21.1 INTRODUCTION TO ORIGINS AND EVOLUTION OF VIRUSES
In this chapter we shall speculate on how viruses may have originated and consider the mechanisms by which viruses evolved in the past and continue to evolve today. The origins and evolution of many cellular organisms can be inferred from fossils, but the fossil record of viruses is limited. The discussion of the possible origins of viruses will be highly speculative, though when we come to mechanisms of virus evolution we will be on firmer ground as sequencing of virus genomes enables the construction of phylogenetic trees and the monitoring of virus evolution as it occurs. Virus evolution is of great importance in a number of areas of applied virology, including the emergence of new pathogenic viruses (Chapter 22) and the development by viruses of resistance to drugs (Chapter 26).
21.2 ORIGINS OF VIRUSES
Viruses by definition are parasites of cells, so there could be no viruses until cells evolved. There is evidence for the presence of living cells about 3.9 billion years ago (Figure 21.1), though the first cells probably came into existence several hundred million years earlier. It is believed that, prior to the development of these primitive prokaryotes, there was a phase of evolution involving organic molecules. These molecules probably included proteins and RNAs, and some of the latter may have evolved capacities for self-replication.
Figure 21.1 Timeline of the Earth’s history. The approximate times when various groups of organisms first appeared are indicated.
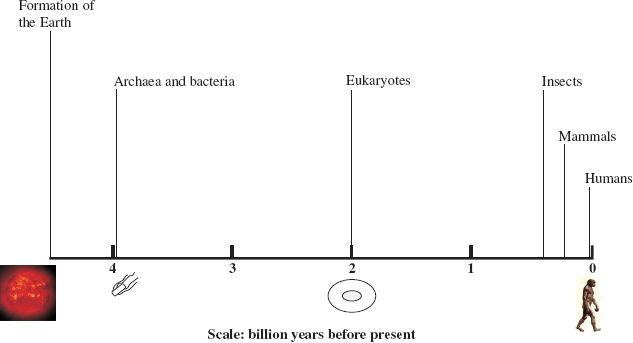
The archaea and the bacteria that inhabit the earth today are the prokaryotic descendants of the early cells. It is likely that viruses developed at an early stage in these primitive prokaryotes, but the extent to which the viruses of the modern prokaryotes (Chapter 20) resemble these early viruses is unknown. Eukaryotic cells appeared much later in evolution (Figure 21.1), so on the evolutionary timescale the viruses that infect eukaryotes are much more recent than those of the prokaryotes. The viruses of modern mammals and birds are presumably descended from those that infected their dinosaur ancestors.
The answer to the question “Where did viruses come from?” is “Basically, we do not know!” We can, however, speculate about possible ancestors of viruses; these include molecular precursors of cellular organisms when the earth was young, components of cells, and intracellular micro-organisms. We shall consider each of these in turn.
21.2.1 Molecular precursors of cellular organisms
It is thought that on the early Earth, before cellular organisms had come into existence, RNA molecules underwent evolution, developing enzyme activities (ribozymes) and the ability to replicate. Once cells had evolved perhaps some were parasitized by some of these RNA molecules, which somehow acquired capsid protein genes. If this happened then these were the first viruses.
21.2.2 Components of cells
Perhaps some cellular components evolved abilities to replicate themselves, independent of host cell control, and thus became parasites of those cells. Potential candidates for precursors of viruses include mRNA molecules and DNA molecules such as plasmids and transposons (Figure 21.2).
The genomes of many plus-strand RNA viruses of eukaryotic hosts have characteristics of eukaryotic mRNA. For example, the 5′ end of the genome is capped in tobamoviruses, the 3′ end is polyadenylated in picornaviruses, while the genomes of coronaviruses are both capped and polyadenylated. The plus strands in the dsRNA genomes of reoviruses are also capped and polyadenylated. Could some RNA viruses be descendants of cell mRNAs?
Covalently closed circular DNA molecules known as plasmids are found in prokaryotic and eukaryotic cells. Could some DNA viruses be descendants of such molecules? Some bacterial plasmids carry genes that specify the production of a protein tube (a sex pilus) that can attach to other bacterial cells and allow the transfer of a copy of the plasmid from one cell to the other. Could some phages, such as the filamentous phages or some of the tailed phages (Chapter 20), be descended from ancient plasmids and their sex pili? On the other hand it has been suggested that the sex pili of modern bacteria may have originated from filamentous phages.
