CONTENTS
13.2 Anatomy and Physiology of the Eye: Implications for Ophthalmic Drug Delivery
13.2.2 Limiting Factors for Ophthalmic Delivery
13.2.2.1 Visibility Requirements
13.2.2.2 Tear Film, Nasolacrimal Drainage, and Reflex Lacrimation
13.2.2.3 Epithelial Permeability Barriers
13.2.2.4 Other Physical Barriers of the Eye
13.3.1 Diseases of the Anterior Cavity
13.3.2 Diseases of the Vitreous Chamber and Retina
13.4 Overview of Drug Delivery to the Eye
13.5 Traditional Topical Drug Delivery
13.6 Advanced Topical Delivery
13.6.2 Contact Lenses and Cul-de-Sac Inserts
13.6.3 Use of Micro- and Nanoparticulate Drug Delivery Systems
13.7 Intravitreal Drug Delivery
13.7.1 Intravitreal Injections
13.7.1.1 Intravitreal Injections for Anti-VEGF Drugs
13.7.1.2 Intravitreal Injections for Gene Therapy
13.7.1.3 Long-Acting Intravitreal Injections
13.8 Transscleral Drug Delivery
13.8.2 Iontophoresis and Electroporation
13.8.3 Microelectromechanical Systems
Vision problems constitute a major burden to society, although some sight-threatening diseases (such as cataracts) are now largely successfully treated. The leading causes of blindness and low vision in the Western world are primarily diseases of the elderly population, including age-related macular degeneration (AMD), diabetic retinopathy, and glaucoma. As the population demographic changes, treating these diseases becomes increasingly important as a health-care priority.
Effective drug delivery to the eye is extremely challenging, and unlike the other epithelial routes described in this book, ophthalmic drug delivery is used only for the treatment of local conditions of the eye and is not considered as a portal of drug entry to the systemic circulation. Significant advances have been made to optimize the localized delivery of medication to the eye, so that the route is now associated with highly sophisticated drug delivery technologies, some of which are unique to the eye. We begin this chapter with a consideration of the anatomical and physiologic barriers of the eye and the concomitant limitations they impose for successful ophthalmic delivery. The pathology of the most important ophthalmic diseases is described, as well as their location in the eye, as this has important implications for the delivery route chosen. This is followed by a discussion of the various routes of ophthalmic drug delivery and the associated drug delivery and targeting technologies for each route.
13.2 ANATOMY AND PHYSIOLOGY OF THE EYE: IMPLICATIONS FOR OPHTHALMIC DRUG DELIVERY
The function of the eye is to produce a clear image of the external world and to transmit this to the visual cortex of the brain. In order to do this, the eye must have constant dimensions, an unclouded optical pathway, and the ability to focus light on the retina. These requirements, and the need for protection of the globe, determine the special structure of the eye and its associated physiological responses.
The eye is a spherical fluid-filled structure composed of three layers: (1) the sclera/cornea, (2) the uvea, and (3) the retina (Figure 13.1). The sclera, the tough outer “white” of the eye, is a layer of dense connective tissue that covers the entire eyeball, except for the cornea. Its stiff nature helps maintain the globe’s shape, making it more rigid, and protecting its inner parts. Extraocular muscles attach to the sclera through tendons, allowing the eye to move. The cornea is a transparent avascular layer at the front of the eye (FOTE), which covers the colored iris. Its curved structure helps to focus light onto the retina (Elkington and Frank 1999).
The anterior cavity (anterior to the lens; FOTE) is subdivided into two chambers: (1) the anterior chamber, between the cornea and the iris, and (2) the posterior chamber, which lies behind the iris, but in front of the lens. The lens is composed of proteins called crystallins, arranged like the layers of an onion; it lacks blood vessels and is completely transparent. Both chambers of the anterior cavity are filled with circulating aqueous humor, described further in the following texts.
