Principles of Controlled Release |
CONTENTS
2.1.2 Mechanisms of Controlling Drug Release
2.1.2.3 Osmotic Pressure Control
2.2 Immediate Release Delivery Systems
2.2.1 Improving Drug Dissolution Rates and Solubility
2.2.2 Improving Disintegration: Fast-Dispersing Products
2.3 Delayed Release Oral Drug Delivery Systems
2.3.1 Drug Release within the Intestine
2.3.2 Mechanisms of Enteric Coating
2.3.3 Drug Release within the Colon
2.4 Sustained Release Delivery Systems
2.4.1 Dissolution-Controlled SR
2.4.1.1 Reservoir Dissolution Systems (Encapsulated Dissolution Systems)
2.4.1.2 Matrix Dissolution Systems
2.4.2.1 Reservoir Diffusion Systems
2.4.2.2 Matrix Diffusion Systems
2.4.3 Gastro-Retentive Sustained Release Systems
2.5 Controlled Release Delivery Systems
2.5.1 Osmotic Pressure–Activated CR Systems
2.5.2 Controlled Release via a Rate-Controlling Membrane
2.5.3 Controlled Release via Matrix Systems
In the prevention and treatment of diseases using pharmacotherapy, the drug of choice is obviously a key factor. However, the site and duration of drug delivery, the rate of drug release, and the pharmacokinetic and side effect profiles of the drug all play pivotal roles in the success of a medical intervention. It is for these reasons that the development of suitable dosage forms or drug delivery systems (DDS; these terms will be used interchangeably in this chapter) is strictly required for any drug, in order to allow the safe, effective, and reliable administration of drugs to patients.
The rate and extent of absorption of a drug into the body, for example, from the gastrointestinal (GI) tract after oral administration, will determine its bioavailability. As described in Chapter 4, the bioavailability of drugs is influenced by physiological factors as well as by the physicochemical characteristics of the drug itself. However, factors associated with the route of administration and properties of the dosage form also have a major influence on the bioavailability of a drug.
An ideal DDS ensures that the active drug is available at the site of action for the appropriate duration of time, while ideally not displaying any physiological effect itself. The DDS should aim to provide drug concentrations at the site of action that are above the minimal effective concentration yet below the maximal tolerated plasma concentration (minimal toxic concentration) (Figure 2.1). Factors that control the drug concentration include the route of administration, the frequency of administration, the metabolism of the drug and its clearance rates, and importantly, the dosage form design itself. Thus, it is not surprising that many different dosage forms have been developed that affect the release and absorption of drugs. Therefore, in clinical practice, it is vital to consider both the drug treatment and the required release properties of the medicine. Since the majority of drugs are administered via the oral route, within this chapter, we mainly consider the options available in controlling drug release from oral solid dosage forms (SDFs), and only to a lesser extent from nonoral dosage forms, both in terms of rate and site of release. We consider how different release profiles can be achieved through the design of different release systems and use of appropriate excipients.
To understand the different types of drug release, in this chapter we categorize these into six basic groups: immediate release (IR), delayed release (DR), sustained release (SR), controlled release (CR), stimulus-sensitive release (SSR), and targeted release (TR). The pharmacopoeias, for example, the U.S. Pharmacopeia (USP), simply differentiate DDS into IR and modified-release (MR) systems; the latter can include DR, SR, and CR systems. Currently, SSR and TR systems are not defined in the pharmacopoeias, but certainly would also be categorized within the group of MR systems. We briefly describe the different types of drug release here:
• Immediate release (IR, also called “fast release”): In this type of drug release, the drug is released immediately after administration and is designed to give a fast onset of drug action (Figure 2.2a). This type of drug release is realized in a range of delivery systems including liquid and SDFs, and it is the most common type of drug release. Within the field of oral drug delivery, typical dosage forms are “normal” disintegrating tablets, as well as chewable, effervescent, sublingual, and buccal tablets.
• Modified release (MR): As stated earlier, this is a summarizing term, describing drug release that occurs sometime after administration (i.e., DR; Figure 2.2b), or for a sustained period (SR or CR; Figure 2.2c), or to a specific target in the body (TR). These systems tend to be used to improve therapeutic outcome of the drug intervention and/or to improve patient adherence. Modification of the time course and/or the site of drug release is more commonly achieved by SDFs, and oral SDFs can be designed to offer all MR formats.
• Delayed release (DR): In this type of drug release, the drug is not released immediately after administration but some time after administration. Within the field of oral drug delivery, typical DR dosage forms are enteric-coated tablets (Figure 2.2b), which delay drug release until the small intestine.
