because of extensive burns or skin diseases such as psoriasis; a large part of the interstitial fluid is subcutaneous,
the intestinal wall, in protein-losing enteropathy,
the glomeruli, in the nephrotic syndrome.
Increased catabolism of albumin
Catabolism (and therefore nitrogen loss) is increased in many illnesses, including sepsis and hyperthyroidism. This may worsen the hypoalbuminaemia due to other causes.
Consequences of hypoalbuminaemia
The following may occur.
Fluid distribution Albumin is quantitatively the most important protein contributing to the plasma
colloid osmotic pressure. Oedema may occur in severe hypoalbuminaemia (see Chapter 2).
Binding functions Albumin binds calcium, bilirubin and free fatty acids. (See Chapter 6 for a discussion of albumin-adjusted calcium.)
A number of drugs, such as salicylates, penicillin and sulphonamides, may become albumin bound. The albumin-bound fractions are physiologically and pharmacologically inactive. A marked reduction in plasma albumin, by reducing the binding capacity, may increase the plasma free concentration of those substances leading to toxic effects.
Albumin-bound drugs may, if administered together, compete for binding sites, thus increasing free concentrations; an example of this is the simultaneous administration of salicylates and the anticoagulant warfarin, thus potentiating the effect of the latter (see Chapter 25).
The acute-phase response
The body responds to tissue damage and to the presence of infecting organisms or other foreign substances by a complex, inter-related series of cellular and humoral responses. These act together to initiate and control the inflammatory reaction and so to remove damaged tissues and foreign substances. Defence mechanisms can be separated into two main groups.
The inflammatory response: this is non-specific, requires changes in the permeability of membranes and depends on humoral factors such as acutephase reactants, complement or cytokines released in response to inflammation and on a phagocytic cellular response dependent on the function of polymorphonuclear leucocytes and monocytes.
The immune response: in this type of response, cellular or humoral factors act on specific foreign organisms. It depends on the function of lymphocytes, which can be differentiated into:
B-lymphocytes, activated by antigens and by lymphokines, which develop into plasma cells in the bone marrow and synthesize and secrete immunoglobulins.
T-lymphocytes, which, in the thymus, acquire a range of surface antigens that play a vital role in T-cell function after migration to other lymphoid tissue. These include cytotoxicity and delayed hypersensitivity. They also have a regulatory function as either helper or suppressor cells. Each group can be further subdivided using antibodies against the cell surface markers; this is known as cluster differentiation (Fig. 19.3).
The inflammatory response and the ability to kill foreign organisms may be impaired if there is deficiency of either cellular or humoral components.
Acute-phase proteins
The innate or natural immune system, which is phylogenetically older than so-called acquired or adaptive immunity, is a rapid first-line defence mechanism based on non-lymphoid tissue components. The acute-phase response is part of this system and results in pronounced changes in the concentration of plasma proteins in response to a variety of stresses, including infection, tissue injury and inflammation. The concentration of some of these proteins increases (positive acute-phase proteins), such as CRP, fibrinogen and plasma amyloid A, whereas that of others decreases (negative acute-phase proteins), such as albumin and transferrin (Fig. 19.4.)
![]() Figure 19.4 Some proteins of the acute-phase response. A, albumin; CRP, C-reactive protein; HG, haptoglobin; T, transthyretin (previously called pre-albumin). The latter pair can be termed negative acute-phase proteins. Reproduced with kind permission from Candlish JK and Crook M. Notes on Clinical Biochemistry. Singapore: World Scientific Publishing, 1993. |
The non-specific changes in plasma protein concentrations occurring in response to acute or chronic tissue damage are caused by increased protein synthesis in the liver in response to peptide mediators or cytokines.
Cytokines regulate the immune and inflammatory responses. They are classified, depending on their principal biological functions, into groups such as interleukins (ILs), interferons (IFNs) and tumour necrosis factors (TNFs). They activate receptors on adjacent cells (paracrine) or on the same cell (autocrine), but those involved in the inflammatory response, such as IL-1, IL-6 and TNF-α, affect distant (endocrine) organs.
The acute-phase reactants include the following:
Activators of other inflammatory pathways such as CRP, so called because it reacts with the C-polysaccharide of pneumococci. This protein combines with bacterial polysaccharides or phospho lipids released from damaged tissue to become an activator of the complement pathway. Plasma concentrations of CRP rise rapidly in response to acute inflammation and its assay is particularly useful in the early detection of acute infection.
Inhibitors, such as α1-antitrypsin, which control the inflammatory response and so minimize damage to host tissue.
Scavengers, such as haptoglobin, which binds haemoglobin released by local in vivo haemolysis during the inflammatory response.
The concentrations of plasma fibrinogen and of several complement components also increase. An acute-phase response increases blood viscosity and therefore the ESR. The plasma albumin concentration falls and secondary decreases of complement and haptoglobin occur if they are used up excessively. Haptoglobin may be undetectable if much haemoglobin is released from red cells due to intravascular haemolysis or haemorrhage into tissues.
