Polyphenols and Flavonoids1
Ronald L. Prior
1Abbreviations: C3G, cyanidin-3-glucoside; DP, degree of polymerization; MAPK, mitogen-activated protein kinase; PA, proanthocyanidin.
Flavonoids, a subclass of phytochemicals, constitute a large class of food constituents, many of which alter metabolic processes and have positive impacts on health. Two books have been published dealing specifically with this group of phytochemical compounds: The Flavonoids: Advances in Research Since 1980, which appeared in 1988 (1), and The Flavonoids: Advances in Research Since 1986, which appeared in 1994 (2). Subsequently, in 2000, a review was published detailing some of the advances in flavonoid research since 1992 (3). In addition, four widely respected books have been published based on proceedings of international phytochemical conferences: Phytochemicals: A New Paradigm (4), Phytochemicals as Bioactive Agents (5), Phytochemicals in Nutrition and Health (6), and Phytochemicals: Mechanisms of Action (7). Readers are also pointed to the classic reviews by the late Elliott Middleton Jr (8, 9, 10), which very eloquently deal with the impact of plant flavonoids on mammalian biology, with emphasis on immunity, inflammation, and cancer. More recently, specific reviews dealing with flavonoids have been published by Beecher (11), as part of an international symposium on tea and human health, and by Havsteen (12), Lambert and Yang (13), and Yang et al (14) on the medical significance of flavonoids and cancer chemopreventive properties of tea and other polyphenolics.
Early research in the field of flavonoids was stimulated by the discovery of the French Paradox, that is, the low cardiovascular mortality rate observed in Mediterranean populations in association with red wine consumption despite high saturated fat intake (15). Further impetus for flavonoid research resulted from epidemiologic studies relating dietary intake to protection against various cancers (16, 17) and cardiovascular disease (18, 19).
MAJOR CLASSES OF FLAVONOIDS FOUND IN FOODS
Flavonoids are perhaps the most important single group of phenolic compounds in plants. They can be divided by their basic structure into several different classes. More than 5000 such compounds have been described. Flavonoids are widely distributed in nature, albeit not uniformly. As a result, specific groups of foods are often rich sources of one or more subclasses of these polyphenols. Flavonoids are often used by botanists for taxonomic classification. These substances may regulate plant growth, inhibit or kill many bacterial strains, inhibit important viral enzymes, and destroy some pathogenic protozoans. However, their toxicity to animal cells is low (12). The content of flavonoids in foods is strongly influenced by variations in plant type, growing conditions, season, freshness, degree of ripeness, food preparation, and processing (20). Plant stressors can also have major effects on the levels of flavonoids in the plant.
Flavonoid structure is based on a 2-phenyl-benzo[α] pyrane or flavane nucleus (Fig. 37.1). This nucleus
is defined by having a system of two benzene rings (A and B), which are connected by an oxygen-containing pyrane ring (C). The flavonoids are further divided into subclasses (flavones, flavonols, flavanones, flavanols, proanthocyanidins [PAs], anthocyanins, and isoflavones) based on the connection of the aromatic B ring to the heterocyclic ring as well as the oxidation state and functional groups of the heterocyclic ring. Within each subclass, individual compounds are characterized by specific hydroxylation and conjugation patterns. Because of limitations in space, the isoflavonoids are not considered in this chapter, but readers are referred to the previous edition of this book (21).
is defined by having a system of two benzene rings (A and B), which are connected by an oxygen-containing pyrane ring (C). The flavonoids are further divided into subclasses (flavones, flavonols, flavanones, flavanols, proanthocyanidins [PAs], anthocyanins, and isoflavones) based on the connection of the aromatic B ring to the heterocyclic ring as well as the oxidation state and functional groups of the heterocyclic ring. Within each subclass, individual compounds are characterized by specific hydroxylation and conjugation patterns. Because of limitations in space, the isoflavonoids are not considered in this chapter, but readers are referred to the previous edition of this book (21).
