Fig. 1
Cartoon diagram of the photoactivatable Rac (PA-Rac). A LOV domain and its C-terminal Jα helix are fused to the N-terminus of a constitutively active mutant of Rac1 and block its interaction with downstream effectors (e.g., PAK) in the dark. Upon illumination, the Jα helix dissociates from the LOV domain and unwinds, restoring PAK binding and activation
2 Materials
2.1 DNA Plasmids for PA-Rac and Its Controls
The majority of the DNA constructs used in the current protocol can be obtained from the addgene website (www.addgene.org, search for reagents from Dr. Klaus Hahn’s laboratory) or from our lab upon request (Table 1). PA-Rac contains the following protein sequences: LOV(404-546)-Rac(4-192, Q61L/E91H/N92H). Q61L is a constitutively active mutation in Rac that renders it GTP hydrolysis deficient. The mutations E91H and N92H were introduced empirically to disrupt Rac-GAP interactions. Overexpression of the LOV-Rac fusion that harbors only the Q61L mutation led to apparent membrane ruffles in cells in the dark, consistent with an elevated background of Rac activation. We speculated that this is due to a dominant-negative effect of this earlier construct on endogenous Rac because it may sequester GAP proteins. The GAP-binding mutations in PA-Rac resulted in improved binding of effector PAK in the light yet substantially reduced the background Rac activity in the dark.
Table 1
Available constructs of PA-Rac from addgene website or upon request
Vector: | pTriEx-4 (transient transfection) or pBabe-TetCMV-puro (stable cell line and inducible expression) |
Fluorescent protein tags: | mCerulean, mVenus, or mCherry |
Modifications of the LOV domain: | WT (photoactivatable), C450A and C450M (dark), or I539E (lit) |
Modifications of Rac1: | Q61L/E91H/N92H (active) or T17N (dominant negative) |
We generated PA-Rac constructs with various fluorescent protein tags, including mCerulean, mVenus, and mCherry, to facilitate the identification of cells expressing PA-Rac in transient experiments. Based on the excitation wavelengths of these fluorescent proteins, mCherry (~590 nm) can be monitored without concern for excitation of the LOV domain. The standard commercial excitation filters for mVenus pass light below 500 nm which can activate the LOV domain. As a result, we recommend using different, somewhat suboptimal, excitation filters that blocks transmission below 510 nm, together with imaging routines that use attenuated excitation intensity and brief exposure time, to avoid unintended photoconversion of the LOV domain. Because the excitation wavelength of mCerulean (433 nm max) falls in the photoactivation spectrum, fluorescence imaging of mCerulean is best used for confirmation of expression at the end of experiments.
For transient transfections, plasmids in pTriEx-4 vector (Novagen) should be used. Alternatively plasmids in tetracycline-inducible expression (Tet-Off) retroviral vector pBabe-TetCMV-puro can be used to generate cell lines that have stable expression. With retroviral gene delivery, lower but more uniform expression of PA-Rac can be achieved, which is beneficial in obtaining consistent photoactivation responses among different cells. The inducible expression system is of favor because of the lack of expression of PA-Rac in cells in the presence of low concentration of doxycycline in the medium. The cells can be maintained and passaged in the light without worrying about unintended photoactivation. We recommend using the included fluorescent protein tag to gauge expression level, determining optimum expression for light-induced Rac activation with minimal background activity in the dark. The expression level, if needed, can be further controlled through titration with different concentrations of doxycycline. Alternatively, populations of stable cells with optimal expression level can be isolated using fluorescence activated cell sorting (FACS).
2.2 Cell Culture, Transfection, and Retroviral System
1.