The non-specific nature of the response means that the measurement of individual acute-phase proteins is rarely helpful as an aid to diagnosis. The demonstration of a rise in plasma CRP concentration is more sensitive and specific than measurement of the ESR and is most often raised in bacterial infections and in other acute inflammatory conditions (Fig. 19.4).
Clinically, plasma CRP is used in the following circumstances:
To detect an infection Plasma CRP concentrations are not usually increased in viral infections, but the highest levels are seen in bacterial infections. It has a particular place in diagnosing neonatal infections. In immunosuppressed patients, the usual systemic response to infection such as neutrophil leucocytosis and fever may not be present, and therefore a raised plasma CRP concentration of greater than 10 mg/L suggests infection.
As a guide to the severity of connective tissue disease activity Such conditions include rheumatoid arthritis, juvenile chronic polyarthritis (Still’s disease) and polymyalgia rheumatica. Systemic lupus erythematosus shows less of a CRP rise, but this may be useful to distinguish infection in SLE from an acute disease flare-up (plasma concentrations more than 60 mg/L indicate the former).
In the diagnosis of inflammatory bowel disease Plasma CRP levels more than 50 mg/L are more indicative of Crohn’s disease than ulcerative colitis.
As an indicator of cardiovascular risk Raised plasma CRP concentrations using a high-sensitivity assay are a reputed cardiovascular risk factor (see Chapter 22), as subclinical inflammatory processes have been implicated in atherosclerosis. Plasma levels less than 1 mg/L constitute low cardiovascular risk; patients with levels more than 3 mg/L are at high risk of cardiovascular disease. Owing to problems
of biological variation at these low concentrations repeat determinations are useful.
Procalcitonin is a 14-kDa protein encoded by the Calc1 gene. Blood levels of procalcitonin are increased in systemic inflammation and procalcitonin has been proposed as a possibly better marker of bacterial sepsis than CRP.
Complement
Macrophages and hepatocytes synthesize a group of proteins called the complement system, which is part of the innate immune system. Sequential activation reduces complement plasma concentrations, but in many instances this is compensated for by increased synthesis of acute-phase reactants. The products of activation attract phagocytes to the area of inflammation (chemotaxis), which, by increasing the permeability of the capillary wall to both cellular and chemical components, allows them to reach affected cells. Activation of the complete complement pathway on foreign cell surfaces results in their lysis and, together with immunoglobulins, some of the complement components act as opsonins, which enhance phagocytosis. Because of inhibitors, complement usually circulates in an inactive form.
![]() Figure 19.5 The complement pathway, much simplified. Ag, antigen; Ab, antibody; IgA, immunoglobulin A. |
Clinically, the most important of the complement proteins is C3. Two main pathways are concerned with its activation (Fig. 19.5). Activation of either results in low plasma C3 concentrations.
Classic pathway
In the classic pathway, C3 is activated by the products of a pathway initiated by the activation of C1, usually
by antigen-bound IgG or IgM (immune complexes), by protein within the cell walls of many strains of Staphylococcus aureus, or by CRP. C4 (and C2) are used during the resultant sequence of events, which also activates the alternative pathway via C3; plasma C3 and C4 concentrations fall. Once the formation of immune complexes stops, plasma C3 and C4 concentrations are restored. The enzyme C1q esterase inhibits the serine protease C1 (which is composed of C1q, C1r and C1s).
by antigen-bound IgG or IgM (immune complexes), by protein within the cell walls of many strains of Staphylococcus aureus, or by CRP. C4 (and C2) are used during the resultant sequence of events, which also activates the alternative pathway via C3; plasma C3 and C4 concentrations fall. Once the formation of immune complexes stops, plasma C3 and C4 concentrations are restored. The enzyme C1q esterase inhibits the serine protease C1 (which is composed of C1q, C1r and C1s).
Alternative pathway
In the alternative pathway, which is the more important of the two, C3 is activated by IgA immune complexes, and substances such as lipopolysaccharides and peptidoglycans in the walls of certain bacteria and viruses, factor B and properdin are involved. C3b is formed, which in turn activates more C3. Other products of C3b cause vasodilatation and cell lysis. The cyclical process is self-perpetuating and plasma C3 concentrations fall. If the initial stimulus is removed, inhibitors such as those formed in the acute-phase reaction control the reaction and C3 concentrations return to normal; factors H and I inhibit the enzyme C3 convertase. The alternative pathway is most important in the absence of preformed antibodies.
Recently, a third pathway of C3 activation has been described involving mannose-binding lectin, one of the colectin proteins. After C3 activation, the terminal complement system consists of other activated proteins such as C5-9, collectively known as the membrane attack complex. This is involved in puncturing cell membranes.
In immune-complex disease, such as SLE, circulating immune complexes persist. The products of C3, released by continued activation of the classic pathway, may damage blood vessels, joints and the kidneys. Plasma concentrations of both C3 and C4 are low. Persistently low plasma C3 concentrations may help to distinguish chronic mesangiocapillary glomerulonephritis, with a poor prognosis, from the less serious and self-limiting acute post-streptococcal glomerulonephritis, in which plasma C3 concentrations return to normal within a few months.