• Sustained release (SR, also called “prolonged release,” or “extended release”): In SR dosage forms, the drug is released over a prolonged period of time. The drug is thus released slower than in IR delivery systems, so that therapeutic plasma levels are maintained over a prolonged period of time (typically 8–12 hours) (Figure 2.2c). Sometimes, an initial fast release of a part of the dose gives rise to a fast onset of drug action, followed by a slower release of the drug over time, to maintain plasma levels. Such dosage forms would also be classified as SR systems. Within the field of oral drug delivery, typical dosage forms are polymer-coated pellets (reservoir systems) and matrix tablets.
• Controlled release (CR): In this type of drug release, the drug is released at a predetermined rate for a prolonged period of time, so that effective drug plasma levels are maintained and controlled over an extended period of time. In contrast to SR dosage forms, CR delivery systems aim to control both the drug release from the dosage form and also the subsequent plasma levels; therefore, in principle at least, plasma levels are solely determined by the drug release kinetics from the DDS. The plasma profile from a CR DDS would then be similar to that achieved via an intravenous infusion. Within the field of oral drug delivery, an example is the oral osmotic system (OROS), but CR delivery systems are also used for other routes of administration, such as transdermal patches, ocular inserts, and implant devices, as discussed further in the following text.
• Stimulus-sensitive release: Drug release is a function of a specific biological/pathological signal (e.g., glucose concentration, to trigger the release of insulin), or an externally applied stimulus (e.g., temperature, or pH). Such systems are still in the early stages of development and are described further in Chapter 14.
• Targeted release (TR): While all of the aforementioned types of drug release control the release of the drug from the dosage forms, or in the case of CR also the plasma levels, none of these dosage forms can influence the distribution of the drug in the body, for example, to specifically reach the site of drug action. However, with TR, it is attempted to deliver the drug to a specific target where the drug release is triggered, for example, through a time delay or as a result in a change in the environment surrounding the MR system (e.g., a change in pH). This can offer enhanced drug efficacy and reduced toxicity; however, TR of a drug does not ensure that the drug is retained at the site of action. Targeted drug delivery using a range of systems is the focus of Chapter 5 and will not be considered further here.
A word of caution: While we have just categorized and defined the different types of drug delivery, to highlight the different principles, it should be noted that for any given dosage form, a strict categorization may be far from simple. For example, in practice it is often difficult to clearly differentiate between SR and CR delivery systems. In fact, after a history of about 60 years of development in drug delivery, the terms CR and SR are often now used interchangeably. SR systems may also show initial IR properties. Furthermore, the release of DR systems, after the delay, may be of an IR or SR type. The development of any release system should not be guided by the desire to achieve a predetermined type of release, but by the principle to develop an optimal delivery system for the specific drug and treatment regime, to maximize patient adherence and therapeutic outcome.
2.1.2 MECHANISMS OF CONTROLLING DRUG RELEASE
Drug release can be controlled via a variety of mechanisms. Chemical approaches utilize chemical degradation, or enzymatic degradation, to effect CR of drug molecules from a drug–polymer conjugate. Physical approaches focus on four main mechanisms:
The drug is associated with a polymeric carrier, which slowly dissolves, thereby liberating the drug. The polymeric carrier can be as follows:
1. A reservoir system (encapsulated dissolution system), whereby a drug core is surrounded by a polymeric membrane. The rate of drug release is determined by the thickness and dissolution rate of the membrane.
2. A matrix system, whereby the drug is distributed through a polymeric matrix. Dissolution of the matrix facilitates drug release.
The drug must diffuse through a polymeric carrier. Again, two main types of design system are used:
1. A reservoir system, whereby the drug is surrounded by a polymeric membrane, known as a rate-controlling membrane (RCM). The rate of drug release is dependent on the rate of diffusion through the RCM.
2. A matrix system (also known as a monolithic device), whereby the drug diffuses through a polymeric matrix.
2.1.2.3 Osmotic Pressure Control
Osmotic pressure induces the diffusion of water across a semipermeable membrane, which then drives drug release through an orifice of the DDS.
Ion-exchange resins are water-insoluble polymeric materials that contain ionic groups. Charged drug molecules can associate with an ion-exchange resin via electrostatic interaction between oppositely charged groups. Drug release results from the exchange of bound drug ions with ions commonly available in body fluids (such as Na+, K+, or Cl–).
However, in many cases, a combination of factors is involved, and this can be exploited to give a range of release profiles. Mechanisms of drug release will be discussed further in the following sections.