The vitreous chamber (posterior to the lens; back of the eye [BOTE]) is the larger, posterior cavity of the eye. It contains a clear, jellylike, viscoelastic gel, the vitreous humor, which is formed during embryonic development and is not replaced thereafter. The character of this gel changes through life, becoming less structured, and therefore more liquid, in the elderly.
FIGURE 13.1 Sagittal section of the eye.
The second layer is the uvea or uveal tract, which is composed of the choroid, iris, and ciliary body. The choroid is highly pigmented (the melanin limits reflections within the eye) and contains many blood vessels that supply the retina. Anteriorly, the choroid meets the iris and the ciliary body. The iris is the thin, pigmented structure in the eye, the shape of a flattened doughnut, responsible for controlling the diameter of the pupil and thus the amount of light reaching the retina. The ciliary body comprises both the ciliary muscle and the ciliary processes. Contraction of the ciliary muscle relaxes the zonular fibers, resulting in a change in the curvature of the lens and facilitating fine focus (accommodation).
The ciliary processes are capillary-rich protrusions on the internal surface, which produce and secrete the aqueous humor. This transparent, slightly alkaline fluid contains less protein and glucose than plasma, but more ascorbic acid, an important antioxidant (Civan and Macknight 2004). Aqueous humor nourishes the lens and cornea, carrying oxygen and nutrients and removing waste, as it circulates through the anterior cavity. It subsequently drains at sclerocorneal trabecula into Schlemm’s canal, to be resorbed into the blood. Normally, aqueous humor is completely replaced every 90 minutes.
The third layer of the eye is the retina, which contains a pigmented layer and a neural layer. The neuroretina comprises three distinct layers of neurons, interconnected by synapses: the photoreceptor layer, the bipolar cell layer, and the ganglion cell layer (Figure 13.2). Visual data are transduced by the photoreceptors and processed through the other neural layers before being transmitted as nerve impulses along the axons that form the optic nerve. There are two types of photoreceptor: rods (especially important for “gray” night vision) and cones (daytime vision). The larger cones only make up 5% of the total population of visual receptors and thus the retina is dominated by rods (about 100 million in man). The 5–6 million cones comprise S-type cones detecting blue light, M-type cones most sensitive to green light, and L-type cones detecting red light. The central retina, known as the macula, has a high density of cones, thus allowing for highresolution central vision.
FIGURE 13.2 Microscopic structure of the retina.
13.2.2 LIMITING FACTORS FOR OPHTHALMIC DELIVERY
A considerable number of anatomical and physiological barriers exist to protect the eye from the entry of foreign substances. These barriers act as major limiting factors for ophthalmic drug delivery, which will be discussed in the following texts. Further reviews of the barrier properties of the eye are discussed in Wilson and Tan (2012) and Mains and Wilson (2013).
13.2.2.1 Visibility Requirements
More than half the sensory receptors in the human body are located in the eyes and a large part of the cerebral cortex is devoted to processing visual information. Transduction of the light energy into a receptor potential occurs in the outer segment of rods and cones. Visual signals are subsequently transmitted through the neuronal cell layers to the retinal ganglion cells, which provide retinal output to the brain. Light must have an unobstructed path to reach the photoreceptors; subsequent neuronal processing and nerve transmission should also be unimpeded.
The eye then affords a particularly challenging route for drug delivery, as a drug delivery system (DDS) cannot interfere with this vital sensory role. For example, other epithelial routes such as the skin, or oral cavity, can use sustained-release technology such as an adhesive patch to provide drug release for a prolonged period of time. The situation is difficult for the eye as even if the device is transparent and well tolerated, any resulting refractive change would cause blinking, possible lacrimation, and attempted removal. This places design limitations for a DDS in the central axis of vision. Similar limitations are encountered within the eye. Delivery systems formulated as colloids and particulates such as nanoparticles and liposomes can be used for controlled release and drug targeting via many epithelial routes. However, within the eye, there is a risk that movement of fluid would transport particulate systems posteriorly, where they could adhere to the lens, causing opacification, or appear as free-floating objects in the visual path, scattering light and decreasing visual acuity.
