13 Pigments and minerals
Introduction
Endogenous pigments
Hematogenous
This group contains the following blood-derived pigments:
Demonstration of hemosiderin and iron
In unfixed tissue, hemosiderin is insoluble in alkalis but freely soluble in strong acid solutions; after fixation in formalin, it is slowly soluble in dilute acids, especially oxalic acid. Fixatives that contain acids but no formalin can remove hemosiderin or alter it in such a way that reactions for iron are negative. Certain types of iron found in tissues are not demonstrable using traditional techniques. This is because the iron is tightly bound within a protein complex. Both hemoglobin and myoglobin are examples of such protein complexes and, if treated with hydrogen peroxide (100 vol), the iron is released and can then be demonstrated using Perls’ Prussian blue reaction (Fig. 13.1). A similar result is obtained if the acid ferrocyanide solution is heated to 60°C in a water bath, oven, or microwave oven. However, the use of heat will sometimes cause a fine, diffuse, blue precipitate to form on both the tissue section and slide. This precipitate will not occur when the slides are stained at room temperature. Metallic iron deposits, or inert iron oxide seen in tissues because of industrial exposure, are not positive when treated with acid ferrocyanide solutions. As a consequence of the tissue response, various mechanisms release some of the iron in a demonstrable form, and such deposits are almost invariably surrounded by hemosiderin. In almost all the instances where demonstrable iron appears in tissues, it does so in the form of a ferric salt. On those rare occasions that iron appears in its reduced state as the ferrous salt, then Lillie’s (1965) method may be used to achieve the Turnbull’s blue reaction to visualize its presence in tissue sections (Fig. 13.2).

Figure 13.1 A section of liver from a patient with hemochromatosis stained for ferric iron with Perls’ method.

Figure 13.2 A section of placenta treated with ferrous sulfate and stained with Lillie’s method for ferrous iron.
An interesting and sometimes useful modification of a serum iron technique was introduced by Hukill and Putt (1962) to demonstrate both ferrous and ferric iron in tissue sections. This method was claimed to be a more sensitive demonstration for the detection of both ferric and ferrous salts, but has not succeeded in replacing the more traditional method for the demonstration of iron. The method uses bathophenanthroline and the resultant color of any iron present in tissues is bright red.
Perls’ Prussian blue reaction for ferric iron (Perls 1867)
Fixation
Avoid the use of acid fixatives. Chromates will also interfere with the preservation of iron.
Method
1. Take a test and control section to water.
2. Treat sections with the freshly prepared acid ferrocyanide solution for 10–30 minutes (see Note a below).
3. Wash well in distilled water.
4. Lightly stain the nuclei with 0.5% aqueous neutral red or 0.1% nuclear fast red.
5. Wash rapidly in distilled water.
Notes
a. Depending on the amount of ferric iron present, it may be necessary to vary the staining times.
b. Some laboratories keep the two stock solutions made up separately and stored in the refrigerator. The two solutions must not be stored for prolonged periods; this precaution will ensure that the solutions retain their viability.
c. It is essential that a positive control is used with all test sections. The choice of material that is most suitable as a control specimen is important. A useful control would be postmortem lung tissue that contains a reasonable number of iron-positive macrophages (heart failure cells). Freshly formed deposits of iron may be dissolved in the hydrochloric acid.
Lillie’s method for ferric and ferrous iron (Lillie & Geer 1965)
Fixation
Avoid the use of acid fixatives. Chromates will also interfere with the preservation of iron.
Method
1. Take test and control sections to distilled water.
2. Dissolve 400 mg of potassium ferrocyanide in 40 ml of 0.5% hydrochloric acid when testing for ferric iron. For testing ferrous iron substitute, 400 mg of potassium ferricyanide. Prepare just before use. Expose sections for 30 minutes.