FLAVONES
The basic flavane nucleus is the structural feature of the flavone (see Fig. 37.1). Among the flavones (˜300 are known), apigenin and luteolin are present chiefly in grains, leafy vegetables, and herbs. Flavonols, which are discussed later, are 3-hydroxyflavones. Many fewer research data are available on the flavones compared with the flavonols.
Apigenin has been shown to have strong cytostatic and antiangiogenic effects in vitro (22). 2′,3′-Dihydroxyflavone, fisetin, apigenin, and luteolin inhibit the proliferation of normal and tumor cells, as well as in vitro angiogenesis, at half-maximal concentrations in the lower micromolar range (23). Although flavonoids may show great potential as cancer chemopreventive agents in cell culture systems, this often does not translate well into in vivo activity (24). When polyphenols are administered orally, only small quantities of these compounds appear in systemic circulation because of very high levels of uridine diphospho (UDP)-glucuronosyltransferases and sulfotransferases in the small intestine and liver, thus resulting in very low oral bioavailability (24). The case for superior anticancer activity for methoxylated flavones has been reviewed by Walle (24).
FLAVONOLS
Chemistry
The flavonols (3-hydroxyflavones) are a subclass of flavonoids, which are diverse in their chemical structure and characteristics (see Fig. 37.1). Quercetin, kaempferol, and myricetin are the most common flavonols. Quercetin (3,3′,4′,5,7-pentahydroxyflavone) is one of the
most abundant and widely studied flavonoids. The availability of the pure compound made much of this research possible. The antioxidant effect of quercetin is thought to be helpful for human health. Fruits, vegetables, and beverages such as tea and red wine are major sources of flavonols in the human diet. Dietary quercetin is primarily present in plants in its glycoside form.
most abundant and widely studied flavonoids. The availability of the pure compound made much of this research possible. The antioxidant effect of quercetin is thought to be helpful for human health. Fruits, vegetables, and beverages such as tea and red wine are major sources of flavonols in the human diet. Dietary quercetin is primarily present in plants in its glycoside form.
Sites of Absorption, Blood Transport, and Intracellular Forms
Quercetin was originally assumed to be absorbed from the small intestine following cleavage of the β-glucoside linkage by colonic microflora (25). Hollman et al (26) found that humans absorb quercetin as the aglycone but concluded that absorption was enhanced by conjugation with glucose. Although quercetin glycosides are subject to deglycosidation by enterobacteria for absorption in the large intestine, the small intestine can act as an effective absorption site for glucose-bound quercetin glucosides. Data suggest that β-glucosidases and also lactase-phlorizin hydrolase in the small intestine may be capable of hydrolyzing quercetin glucosides, and that these compounds are thus taken up in the aglycone form and not as intact glycosides (27).
Despite some discrepancies in the literature, it seems that the absorption of quercetin glycosides from the diet depends largely on the type of sugar groups attached to their phenolic group. Glucose-bound glycosides are likely to be much more absorbable than others that are sugarbound (28). Hydrolysis to its aglycone form by enterocytes or enterobacteria seems to be important for the effective absorption of quercetin glycosides in the intestinal tract. Using a cell culture model for intestinal absorption, hydrolysis of quercetin glucosides accelerated quercetin absorption (28). Crespy et al (29) found that quercetin, but not its glycosides, were absorbed from the rat stomach.
Based on in vitro studies of Caco-2 cells, Walgren et al demonstrated a complete lack of absorption of the glucosides of quercetin, mainly as a result of effective efflux by the multidrug resistance protein 2 transporter (MRP2) (30, 31), but quercetin was readily absorbed. In subsequent studies in human subjects, Walle et al (32) found that quercetin glucosides were hydrolyzed in the small intestine by bacterial enzymes.