The extreme sensitivity and delicacy of the eye is a further difficulty and contrasts with more robust drug delivery sites such as those of the skin or the oral cavity. For example, the cornea is one of the most sensitive surfaces in the body, containing 300–600 more pain receptors than the skin (Belmonte and Cervero 1996). Thus, some chelating agents and surfactants, widely explored at high concentrations as a means of enhancing epithelial permeability via the oral, nasal, pulmonary, and buccal routes cannot be used in ocular topical preparations.
13.2.2.2 Tear Film, Nasolacrimal Drainage, and Reflex Larimation
The tear film comprises a three-layer structure: an inner mucin layer produced by conjunctival goblet cells that adheres to the cornea, a middle aqueous layer secreted by the lacrimal gland, and an external lipid layer produced by meibomian glands of the eyelid (Figure 13.3).
In the physiological process of nasolacrimal drainage, the tear film spreads over the surface of the eyeball due to the blinking of the eyelids. The tears are then cleared away as rapidly as they are produced, draining first into the lacrimal canals, then the lacrimal sac, and finally into the nasal cavity (Figure 13.4). The tear film and the process of nasolacrimal drainage combine to protect the eye by removing foreign material and form the first optical surface. Tears also lubricate and moisten the eyeball and carry nutrients to the cornea and conjunctiva. Each blink partially redistributes the tear film over the surface of the cornea and conjunctiva, and nutrients are replenished.
This process has important implications for drug delivery to the eye surface. Any topical medication must be retained on the surface of the eye despite a constant flow of tears and continuous eye blinking. In practice, nonviscous topical medications are rapidly cleared by this mechanism: up to 90% of the dose administered from conventional eyedrops is lost in the first 15 seconds after administration. Clearance by nasolacrimal drainage results in drug loss and also raises the possibility of systemic side effects, due to drug absorption through the mucous membrane of the nasolacrimal duct. This represents a particular concern for drugs such as the beta-blockers used in the management of glaucoma: unwanted systemic absorption can result in adverse cardiovascular and respiratory effects.
Under normal conditions, the human tear volume is about 7–9 μL and is relatively constant. The maximum amount of fluid that can be held in the lower eyelid sack is 15–20 μL, but only 3 μL of a solution can be incorporated in the precorneal film without causing it to destabilize. Eyedrop administration results in a sudden increase in tear volume, causing rapid reflex blinking. The volume of a drop is typically 33–38 μL and consequently some of the eyedrop can be spilled on the cheeks and eyelashes, resulting in drug wastage. Further, a large proportion of the retained instilled volume is pumped into the nasolacrimal duct, resulting in drug loss and the risk of systemic side effects.
FIGURE 13.3 The structure of the tear film.
FIGURE 13.4 Lacrimal flow and drainage. The lacrimal gland produces tears, which are distributed by blinking. At normal rates of flow, tears are cleared by nasolacrimal drainage. Sudden increases produced by instillation, or reflex tearing, overspill the lower fornix and roll over the cheek. (Modified from Blamb/Shutterstock.com.)
Any irritation of the eye surface will induce a reflex lacrimation and blinking, as a protective mechanism. Again, this has implications for topical drug delivery, in particular for the formulation of topical products. The exposure of the eye surface to an acidic fluid may cause protein denaturation, forming insoluble complexes. Alkalization of the tear film tends to cause a saponification of lipids due to an interaction of the hydroxyl ions with cell membranes. At a high pH, alkalis behave as hydrophobic species and are more damaging to the cornea than acids (since precipitation of protein limits further ingress). Mildly acidic solutions are therefore better tolerated than slightly alkaline solutions, and reflex tears neutralize the irritant solution by dilution but will also wash away the instilled drug.