Transposons are sequences of mobile DNA in the genomes of prokaryotes and eukaryotes. They are described as mobile because they can move from one part of the genome to another, usually by a “cut and paste” mechanism. Perhaps some DNA viruses are descendants of transposons.
For any of these DNA or RNA molecules to evolve into a virus the putative virus genome would somehow have to acquire a range of genes, including those for capsid protein(s) and, in many cases, for a polymerase to replicate the virus genome.
21.2.3 Intracellular micro-organisms
There is strong evidence that the mitochondria and chloroplasts of eukaryotic cells are derived from prokaryotic cells that adopted new modes of life within the cells of host organisms. It is assumed that the ancestors of these organelles adopted parasitic or mutually beneficial modes of life in host cells, and that over time they became increasingly dependent on their hosts, losing the ability to perform various functions, and losing the genes that encode those functions. Perhaps a similar evolutionary process continued further, leading to greater degeneracy and loss of functions such as protein synthesis, until the intracellular intruder was no longer a cell or an organelle, but had become a virus.
A virus that may have arisen in this way is the mimivirus (Section 1.3.1). The 1.2 Mb genome of this virus encodes a wide variety of proteins, including enzymes for polysaccharide synthesis and proteins involved in translation; it also encodes six transfer RNAs.
21.2.4 How did viruses originate?
The answer to this question is that we do not know! The great variety of virion structures, and of virus genome types and replication strategies, indicates that viruses had multiple origins. Small simple viruses, such as parvoviruses and picornaviruses, perhaps evolved from molecular precursors, while some large complex viruses, such as the mimivirus, perhaps evolved from cellular precursors.
We noted in Chapter 3 that the viruses found in certain categories of organism have a predominance of certain types of genome: dsDNA genomes in prokaryote viruses, ssRNA genomes in plant viruses, and dsRNA genomes in fungal viruses. We can now note that certain structural types of virus are restricted to particular categories of host: naked, rod-shaped ssRNA viruses are restricted to plant hosts, while viruses with a head–tail structure are rarely found outside the prokaryotes. The reasons for these distributions presumably concern diverse origins of the viruses in these very different hosts.
There is one feature, however, that is universal throughout the virus world: icosahedral symmetry. There are icosahedral viruses with dsDNA, ssDNA, dsRNA, and ssRNA genomes, and icosahedral viruses infect bacterial, archeal, fungal, plant, and animal hosts. These viruses have evolved from a variety of origins, but evolution has chosen the icosahedron for capsid design because it is a particularly stable structure.
One final thought on virus origins: we must entertain the possibility that today new viruses are continuing to evolve from molecular and cellar precursors.
21.3 EVOLUTION OF VIRUSES
In his book On the Origin of Species (Figure 21.3), Charles Darwin presented concepts of over-reproduction and survival of the fittest in terms of animals and plants. These same concepts can also be applied to viruses. (It can also be argued that the myriad exquisite viral structures entitles viruses, as well as plants and animals, to be included in the “. . . endless forms most beautiful and most wonderful . . .”) Like a cellular organism, a virus has genes that strive to perpetuate themselves. The expression of the combination of genes that constitutes the genome permits the virus to replicate itself. Changes to individual genes and new gene combinations continually throw up new genotypes, most of which are less successful than the parental genotypes and do not survive. Occasionally, however, a new genotype is more successful than the parental genotype and might even supplant it. Sometimes a new virus genotype enables the infection of a new host species.
21.3.1 Viruses from the past
The reverse transcription–polymerase chain reaction (RT–PCR) has been used to recover and amplify genomes of at least two RNA viruses from the past: influenza A virus from the 1918–19 pandemic (see Sections 16.4 and 16.5) and tomato mosaic virus. The latter virus is very stable and it can be detected in the atmosphere. Its presence in Arctic ice was predicted, and when cores of ice between 500 and 140 000 years old were examined, the RNAs of 15 strains of tomato mosaic virus were detected.
“Virus fossils” can also be found as DNA sequences in the genomes of plants, animals and bacteria. These sequences originated from integrations of viral sequences into the genomes of the ancestors of these organisms, and in many cases only frag-ments of the original sequences remain.