The small intestine is also recognized as the site for metabolic conversion of quercetin and other flavonoids because it possesses enzymatic activity for glucuronidation and sulfation. The major circulating metabolites are glucuronide-sulfate conjugates of isorhamnetin (3′-O-methyl quercetin) and of quercetin. Glucuronides, sulfates, and their O-methylated derivatives accumulate as quercetin metabolites in the circulation after intake of a quercetin-rich diet (28, 33). Modulation of the intestinal absorption and metabolism may be beneficial for regulating the biologic effects of dietary quercetin (28).
Hollman et al (34) found that absorption of quercetin in individuals with an ileostomy was 52 ± 5% for quercetin glucosides from onions, 17 ± 15% for quercetin rutinoside, and 24 ± 9% for quercetin aglycone. Excretion of quercetin or its conjugates in urine in four separate studies ranged from 0.07% to 17.4% of intake (35). Fecal microflora can rapidly deconjugate the flavonols rutin (quercetin-3-rutinoside), isoquercitrin, and a mixture of quercetin glucuronides. The main metabolite, 3,4-dihydroxyphenylacetic acid, appeared rapidly (within 2 hours) and was dehydroxylated to 3-hydroxyphenylacetic acid within 8 hours. Hydroxyphenylacetic acids were not methylated by colon flora in vitro (36).
Biologic Effects
Biologic effects of flavonols vary depending on the flavonol metabolites produced. Following intragastric administration of quercetin (10 or 50 mg/kg), quercetin glucuronide or sulfate conjugates, or both, were present in the plasma, and the plasma was more resistant to copper sulfateinduced lipid peroxidation than the control plasma on the basis of the accumulation of cholesterol ester hydroperoxides and the consumption of α-tocopherol (37). These results suggest that some conjugated metabolites of quercetin can act as effective antioxidants. Antioxidant activity of conjugated metabolites has also been observed in vitro (33, 38).
Numerous other in vitro effects have been reported (8, 21, 39, 40, 41, 42). However, one study suggested that quercetin supplementation in humans in doses of 500 to 1000 mg/day for 12 weeks increased plasma quercetin concentration but did not alter several measures of oxidative stress or antioxidant capacity (43). Studies have indicated that dietary quercetin may inhibit bone loss in ovariectomized mice (44), and it may increase mitochondrial biogenesis with an associated increase in maximal endurance capacity and voluntary wheel running activity in mice (45). However, more clinical trials in humans are necessary to confirm whether quercetin may have effects on exercise outcomes (46, 47, 48).
FLAVANONES
Chemistry
Flavanones constitute most of all the flavonoids in citrus fruits, including oranges, tangerines, mandarins, grapefruit, lemons, and limes (see Fig. 37.1). Overall, citrus flavonoids encompass a diverse set of structures, including numerous flavanone and flavone O– and C-glycosides and methoxylated flavones. Primary flavanones in citrus include hesperidin, naringin, narirutin, eriocitrin, neohesperidin, didymin, neoeriocitrin, and poncirin. Liquid chromatography-mass spectometry analyses of 12 dietary flavonoids in human urine showed that no flavonoid glycosides were excreted, and the citrus flavanones were excreted in higher amounts than the flavonols (49).
Biologic Effects
Citrus flavonoids exhibit several in vitro and in vivo antiinflammatory and anticancer actions (50). These biologic properties are consistent with their effects on the microvascular endothelial tissue. Evidence suggests that the biologic actions of the citrus flavonoids are possibly linked to their interactions with key regulatory enzymes involved in cell activation and receptor binding. The citrus flavonoids show little effect on normal, healthy cells and thus typically exhibit remarkably low toxicity in animals. The citrus flavonoids extend their influence in vivo through their induction of hepatic phase I and II enzymes and through the biologic actions of their metabolites (27, 50).
Phytochemicals in grapefruit juice have some unique properties that can change the bioavailability of certain drugs and alter drug action. Naringin is likely one of the grapefruit components influencing drug metabolism. Grapefruit juice acts by inhibiting presystemic drug metabolism mediated by the cytochrome P-450 isoenzyme CYP3A4 in the small intestine. This interaction appears to be more relevant if the CYP3A4 content is high and the drug has a high degradation rate on first pass through the liver (51, 52).