13.2.2.3 Epithelial Permeability Barriers
The eye presents sequential epithelial barriers, which reduce flux of nonnutrients into the eye, as a further natural defense mechanism against the entry of hazardous agents. As described in detail in Chapter 4 (Section 4.3), drug permeation across epithelial barriers is generally restricted to two main routes: the paracellular route (between adjacent epithelial cells) and the transcellular route (across the epithelial cells). The presence of tight junctions with resistances in excess of 120 Ωcm2 between the epithelial cells of the eye severely restricts paracellular transport, confining the route to very small, hydrophilic molecules. The alternative, transcellular transport, is a viable transport pathway for drugs that possess the requisite physicochemical properties to allow for passive diffusion across many layers of epithelial cells. The physicochemical properties that favor transcellular diffusion are broadly the same as those affecting transepithelial absorption at any site and have been discussed in Chapter 4. In summary, favorable transport properties for transcellular passive diffusion include high lipid solubility and partition coefficient, low molecular weight and compact molecular volume, absence of charge, aqueous solubility, and chemical stability.
In medicines development through the turn of the century, massive jumps were made in the production of materials from cell sources rather than by the chemical synthetic route. As described in other chapters, these new “biologics” include peptide and protein drugs, as well as DNA-based therapeutics. For the eye, an early interest was in agents that restricted the uncontrolled growth of immature vessels following retinal ischemia—notably compounds that regulated expression of vascular endothelial growth factor (VEGF) and gene vectors. Such compounds had already appeared in cancer compound selection, since anti-VEGF molecules restrict the growth of solid cancers. All the drugs in this group are large and polar and therefore tend to have very low flux across membranes. Usually, they must be introduced by intravitreal injection, as the epithelial barriers restrict their penetration to the BOTE (see Section 13.7.1.1).
The principal epithelial barrier to drug transport in the eye was thought to be the cornea, but since it makes up only 20% of the outer eye area in contact with an instilled drop, the conjunctiva must also play a significant role in the absorption process. The conjunctiva is a thin, transparent mucous membrane composed of highly vascularized, nonkeratinized, stratified, columnar epithelium, which is approximately 5–15 cell layers thick. It also contains goblet cells that help lubricate the eye by producing the mucous component of the tear film. The conjunctiva lines the inside of the eyelids (tarsal conjunctiva) and the anterior one-third of the eyeball, apart from the cornea (bulbar conjunctiva). The tight junctions and multilayered nature of the conjunctiva make it an important barrier against infection but also limit the permeation of topically applied drugs. Since the conjunctiva is highly vascularized, significant drug loss to the systemic circulation can occur, resulting in drug wastage and the risk of systemic adverse effects.
The adult cornea measures 11–12 mm horizontally and 9–11 mm vertically. Corneal thickness varies, being thinnest centrally (550 μm) and gradually increases to the periphery (700 μm). It is an avascular five-layered structure comprising (anteriorly to posteriorly) the epithelium, Bowman’s membrane, stroma, Descemet’s membrane, and endothelium (Figure 13.5).
The outer corneal epithelium of the cornea constitutes the most important barrier for corneal drug flux. It comprises five to six layers of nonkeratinized, stratified, squamous epithelium, with an abundance of tight junctions. As introduced earlier, the tight junctions form a highly resistant barrier to paracellular transport, so that transport across the cornea is usually limited to the transcellular route. The inner stroma layer of the cornea (about 90% of the thickness of the cornea in most mammals) is composed of a modified connective tissue, rich in collagen, other proteins, and mucopolysaccharides. About 70%–80% of the wet weight is water, which presents a barrier to highly lipophilic drugs. The innermost layer of the cornea is a monolayer of endothelium, but this has leaky endothelial junctions that do not impede drug transit.
13.2.2.4 Other Physical Barriers of the Eye
Deep to the outer corneal and conjunctival epithelial barriers, the eye presents further obstacles to drug transport.