3. Wash well in distilled water.
4. Stain nuclei with 0.1% aqueous nuclear fast red for 5 minutes.
Hukill and Putt’s method for ferrous and ferric iron (Hukill & Putt 1962)
Solution
Bathophenanthroline (4, 7-diphenyl-1, 10-phenanthroline) | 100 mg |
3% aqueous acetic acid | 100 ml |
Method
1. Take test and control sections to distilled water.
2. Stain sections in bathophenanthroline solution for 2 hours at room temperature.
3. Rinse well in distilled water.
4. Counterstain in 0.5% aqueous methylene blue for 2 minutes.
5. Rinse well in distilled water.
6. Stand slides on end until completely dry.
Hemoglobin
Demonstration of hemoglobin
Two types of demonstration method can be used to stain hemoglobin in tissue sections. The first demonstrates the enzyme, hemoglobin peroxidase, which is reasonably stable and withstands short fixation and paraffin processing. This peroxidase activity was originally demonstrated by the benzidine-nitroprusside methods, but because of the carcinogenicity of benzidine these methods are not recommended and are no longer used. Lison (1938) introduced the patent blue method that was later modified by Dunn and Thompson (1946) (Fig. 13.3). Tinctorial methods have also been used for the demonstration of hemoglobin; the amido black technique (Puchtler & Sweat 1962) and the kiton red-almond green technique (Lendrum 1949) are worth noting.

Figure 13.3 A section of kidney from a patient with hemoglobinuria stained for hemoglobin with leuco patent blue V.
Leuco patent blue V method for hemoglobin (Dunn & Thompson 1946)
Fixation
Formalin (removed formal mercury due to toxicity?) Poor preservation with Heidenhain’s Susa has been noted by Drury and Wallington (1980).
Solutions
Stock solution
1% aqueous patent blue V (CI 42045) | 25 ml |
Powdered zinc | 2.5 g |
Glacial acetic acid | 0.5 ml |
Method
Notes
a. Experience has shown that the hemoglobin demonstrated by this method tends to be more of a green-blue color.
b. In some instances, if the staining solution is left on the section for too long, it may start to decolor a positive reaction.
c. Fixation in excess of 36 hours may give rise to unreliable results.
d. This method demonstrates peroxidase activity, including the peroxidases in other blood cells, particularly in the lysosomes of polymorphonuclear leukocytes and their precursors. Tissue peroxidases are also demonstrated.
Bile pigments
In a hematoxylin and eosin (H&E) stained section of liver, bile, if present, is most commonly seen in the hepatocytes in the early stages as small yellow-brown globules and then subsequently within the bile canaliculi as larger, smooth, round-ended rods or globules commonly referred to as bile thrombi. The latter, if present, in liver sections is a histopathological indication that the patient has obstructive jaundice due to a blockage in the normal flow of bile from the liver into the gallbladder and subsequently into the bowel, probably because of gallstones or a carcinoma of the head of pancreas. Masses of bile in the canaliculi of liver sections are easily distinguished microscopically because of their characteristic morphology and their situation. Bile in hepatocytes must be distinguished from the lipofuscins that are also commonly seen within these cells and can appear as small yellow-brown globules. The need to distinguish between bile and lipofuscin in hepatocytes is particularly important in liver biopsies taken from liver transplant patients where sepsis is suspected. Bile is difficult to identify in the sections of normal liver. It is important to note that both bile and lipofuscin can be positive with Schmorl’s ferric ferricyanide reduction test (Golodetz & Unna 1909). Bile is also seen in H&E-stained sections in the gallbladder where it can appear as amorphous, yellow-brown masses adherent to the mucosa or included as yellow-brown globules within the epithelial-lined Aschoff-Rokitansky sinuses in the gallbladder. Bile is also present, together with cholesterol, in gallstones.
Virchow (1847) first described extracellular yellow-brown crystals and amorphous masses within old hemorrhagic areas, which he called hematoidin. Pearse (1985) reviewed the histochemistry of bile pigments. Microscopically, hematoidin frequently appears as a bright yellow pigment in old splenic infarcts, where it contrasts well against the pale gray of the infarcted tissue. Hematoidin can also be found in old hemorrhagic areas in the brain. Bearing in mind the differences discussed above, it is almost certain that hematoidin is related to both bilirubin and biliverdin, even though it differs from them both morphologically and chemically. It is thought that heme has undergone a chemical change within these areas which has led to it being trapped, thus preventing it from being transported to the liver to be processed into bilirubin.