FLAVAN-3-OLS (CATECHINS)
Chemistry
Monomeric flavanols or catechins, which are precursors of PAs, are characterized by having a C6-C3-C6 skeleton with a hydroxyl group in the 3-postion of the C-ring (see Fig. 37.1). Catechin and epicatechin are the most common of the flavan-3-ols. Their galloyl-substituted derivative gallocatechin (GC), epigallocatechin (EGC), epicatechin gallate (ECG), and epigallocatechingallate (ECGC) are the forms usually found in foods, particularly in teas. They are not usually found in their glycosylated from, unlike some other flavonoids such as anthocyanins.
Sites of Absorption, Blood Transport, and Intracellular Forms
Catechins are bioavailable. Human intervention studies with green and black tea demonstrate a significant increase in plasma antioxidant capacity approximately 1 hour after consumption of moderate amounts of tea (1 to 6 cups/day). The initial indications are that the enhanced blood antioxidant potential leads to reduced oxidative damage to macromolecules such as DNA and lipids. However, the measurement of oxidative damage through biomarkers needs to be further established (53). The apparent bioavailability of the gallated catechins is lower than the nongallated forms (54). However, absorbed gallated catechins are rapidly eliminated by excretion in the bile.
Catechins are excreted as free and conjugated in both intact and methylated forms. In animal studies, excretion of conjugated (epi) catechins was as high as 60% of the total consumed in some cases (55). Most of both catechins and epicatechins excreted in the urine were in a methylated form at the 3′ or 4′ positions (55, 56, 57). Excretion of epicatechins, including their methylated forms, ranged from 30% to 47% of the ingested amount, whereas that of catechins, including their methylated forms, ranged from 9% to 31% (55). Urinary excretion of (epi) catechins was dose dependent and increased with the amount of (epi) catechins present in the diet. Based on excretory pattern of (epi) catechins in the urine, the bioavailability of epicatechins may be higher than catechins in rats (55).
Plasma from humans was shown to contain up to 18 metabolites of (epi) catechin and (epi) gallocatechin, principally methylated, sulfated, and glucuronidated conjugates with peak plasma concentrations of 100 to 400 nM, which occurred within 0.8 to 2.3 hours of consumption, depending on the source and dose (56, 58, 59). (epi) Catechins are highly bioavailable, being absorbed and excreted to a much greater extent than most other flavonoids. Moreover, flavan-3-ol metabolites are rapidly turned over in the circulatory system, and, as a consequence, maximal concentration values are not an accurate quantitative indicator of the extent to which absorption occurs (58).
Biologic Effects
Tea remains the most widely consumed drink in the world after water. An accumulated number of population studies suggested that consumption of green and black tea beverages may bring positive health effects. One hypothesis explaining such effects is that the high levels of flavonoids in tea can protect cells and tissues from oxidative damage by scavenging oxygen free radicals. The effects of tea and green tea catechins on biomarkers of oxidative stress, especially oxidative DNA damage, appear very promising in animal models, but data on biomarkers of in vivo oxidative stress in humans are limited and insufficient to draw firm conclusions (53, 60, 61).
Catechins may also function indirectly as antioxidants through the following: (a) inhibition of the redox-sensitive transcription factors, nuclear factor-κB and activator protein-1; (b) inhibition of “pro-oxidant” enzymes, such as inducible nitric oxide synthase, lipoxygenases, cyclooxygenases, and xanthine oxidase; and (c) induction of phase II and antioxidant enzymes, such as glutathione S-transferases and superoxide dismutases. Chemically, the flavonoids found in green and black tea are very effective radical scavengers. The tea flavonoids may therefore be active as antioxidants in the digestive tract or in other tissues after uptake.