2.2 IMMEDIATE RELEASE DELIVERY SYSTEMS
As mentioned earlier, the primary role of IR dosage forms is to give a rapid onset of drug action and to achieve a high plasma concentration quickly. Most drugs exert their action through interaction with receptors in the body. To enter into the body, for example, from the GI tract and to interact with biological receptors, the drug must be in solution, and hence liquid dosage forms can offer advantages in this respect. If a drug is delivered in a SDF, disintegration and dissolution are required so that the drug is released into solution. Therefore, both solubility, and dissolution rate, control drug release from the delivery system. However, drug absorption across the epithelial barriers is also required; therefore, drug membrane permeability must also be considered. It should be noted though, that influencing permeability by means of drug delivery is much more difficult than influencing disintegration, dissolution, or solubility. The most common type of any solid dosage form (SDF) is the IR tablet. These tablets are therefore often referred to as plain or conventional tablets. If a fast onset of drug action is required from SDFs, key factors that can be considered are (1) improving drug dissolution, (2) improving drug solubility, and (3) improving dosage form disintegration.
2.2.1 IMPROVING DRUG DISSOLUTION RATES AND SOLUBILITY
During dissolution of solid drug particles in the GI tract, the molecules in the surface layer dissolve, creating a saturated solution of the drug at the surface of the particle, which in turn, through diffusion, leads to the formation of a drug concentration gradient around the particles: from saturation concentration (Cs) at the surface, to the equilibrium bulk concentration of the dissolved drug (Ct; initially this will be zero). This diffusion layer has to be crossed by further dissolving drug molecules, which then diffuse into the GI tract and eventually reach the epithelial membrane for absorption. The Noyes–Whitney equation is often used to describe the dissolution of particles and can be applied here to understand the dissolution process. It states that the rate of mass transport of drug molecules through the aforementioned diffusion layer (dm/dt) is directly proportional to the available surface area (A) and the concentration difference across the diffusion layer (ΔC = Cs – Ct) and inversely proportional to the thickness of the diffusion layer (h). The term D is the diffusion coefficient of the dissolving molecules (in our case, the drug):
If the difference between the saturation concentration (Cs) and the concentration of drug dissolved (Ct) is large, i.e., if the solubility of the drug is high and the dose of the drug to be dissolved is low, Equation 2.1 may be simplified to
From the Noyes–Whitney equation, we can see that the dissolution rate can be increased if the surface area available for dissolution is increased (i.e., if the size of the particles from which dissolution occurs is decreased) and if the saturation concentration (i.e., the solubility) of the drug is high.
For IR DDS, high drug solubility and a fast dissolution rate are of the utmost importance to achieve the desired release and thus the desired plasma concentration profile. However, if the solubility and dissolution rate of a given drug are low, there is a range of options to improve dissolution rate and solubility, such that an IR dosage form can be formulated. Mechanisms to improve the water solubility of solid oral IR dosage forms are discussed in detail in Chapter 3. To summarize here, some of the options include using salt forms of drugs, rather than using the free acid or base form; using micronized or even nanonized drug particles to prepare the SDF in order to improve the dissolution rate; and using amorphous forms of the drug rather than a crystalline form. Of these methods, currently the formulation of drugs as salts is a commonly used approach to improve solubility and dissolution rate.
To increase the solubility of a drug through salt formulation, the pKa values of the drug and the counterion need to be considered; as a “rule of thumb,” for acidic drugs, the pKa of the counterions should be at least 2 pH values higher than that of the drug, and for basic drugs, the pKa of the counterion should be at least 2 pH units below that of the drug. In pharmaceutical development, a lot of effort is placed on finding the best salt form for a given drug, but it is fair to say that increasing dissolution rate and solubility are not the only concerns in this so-called salt screening process, but stability and processability also need to be considered. It should also be noted that not all drugs can be converted into a salt form, and even in cases where this is possible, it might not be the pursued option, due to stability or processing problems.
Another method to improve the solubility of a compound is to use a metastable polymorphic crystalline form or the amorphous form of the drug, instead of the stable crystalline polymorph. While using a metastable polymorph only in exceptional cases leads to a substantial increase in solubility, using the amorphous form instead of a crystalline form of the drug has gained considerable interest within the pharmaceutical industry in recent years as a suitable strategy to address solubility issues of poorly water-soluble compounds. The amorphous form does not have a crystal lattice structure, but the molecular arrangement is that of a “frozen liquid.” The absence of a crystal lattice means that the molecules in an amorphous form are in a higher energetic state compared to the crystalline form and thus show an often very significant increase in solubility. However, the use of amorphous forms has to be treated with great caution as the amorphous form is thermodynamically unstable, and crystallization of the drug may occur during manufacturing, storage, or even administration.