The sclera: The sclera is a layer of dense connective tissue, consisting primarily of collagen and elastin fibers, as well as proteoglycans, embedded in an extracellular matrix. It is thinnest at the site of extraocular muscle attachment (0.3 mm) and thickest at the posterior pole (1 mm). The essentially hydrophilic proteoglycan extracellular matrix can be expected to impede the diffusion of hydrophobic drugs through its tissue. Positively charged drugs may also interact with negatively charged proteoglycans and thus show reduced flux.
The retina: The retinal pigment epithelium (RPE) comprises a monolayer of cuboidal epithelial cells, lining the back of the retina (Figure 13.2). Tight junctions between the RPE cells form a blood/retina barrier and limit the access of drug molecules from the blood circulation Descemet’s membrane endothelium to the retina. The RPE also contains several efflux pumps including P-glycoprotein and multidrug resistance–associated protein, which further limit the passage of compounds into the vitreous. The inner retina receives a blood supply from the central retinal artery, which passes with the optic nerve into the eye. These blood vessels are lined with tightly packed endothelial cells thus forming the inner limiting membrane (ILM). The barrier in the inner globe is the meshwork of ILM and neuronal cells.
FIGURE 13.5 The corneal epithelial barrier.
Vitreous humor: The vitreous is a viscoelastic gel composed of Type II collagen fibers, which trap coiled hyaluronic acid and water molecules. As the volume of adult human vitreous is around 4 mL, the vitreous humor presents a large diluting sink. Additionally, the network of collagen and hyaluronic acid presents a diffusional barrier, limiting the passage of cells and macromolecules, particularly cationic molecules and cationic carriers, due to charge interactions.
The eye is prone to a wide variety of diseases, which may be of a systemic origin, such as diabetes or hypertension, or peculiar to the eye, such as glaucoma, cataract, and macular degeneration. Many of these conditions, such as macular degeneration, glaucoma, and diabetic retinopathy are chronic conditions that require continuous therapy for treatment. Furthermore, since the eye is located on the surface of the body, it is also easily injured and infected. According to the location of the disease, ocular disorders are grouped as disease of the anterior cavity or vitreous chamber.
13.3.1 DISEASES OF THE ANTERIOR CAVITY
Dry eye is a common problem, estimated to affect over 4 million Americans (Schein et al. 1997) and its incidence increases with age. Dry eye will result if the composition of tears is changed, or an inadequate volume of tears is produced. Dry eye conditions are not just a cause for ocular discomfort, but prolonged dry eye can lead to corneal damage, keratitis, and compromised vision.
A variety of different inflammatory eye conditions are described, according to the region of the eye affected. For example, blepharitis is an inflammation of the eyelid, most commonly caused by a bacterial infection, usually Staphylococcus aureus. Conjunctivitis is an inflammation of the conjunctiva, resulting in redness to the visible white of the eye. Allergic conjunctivitis (AC) is defined as inflammation of the conjunctiva in response to an allergen. AC may be seasonal or perennial. Primary symptoms are itching, burning, and then a watery discharge. Bacterial conjunctivitis can result in pain and a purulent discharge, while viral conjunctivitis results in a clear discharge. Trachoma is an infectious cause of conjunctivitis caused by the organism Chlamydia trachomatis. The resulting chronic granular inflammation causes a fibrosis of the conjunctival lid surface, resulting in inversion of the lid margin. Subsequent abrasion from eye lashes results in keratitis and can lead to blindness unless treated; it is the most common cause of blindness in North Africa and the Middle East (Burton and Mabey 2009). Keratitis is an inflammation or infection (bacterial, viral, or fungal) of the cornea. Patients with keratitis present with decreased vision, ocular pain, foreign body sensation, red eye, and often a cloudy/opaque cornea.