HeLa, mouse embryonic fibroblast (MEF), and LinXE cells are maintained in high-glucose DMEM supplemented with 10 % fetal bovine serum (FBS), GlutaMAX, and Pen/Strep (Invitrogen). For convenience, we use a prior established Tet-Off MEF cell line (Clontech) that stably expresses tetracycline transactivator (tTA). When the Tet-Off cell line is not available, coinfecting other murine cells with retroviral particles produced from the plasmid pRev-Tet-Off (Clontech) may be necessary. The Tet-Off MEFs are maintained in above culture medium with 100 μg/ml of G418 for drug-resistance selection of the tTA gene. LinXE is a HEK293-derived retroviral producer cell line. It stably expresses packaging and envelope proteins for the production of ecotropic, MoMuLV-based retroviruses that infect dividing murine (mouse and rat) cells. LinXE cells are maintained in above medium with 100 μg/ml of hygromycin.
2.
X-tremeGENE (Roche) and Opti-MEM I reduced serum medium (Invitrogen) are needed for transient transfection.
3.
Medium 199 without phenol red (Invitrogen) or F-12K nutrient mixture without phenol red (custom made from Invitrogen) is used in epifluorescence imaging.
4.
25 mm round coverslips that are cleaned with detergent and by sonication. After extensive rinsing, the coverslips can be stored in 70 % ethanol in a sealed jar.
5.
A stock solution (10,000×) of doxycycline (Clontech) at 100 μg/ml is prepared for switching off protein expression in Tet-Off MEFs and a stock solution (1,000×) of puromycin (Gold Biotechnology) at 10 μg/μl for selection of stable cells after retroviral infection.
6.
2× HBS (HEPES-buffered saline): 50 mM HEPES, 280 mM NaCl, and 1.5 mM Na2HPO4, adjust pH exactly to 6.95 with HCl. Sterile filter the solution through 0.2 μm filter and store at 4 °C.
7.
2 M CaCl2. Filter sterilize through 0.2 μm filter and store at 4 °C.
8.
0.45 μm PES filter and 5 ml syringes with luer lock.
9.
Polybrene (Sigma) stock solution (1,000×) at 6 mg/ml.
Other common reagents for cell culture can be obtained from Invitrogen. Additional reagents for specific imaging applications will be described in Subheading 3.
2.3 Illumination Control and Microscope Customizations
Localized illumination with precise computer control can be accomplished using most laser scanning confocal microscopes, and applications using PA-Rac on these systems should be relatively straightforward (see Note 1 ). Alternatively, localized illumination can be achieved using most conventional wide-field microscopes that have a field stop/diaphragm in place or can be modified to incorporate a pinhole in a conjugate image plane. The broad-spectrum light source used for epifluorescence excitation can be used to illuminate a small region of the cell. Commercial vendors have devised a variety of add-on solutions for laser irradiation of small portions of the field of view, usually for FRAP studies. A laser beam can be coupled into the light path and focused on the focal plane to a diffraction-limited spot or dilated to bigger areas through z offset. These spots can also be mobilized to scan across different shapes either manually or using galvanometer-driven mirrors. Complex patterns can be achieved using digital micromirror-based devices such as the commercially available Mosaic active illumination system (Andor) or more advanced methods based on liquid-crystal