Examples of virus sequences in cell genomes include those of caulimoviruses in plants, flaviviruses in mosquitoes, and hepadnaviruses in birds. Mammalian genome sequencing has uncovered sequences originating from a wide range of DNA and RNA viruses, including retroviruses. The retroviral sequences are known as endogenous retroviruses (Section 17. 6) and are the likely sources of retrotransposons (Section 21.3.5.a).
21.3.2 Monitoring virus evolution
Evolutionary relationships between viruses can be found by comparing their genome sequences. The relationships can be portrayed in phylogenetic trees (Section 10.2.1; Figure 21.4). Genome sequencing can also be used to follow changes in rapidly evolving viruses, such as influenza A virus and HIV-1.
Figure 21.4 Phylogenetic tree showing relationships between primate lentiviruses. The tree is based on pol sequences. Simian immunodeficiency viruses (SIVs) are denoted by the name of the host species in black. Human immunodeficiency viruses (HIVs) are indicated in red. AGM: African green monkey. RCM: red-capped mangabey.
Source: Adapted from Müller and De Boer (2006) PLoS Pathogens, 2 (3): e15.
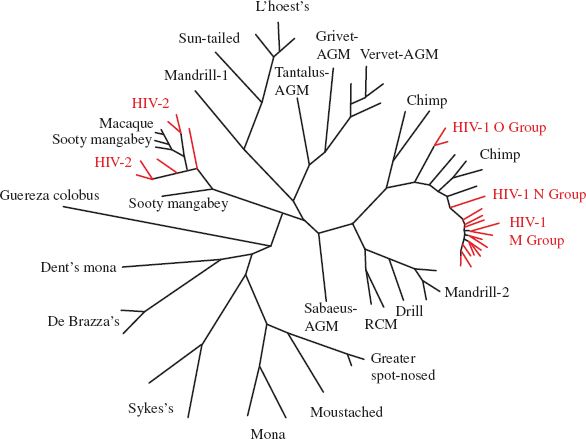
21.3.3 Mechanisms of virus evolution
In many respects, the underlying processes that drive virus evolution are the same as those that drive the evolution of cellular organisms. These processes involve the generation of genome variants, the vast majority of which are deleterious and do not survive, but a few provide an advantage in a particular niche. For a virus the niche might be a new host species or the presence of an anti-viral drug, and a variant may proliferate in that niche as a new virus strain. Virus genome variants arise as a result of mutations, recombination, reassortment of genome segments, and acquisition of cell genes. Some viruses are able to undergo two or more of these processes. We shall consider each process in turn.
21.3.3.a Mutations
When nucleic acids are copied by polymerases some errors are made. If an error is in a protein-coding sequence and if the error results in a change in the amino acid encoded, then the error results in a mutation. Natural selection operates and the mutations that survive are those that best fit the virus for its continued survival.
There are many selection pressures on viruses. These pressures include the immune response of the host; for example, a new antigenic type of an animal virus that the host’s immune system has not previously encountered is at an advantage compared with antigenic types against which the host has acquired immunity. There is, therefore, heavy selection pressure on virus proteins (e.g. HIV-1 gp120) that are targets of the host’s immune response, and these proteins tend to be the least conserved. Those regions of the proteins that are targets for neutralizing antibodies are especially variable. There are, however, constraints on the evolutionary process. Attachment proteins must retain the configurations that enable them to bind to cell receptors, and enzymes, such as reverse transcriptases, must retain their catalytic abilities.
For some viruses there are additional constraints. A virus such as potato yellow dwarf virus, which needs to replicate in both a plant host and an insect host, can tolerate a mutation that enhances its replication in the insect host only if the mutation does not compromise its ability to replicate in the plant host, and vice versa.
When virus DNA genomes are replicated, the error rates are much lower than when RNA genomes are replicated (Figure 21.5). This is because DNA-dependent DNA polymerases have a proofreading mechanism that corrects most of the errors, whereas RNA polymerases and reverse transcriptases have no such mechanism. As a result, DNA viruses, such as papillomaviruses, evolve much more slowly than RNA viruses, such as picornaviruses.