Demonstration of bile pigments and hematoidin
The need to identify bile pigments arises mainly in the histological examination of the liver, where distinguishing bile pigment from lipofuscin may be of significant importance. Both appear yellow-brown in H&E-stained paraffin sections, and it is worth remembering that the green color of biliverdin is often masked by eosin. In such cases, unstained paraffin or frozen sections, lightly counterstained with a suitable hematoxylin (e.g. Mayer), will prove of value. Bile pigments are not autofluorescent and fail to rotate the plane of polarized light (monorefringent), whereas lipofuscin is autofluorescent. The most commonly used routine method for the demonstration of bile pigments is the modified Fouchet technique (Hall 1960), in which the pigment is converted to the green color of biliverdin and blue cholecyanin by the oxidative action of the ferric chloride in the presence of trichloroacetic acid (Fig. 13.4). The Fouchet technique is quick and simple to carry out, and when counterstained with van Gieson’s solution the green color is accentuated.
Modified Fouchet’s technique for liver bile pigments (Hall 1960)
Method
Notes
a. Two control sections are stained with the test section, one stained with Fouchet’s reagent and van Gieson, and the other with Fouchet’s reagent alone.
b. Although the solutions used in Fouchet’s reagent have a reasonable shelf life, experience has shown that a freshly prepared solution gives a more reliable result.
c. Bile that may be present in situations outside the liver such as that seen in the Aschoff-Rokitansky sinuses or in hemorrhagic and infarcted areas is likely to show no color change with this method. This type of pigment can be shown using the Gmelin (see below) or the Stein technique. Sirus Red F3B (CI 35780) may be substituted for acid fuchsin. Bile is a reducing substance and therefore will stain with the Masson-Fontana and Schmorl techniques.
Gmelin technique (Tiedermann & Gmelin 1826)
This technique is the only method that shows an identical result with liver bile, gallbladder bile, and hematoidin. The method tends to be messy, capricious, and gives impermanent results. Deparaffinized sections of tissue containing bile pigments are treated with nitric acid, and a changing color spectrum is produced. Because it can be unreliable, it is advisable to repeat the test at least three times before a negative result is acceptable. A popular modification of this technique is that of Lillie and Pizzolato (1967), in which bromine in carbon tetrachloride is used as an oxidant.
Method
1. Sections to distilled water and mount in distilled water.
2. Place mounted section under the microscope using an objective with reasonable working distance.
3. Place 2–3 drops of concentrated nitric acid to one side of the coverglass and draw under the coverglass by means of a piece of blotting paper on the opposite side.
4. Remove excess solution and observe pigment for color changes.
Oxidation methods aim to demonstrate bilirubin by converting it to green biliverdin. In practice they fail to produce the bright blue-green color seen in the more popular Fouchet technique and tend to be a dull olive green color. These oxidation methods are of little value in routine surgical pathology and are rarely used. Another group of methods that has been used to demonstrate bile pigments is the diazo methods that are based on a well-known technique previously used in chemical pathology, namely the van den Burgh test for bilirubin in blood. The method is based on the reaction between bilirubin and diazotized sulfanilic acid. Raia 1965, 1967) modified the method for use on cryostat sections but the reagents are complex to make up and section loss may be high; therefore its use is limited.
Non-hematogenous endogenous pigments
This group contains the following:
Melanins
Melanins are a group of pigments whose color varies from light brown to black. The pigment is normally found in the skin, eye, substantia nigra of the brain, and hair follicles (a fuller account of these sites is given later.) Under pathological conditions, it is found in benign nevus cell tumors and malignant melanomas. The chemical structure of the melanins is complex and varies from one type to another. Melanin production is not fully understood but the generally accepted view is that melanins are produced from tyrosine by the action of an enzyme tyrosinase (syn. DOPA oxidase). This enzyme acts on the tyrosine slowly to produce the substance known as DOPA (dihydroxyphenylalanine) which is subsequently rapidly acted upon by the same enzyme to produce an intermediate pigment which then polymerizes to produce melanin. The later stages of melanogenesis remain largely speculative, and it is beyond the scope of this chapter to evaluate the many studies relating to melanin biosynthesis that have been carried out recently. Pearse (1985) gives a more detailed account of melanin production.
The melanins are bound to proteins, and these complexes are localized in the cytoplasm of cells within so-called ‘melanin granules’. Ghadially (1982) described these granules as the end stage of the development of the melanosome as seen at ultrastructural level.
There are four recognized stages of melanosome maturation:
1. Tyrosine is synthesized in the Golgi lamellae and pinched off into vesicles with no melanin present.
2. The characteristic lattice-like appearance becomes evident at this stage.
3. Melanin deposition is first observed.
4. The fully mature granule has its structure obscured by melanin pigment.
Ultrastructurally the lamellar structures become increasingly difficult to see following melanin deposition. By the time the melanosome reaches stage 4 the lamellae structures are completely obscured (Fig. 13.5).