spatial light modulators, realized in applications involving laser tweezers [17] and adaptive optics [18]. We opted for the Mosaic active illumination system because of its ability to simultaneously illuminate multiple regions of interest and fast switching time. One of the concerns with this method is insufficient intensity output. Because the LOV domain has a reasonable extinction coefficient (ε 449 = 12, 200 mol−1 cm−1) but high quantum yield (0.44) [19], we found that even collimated consumer LED light source (<1 W output) can induce membrane protrusion in live cells upon brief (seconds), localized illumination. We found that recent versions (TE2000 and Ti) of Nikon inverted epifluorescence microscopes are convenient for such applications because they have a “stage-up” design (see Note 2 ), proven advantageous over systems from other microscope manufacturers. The stage-up design allows an ideal incorporation of the Mosaic system so that epifluorescence excitation and Mosaic active illumination can be incorporated independently in the infinity space between objective and camera. Two motorized turrets for filter blocks also permit rapid and independent control of filter settings for imaging and photoactivation (see Fig. 2). Additional components needed for the customization of such an imaging system are:
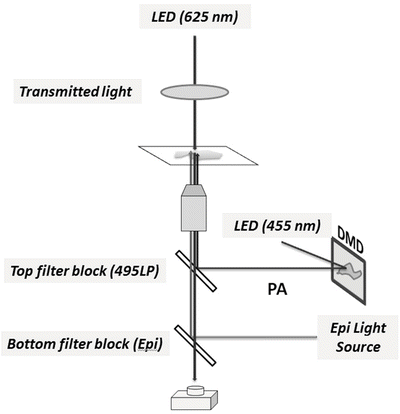
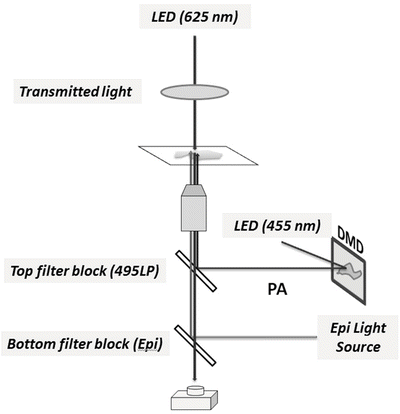
Fig. 2
Schematic diagram of customization of an epifluorescence microscope for application of PA-Rac. A digital micromirror device (DMD) is used to generate active spatial patterns of illumination. The DMD is coupled to the image conjugate plane of the microscope and reflects a collimated light beam from a high-power LED source (455 nm) which activates the LOV domain. An additional dichroic mirror (495LP) is inserted in the infinity space of the conventional epifluorescence imaging path to incorporate the activation light beam. The transmitted light source, typically halogen, is replaced with a red light-emitting (625 nm) high-power LED to avoid activation of the LOV domain during acquisition of DIC images
1.
LED light source to be mounted on the Mosaic active illumination system: a high-power LED (Thorlabs) at 455 nm wavelength, with a power output rated at 900 mW, and with a beam collimator and an adapter to the Mosaic system.
2.
Modification of transmitted light: replace the halogen light source with a high-power LED (Thorlabs) at 625 nm wavelength, with a power output rated at 440 mW, and with a beam collimator and an adapter to the transmitted lamp house.
3.
LED DC current drivers with TTL inputs (Thorlabs) and a DAQ board for shutter-free switching of LEDs using TTL outputs (NI, USB-6259).
4.
A 495LP dichroic mirror is installed in the top filter block to reflect the photoactivation beam “shaped” by the Mosaic system to the samples while passing excitation and emission at longer wavelengths during image acquisition. Because the LEDs have well-confined spectrum range (~20 nm full width at half maximum (FWHM)), it was unnecessary to use cleanup filters in front of the LEDs in our hands.
We also installed a small CO2 incubator near the microscope in the darkroom and yellow or red consumer LEDs (PAR38) for darkroom lightings for convenience in sample handling.
3 Methods
3.1 Transient Expression of PA-Rac in HeLa Cells
1.