Population studies of the association of high intakes of catechins with cancer incidence indicated that catechins derived primarily from fruits, (+)-catechin and (−)-epicatechin, tended to be inversely associated with upper digestive tract cancer, whereas catechins derived
from tea were inversely associated with rectal cancer in postmenopausal women (62). Many epidemiologic, casecontrol, and cohort studies have investigated the effects of tea consumption on human cancer incidence (63, 64, 65, 66, 67, 68, 69). A brief synopsis (70) of 30 studies aimed at examining tea consumption as a factor in the incidence of colon and rectal cancers from 12 countries, and with data on consumption of both black and green tea, did not provide consistent evidence to support the theory from animal studies and basic research that tea is a potent chemopreventive agent. The assessment of tea consumption in most of these studies was based on a single question and therefore may be subject to significant measurement error compared with more recent studies specifically aimed at assessing tea consumption (70).
from tea were inversely associated with rectal cancer in postmenopausal women (62). Many epidemiologic, casecontrol, and cohort studies have investigated the effects of tea consumption on human cancer incidence (63, 64, 65, 66, 67, 68, 69). A brief synopsis (70) of 30 studies aimed at examining tea consumption as a factor in the incidence of colon and rectal cancers from 12 countries, and with data on consumption of both black and green tea, did not provide consistent evidence to support the theory from animal studies and basic research that tea is a potent chemopreventive agent. The assessment of tea consumption in most of these studies was based on a single question and therefore may be subject to significant measurement error compared with more recent studies specifically aimed at assessing tea consumption (70).
In animal models of atherosclerosis, green and black tea administration resulted in modest improvements in the resistance of lipoproteins to ex vivo oxidation, although limited data suggest that green tea or green tea catechins inhibit atherogenesis (61). One epidemiologic study indicated that high intake of tea and flavonoids may contribute to the primary prevention of ischemic heart disease (61). Cardiovascular effects of monomeric catechins have been reviewed (56).
PROANTHOCYANIDINS
Chemistry
PAs, better known as condensed tannins, are oligomeric and polymeric flavan-3-ols (see Fig. 37.1). They are ubiquitous and present as the second most abundant natural phenolic after lignin in plants. The flavan-3-ol units are linked mainly through the C4→C8 bond, but the C4→C6 bond also exists (both called B-type) (see Fig. 37.1). The flavan-3-ol units can also be doubly linked by an additional ether bond between C2→O7 (A-type). The size of the PA molecules can be described by the degree of polymerization (DP) (71).
Sites of Absorption, Blood Transport, and Intracellular Forms
Unlike the lower oligomers, or monomeric catechins, PAs with a DP greater than 3 appear not to be absorbed directly in the gastrointestinal lumen (72, 73). They have been suggested to depolymerize into mixtures of epicatechin monomers and dimers in the acidic environment of the stomach. The resultant monomers and dimers are absorbed in the small intestine (74). An observation in weaning pigs indicated that 8% to 15% of ingested polymers (DP > 10) were depolymerized after 4 hours in the stomach, with a concurrent increase of monomers, dimers, and trimers. It has been suggested that depolymerization is a slow process and that most PAs may transit into the small intestine intact (75). Approximately 65% of the ingested PAs were degraded in the entire gastrointestinal lumen at 4 hours after eating.