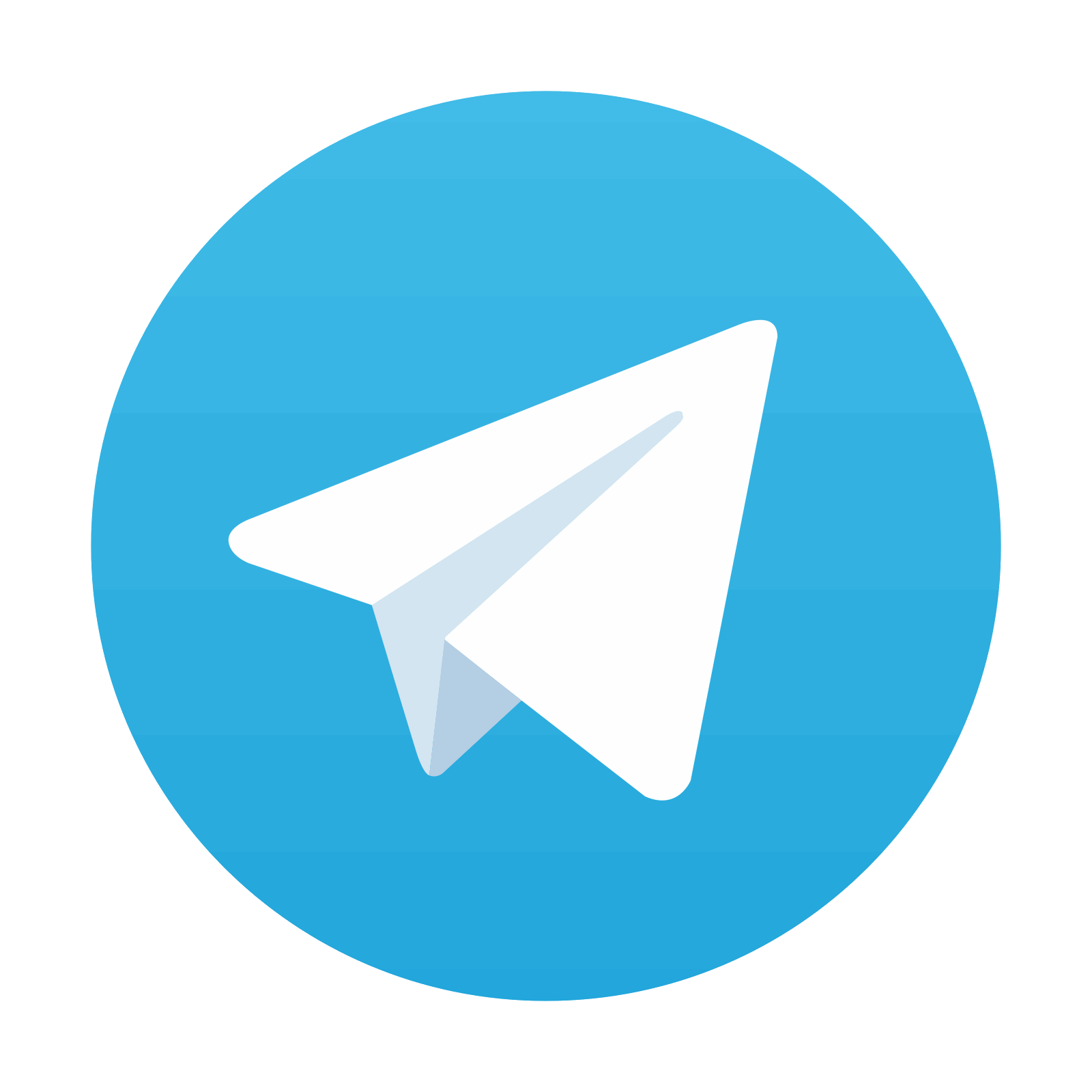
Stay updated, free articles. Join our Telegram channel

Full access? Get Clinical Tree
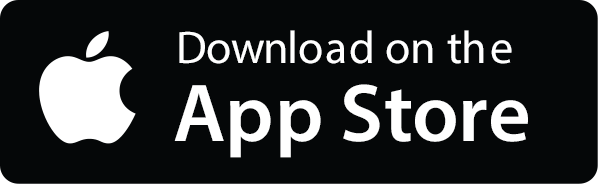
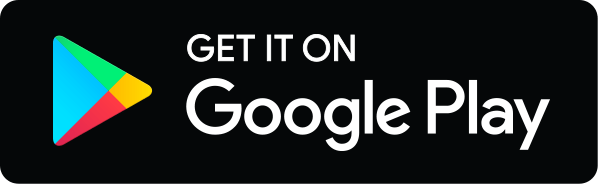