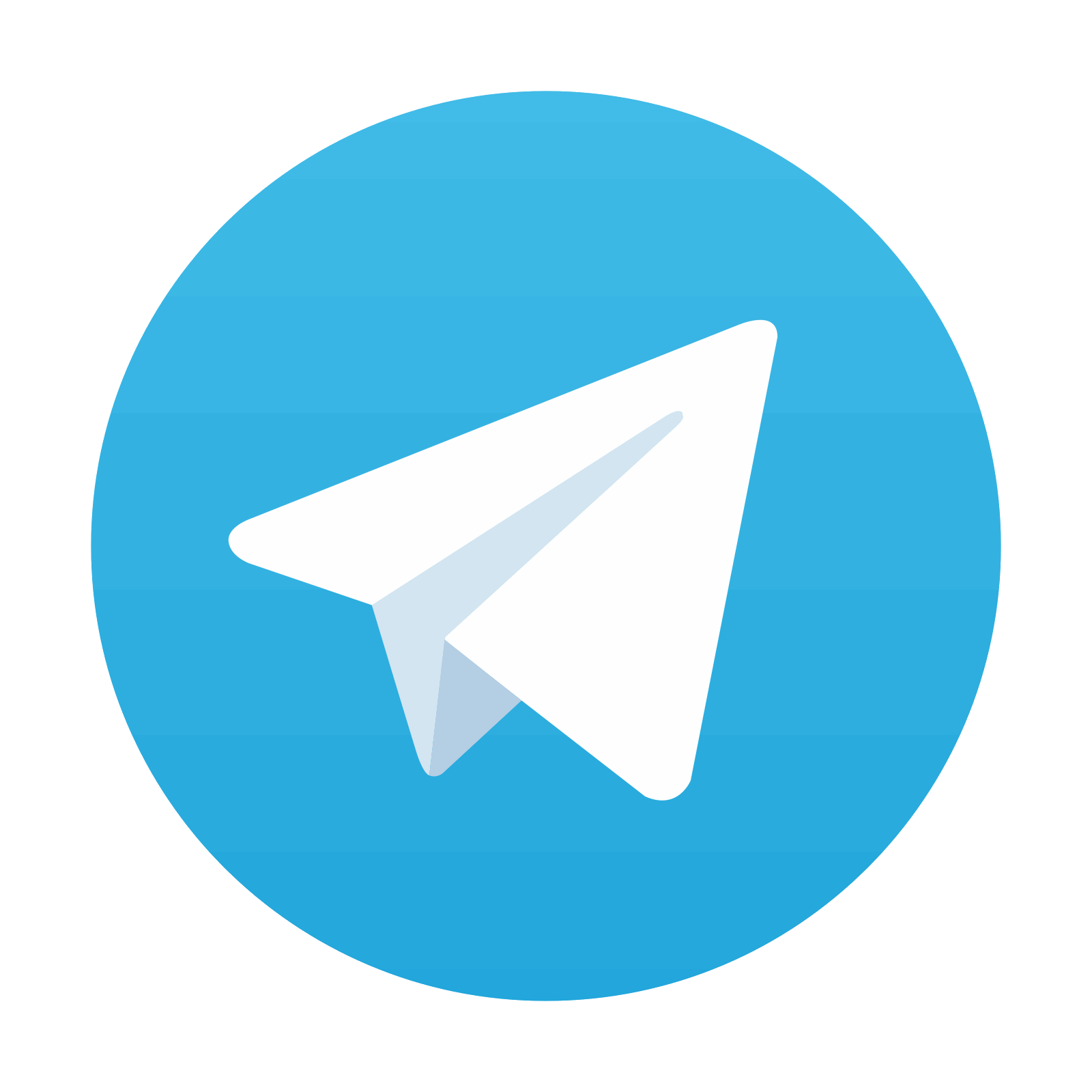
Day 1: trypsinize a well-maintained stock of HeLa cells, and seed the cells at 30–50 % confluency in a 35 mm culture dish in the morning. The cells should adhere and spread within a few hours and will be ready for transfection at the end of the day.