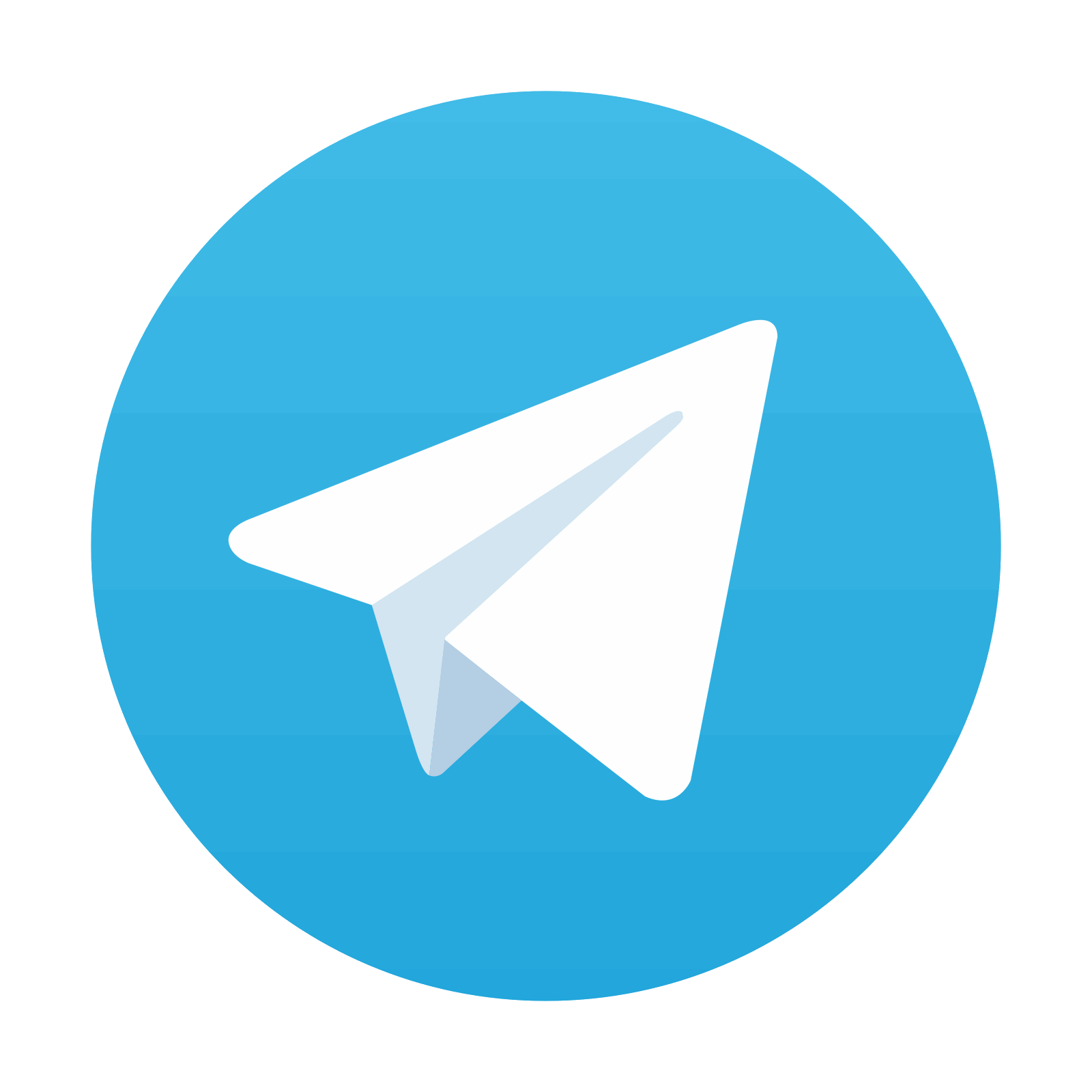
Stay updated, free articles. Join our Telegram channel

Full access? Get Clinical Tree
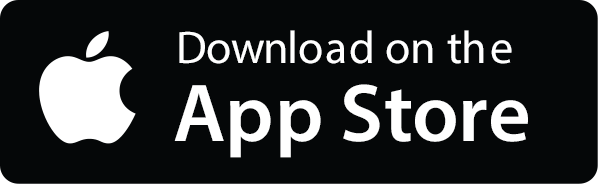
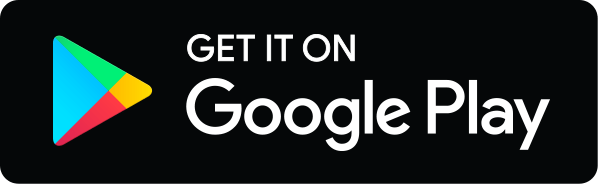