The enzyme tyrosinase cannot be demonstrated in the mature granule.
The most common sites where melanin can be found are
1. Skin, where it is produced by cells called melanocytes that are usually scattered within the basal layer of the epidermis. In certain inflammatory skin diseases melanin may also be found in phagocytic cells (‘melanophages’) in the upper dermis. The melanophages may also phagocytize other material such as lipofuscins and lipoproteins, thus producing a mixture that after denaturation may give unexpected staining results. Pathological deposition of melanin occurs in a benign lesion called a nevus or ‘mole’. The malignant counterpart to the nevus is the malignant melanoma; it is in the diagnosis of this important tumor, and its metastases, that histological demonstration of melanin finds its most important practical application (Fig. 13.5). Melanin is also found in the hair follicles of dark-haired people.
2. Eye, where it is found normally in the choroid, ciliary body, and iris. Similar brown-black pigment is found in the retinal epithelium but its identity with melanin is uncertain. Melanomas can occur in the eye but these tumors are rare.
3. Brain, where it is found particularly in the substantia nigra, in such quantities that this structure is macroscopically visible as a black streak on both sides of the mesencephalon. In patients with long-standing Parkinson’s disease this area is markedly reduced. Black melanin is also found in patches in the arachnoid covering some human brains, and has been described as having a ‘sooty’ appearance.
1. Reducing methods such as the Masson-Fontana silver technique and Schmorl’s ferric-ferricyanide reduction test.
2. Enzyme methods (e.g. DOPA reaction).
3. Solubility and bleaching characteristics.
Reducing methods for melanin
Melanin is a powerful reducing agent and this property is used to demonstrate melanin in two ways:
1. The reduction of ammoniacal silver solutions to form metallic silver without the use of an extraneous reducer is known as the argentaffin reaction. Masson’s (1914) method (using Fontana’s silver solution) and its various modifications, which also rely on melanin’s argentaffin properties, are now widely used for routine purposes. Melanins are blackened by acid silver nitrate solutions. Melanin is also argyrophilic, meaning that melanin is colored black by silver impregnation methods that use an extraneous reducer (Fig. 13.6). This is not a property considered to be of diagnostic value.
2. Melanin will reduce ferricyanide to ferrocyanide with the production of Prussian blue in the presence of ferric salts (the Schmorl reaction). This type of reaction (Fig. 13.7) is also seen with certain other pigments (e.g. some lipofuscins, bile, and neuroendocrine cell granules).
3. Other methods for demonstrating melanin are Lillie’s ferrous ion uptake (described in Lillie & Fullmer 1976) and Lillie’s Nile blue A (1956).
Masson-Fontana method for melanin (Fontana 1912; Masson 1914)
Sections
Works on all types of section, although some adjustment may be necessary for resin sections.
Preparation of silver solution (after Fontana)
Note: Ammoniacal silver solutions are potentially explosive if stored incorrectly.
Method
1. Take test and control sections to distilled water.
2. Treat with the ammoniacal silver solution in a Coplin jar that has been covered with aluminum foil, for 30–40 minutes at 56°C or overnight at room temperature.
3. Wash well in several changes of distilled water.
4. Treat sections with 5% aqueous sodium thiosulfate (also known as hypo) for 1 minute.
5. Wash well in running tap water for 2–3 minutes.
6. Lightly counterstain in 0.5% aqueous neutral red or 0.1% aqueous nuclear fast red for 5 minutes.
Notes
a. Only use thoroughly clean glassware, as the silver solution will react with any residual contaminant left on glassware.
b. Prolonged exposure to 56°C may give rise to a fine deposit over the section.
c. Friable material may need to be coated with celloidin as the ammonia in the silver solution could lead to sections lifting off the slide.