Normal cellular response to infection
Two types of phagocytic cells help to combat infection. Polymorphonuclear neutrophil leucocytes migrate into sites of acute inflammation and represent a nonspecific first line of defence against pyogenic bacteria. Mononuclear phagocytes circulate in blood as monocytes. They migrate to sites of chronic inflammation, where they develop into macrophages. Under T-cell control, macrophages are activated and develop a greatly enhanced ability to phagocytose and digest pathogenic organisms.
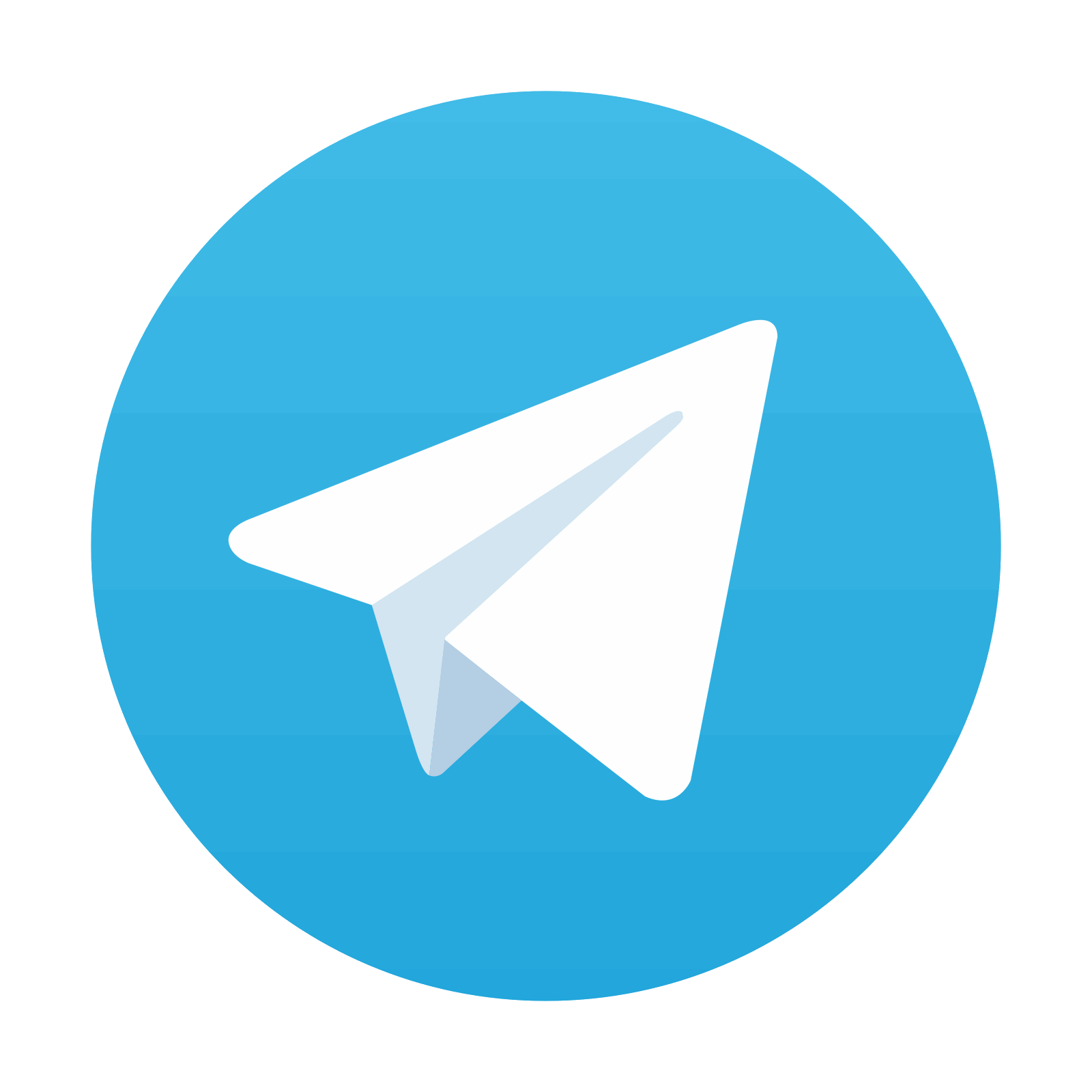
Stay updated, free articles. Join our Telegram channel

Full access? Get Clinical Tree
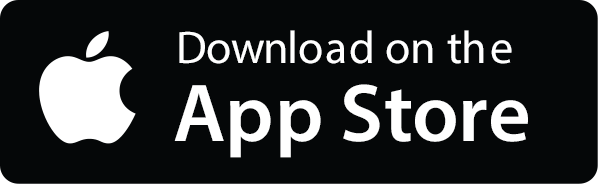
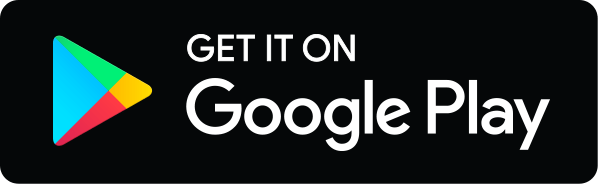