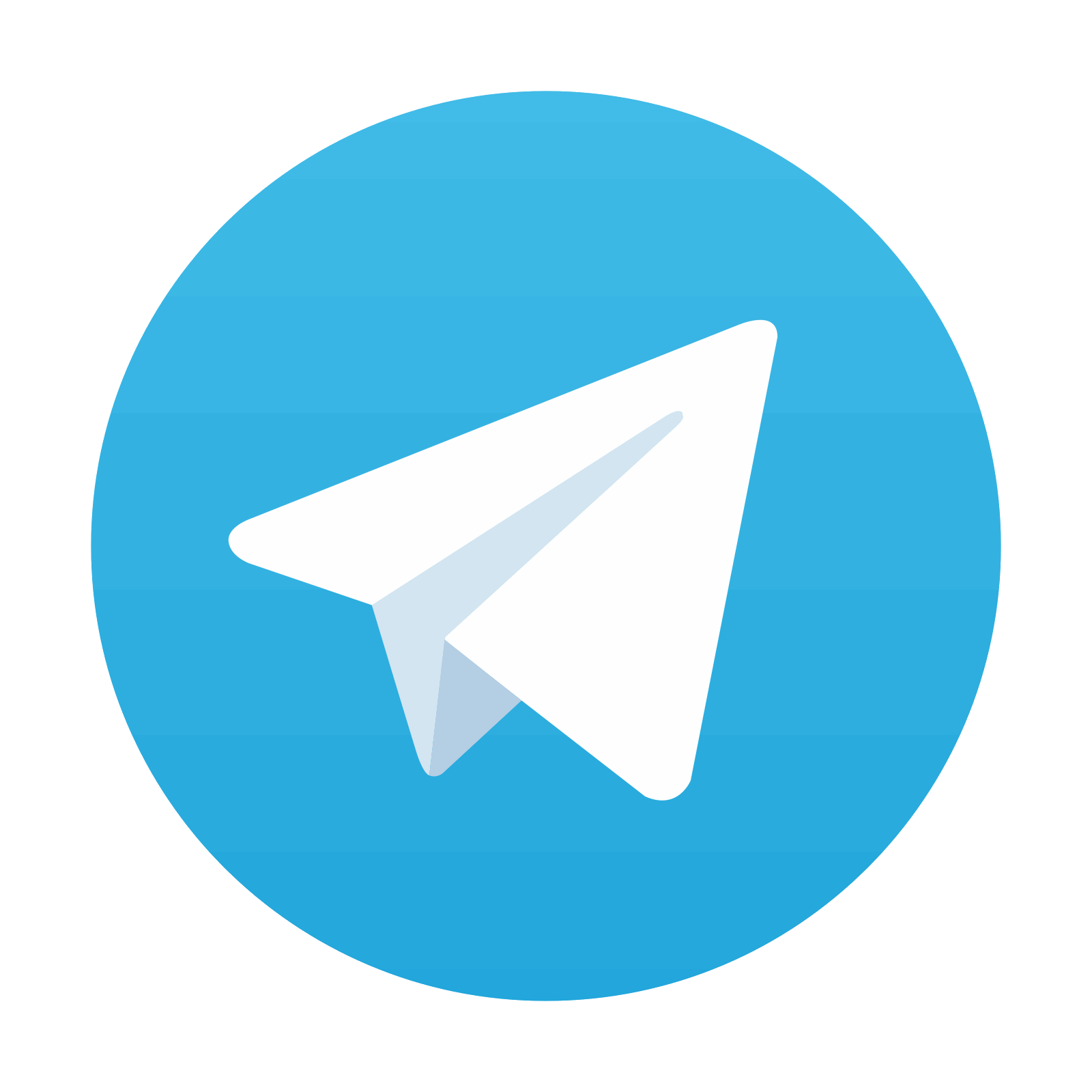
Stay updated, free articles. Join our Telegram channel

Full access? Get Clinical Tree
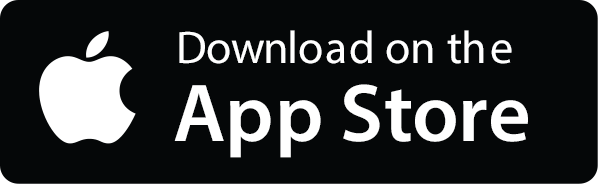
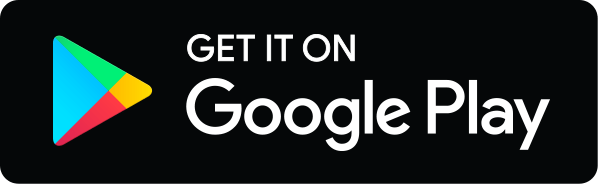