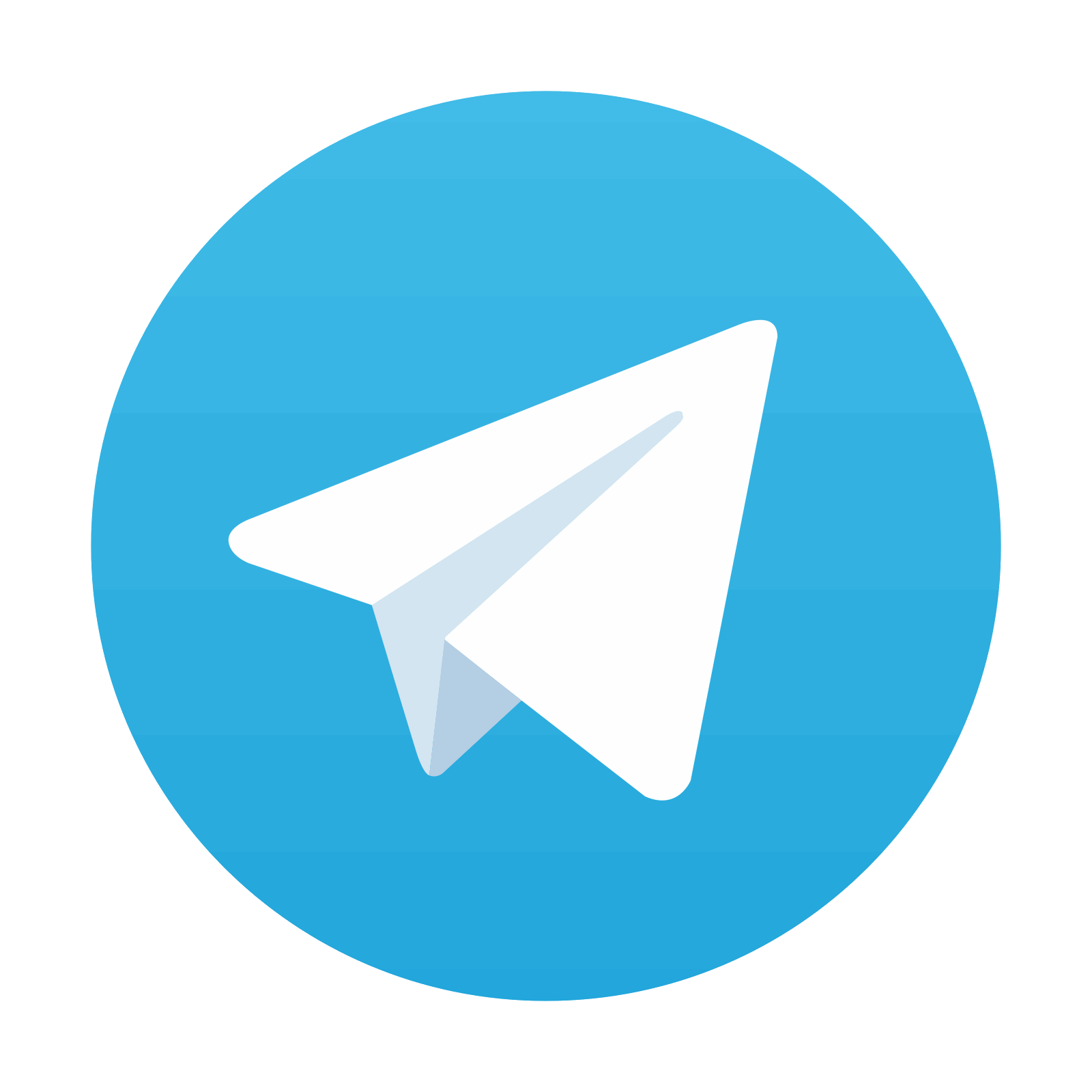
Stay updated, free articles. Join our Telegram channel

Full access? Get Clinical Tree
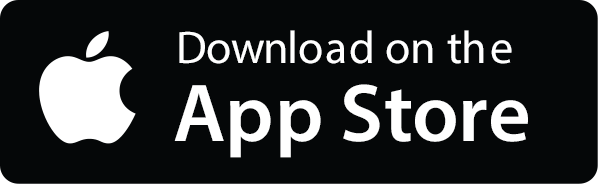
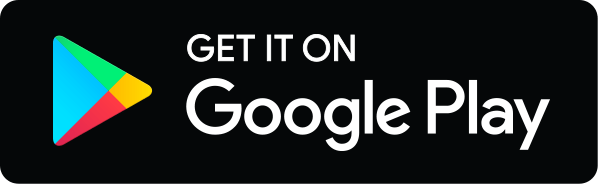