2.2.2 IMPROVING DISINTEGRATION: FAST-DISPERSING PRODUCTS
From the Noyes–Whitney equation, we have seen that increasing the surface area available for dissolution will increase the dissolution rate. Thus, improving the disintegration time of a formulation has become a relatively common strategy, leading to the development of orally disintegrating tablets (ODTs) (also known as “fast melts”) and orally dissolving/disintegrating films. Both systems offer the advantages of tablets (dose accuracy, ease of administration) combined with those of liquid dosage forms (easy to swallow and rapid drug absorption). In the case of ODTs, these are designed to disintegrate or even completely dissolve in a matter of seconds after contact with saliva, thereby removing the need to chew a tablet or swallow an intact tablet. FDA guidance on these formulations states that ODTs should have an in vitro disintegration time of approximately 30 seconds or less, determined using the USP disintegration test method, and that generally the ODT tablet weight should not exceed 500 mg. Current technologies used in commercially available ODTs can be divided into three main categories: (1) lyophilized tablets, (2) compressed tablets, and (3) others—including molded tablets, spray-dried powders, sugar floss, and films. Table 2.1 lists examples of various ODT technologies. In these systems, the ODT platforms offer bioequivalence to conventional oral SDFs.
TABLE 2.1
Examples of Orally Disintegrating Tablet Technology
Name | Proprietary Technology | Drug | Clinical Indication |
Lyophilized tablets | |||
Zofran Zydis | Zydis | Ondansetron | Chemotherapy-associated nausea |
Loperamide Lyoc | Lyoc | Loperamide | Diarrhea |
Propulsid Quicksolv Compressed tablets | Quicksolv | Cisapride | Oral prokinetic |
Lactimal ODT | Advatab | Lamotrigine | Epilepsy |
Remeron Soltab | Orasolv/Durasolv | Mirtazapine | Depression |
Prevacid Solutab | Flashtab | Lansoprazole | Heartburn, ulcers |
Other | |||
Ralivia | Flashdose (cotton candy) | Tramadol HCl | Opioid analgesic |
Benadryl FastMelt | Wow-Taba | Diphenhydramine | Allergy |
Zuplenz | PharmFilm (film) | Ondansetron | Chemotherapy-associated nausea |
a Wow-Tab, without water tablet. |
In the case of lyophilized systems, rapid disintegration can be promoted by forming the waferlike, highly porous structure obtained using the freeze-drying process. In the case of the Zydis® system, the drug is dispersed in a matrix consisting of a water-soluble polymer (e.g., gelatin) to give the tablet structure and a sugar or sugar alcohol (typically mannitol). This highly porous structure promotes rapid penetration of water, dissolution of the matrix, and rapid disintegration.
The basis of compressed tablet ODTs is the use of superdisintegrants or effervescent agents, which improve the dispersion of the dosage form in the fluids of the GI tract. Superdisintegrants are commonly starch, cellulose, or poly(vinylpyrrolidone) (PVP) derivatives and examples include sodium starch glycolate, croscarmellose, and cross-linked PVP. As these are all cross-linked and water insoluble, when in contact with water, the polymers rapidly swell, resulting in fast disintegration.
Alternative approaches to enhancing disintegration time include the production of fibers from molten saccharides (sucrose, dextrose, or lactose) or polysaccharides. Flashdose® uses this technology, and the high solubility of the sugar components, combined with the porosity of the system, promotes rapid disintegration. In the case of Wow-Tab®, tablets are molded using highly soluble sugars that dissolve rapidly.
In addition to tablet formulations, thin-film technologies are now being used to prepare oral fast-dispersing systems. These films are several micrometers (e.g., 50–200 μm) in thickness and consist of hydrophilic polymers. The drug concentration is controlled through the concentration in the film and the film thickness. The films are prepared by liquid casting and evaporation of the solvent within ovens. The dried film is then cut into single unit doses. These systems offer rapid disintegration, but are limited in drug loading and require specific packaging (protection from moisture). There is also a range of buccal, sublingual, and nasal IR DDS available, and the reader is referred to Chapters 8 and 10 respectively, for further details on these systems.
2.3 DELAYED RELEASE ORAL DRUG DELIVERY SYSTEMS
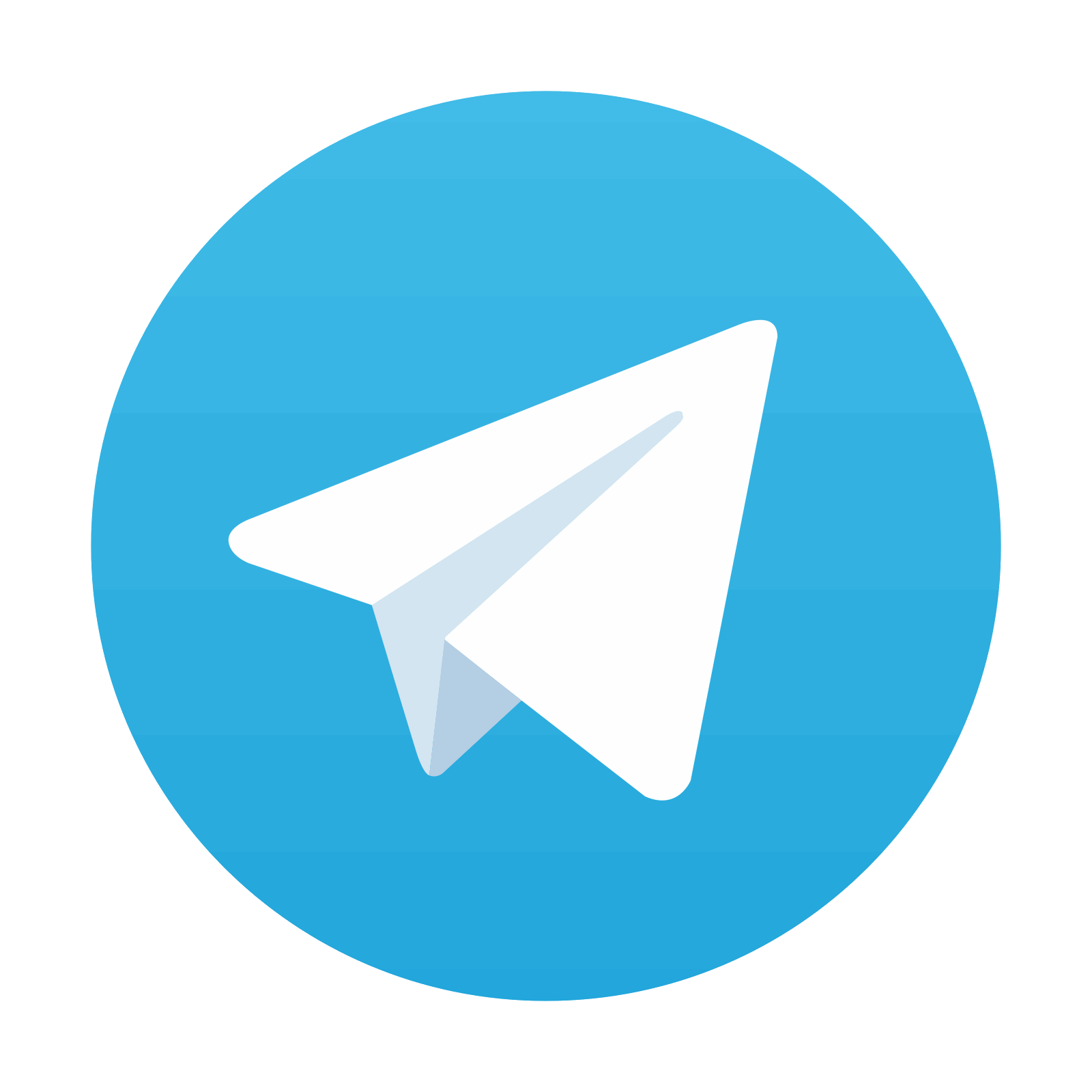
Stay updated, free articles. Join our Telegram channel

Full access? Get Clinical Tree
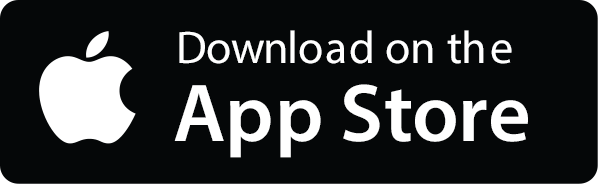
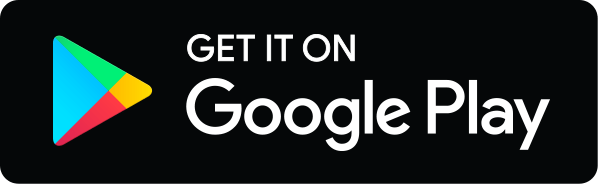