Glaucoma refers to a group of different eye conditions, in which damage to the optic nerve leads to progressive, irreversible, vision loss. It is sometimes categorized as a stand-alone disease, separate from the posterior segment diseases, since it is treated by local topical therapy to the anterior eye. It is considered one of the major global ophthalmic clinical problems; it is the second leading cause of blindness, after cataracts, worldwide. In the United States, primary open-angle glaucoma (POAG) is the most common form of the disease, accounting for 90% of all cases. It occurs mainly in the over 50-year-olds and is characterized by an increase in the intraocular pressure (IOP) caused by a restriction of outflow and decreasing oxygenation. The associated nerve damage involves loss of retinal ganglion cells. Visual loss develops first in the peripheral visual field and then progresses to the central visual field. POAG is a painless, insidious disease in which injury develops over years. Symptoms are absent until extensive optic nerve damage has been produced. If the condition is left untreated, blindness will result.
13.3.2 DISEASES OF THE VITREOUS CHAMBER AND RETINA
Intraocular infections are those infections of the inner eye, including the aqueous humor, iris, vitreous humor, and retina. They may occur after ocular surgery, trauma, or may arise from systemic infections. Intraocular infections carry a high risk for loss of vision and may result in the spread of infection from the eye into the brain.
Uveitis refers to an inflammation of the anterior uvea (that portion of the eye including the anterior chamber, iris, and ciliary body) and surrounding tissues. In addition, a posterior segment component (retinochoroidal) may be involved. Uveitis may arise due to an autoimmune disorder, following an infection, or other causes. If untreated, inflammation in the anterior segment may lead to glaucoma and cataracts, while inflammation in the posterior segment may lead to macular edema.
Cytomegalovirus retinitis (CMVR) is an inflammation of the retina of the eye, which may lead to blindness. It is caused by an infection of human CMV (HCMV), a herpes-type virus. HCMV opportunistically infects patients with a weakened immune system; it came to the attention of the public with the appearance of HIV in the 1990s and may be masked by the concomitant use of corticosteroids. Much of the early research on ocular implants was stimulated by the urgent need to treat this disease.
AMD* is a degenerative disorder of the retina, typically in persons aged 50 years and older. Central vision becomes increasingly blurred, thereby limiting perception of fine detail, which is required for activities like reading, driving, recognizing faces, and seeing the world in color. The disease begins as the more common dry AMD (85% of patients) but can later progress to the second, much more severe form, known as wet AMD (neovascular AMD; 15% of patients), which is associated with acutely progressive vision loss.
In dry AMD, debris associated with the turnover of photoreceptors surrounds the most lightsensitive region of the retina, the macula lutea. The pigmented layer atrophies and degenerates, resulting in a gradual deterioration of central vision. In wet AMD, degeneration of the macula is due to the growth of new subretinal blood vessels, which are fragile and leaky; fluid leakage causes permanent macula injury.
The emergence of CMVR (as a consequence of HIV infection) in the late 1970s was a significant driver at the time for the development of new ocular delivery technologies such as ocular inserts. In a similar manner, a large section of current ophthalmic drug delivery research is driven by the need to achieve successful drug delivery to the BOTE in order to treat AMD. AMD remains a leading cause of irreversible blindness and visual impairment in the world (Agarwal et al. 2015). As many as 11 million people in the United States have some form of macular degeneration. Although previous estimates suggested that age is a prominent risk factor, with 2% risk of contracting the disease for those ages 50–59, to nearly 30% for those over the age of 75, the incidence of AMD in the United States is declining in each successive generation and adults born after World War II have a significantly lower risk of early disease progression (Cruickshanks et al. 2014).
Diabetic retinopathy is one of the major complications of diabetes and results from hyperglycemia-induced changes in the retinal microvasculature. Initially, the damage is limited to microaneurysms in the retinal capillary walls, which can leak fluid. It then progresses to scarring, followed by the proliferation of a new leaky vasculature, stimulated by VEGF. The overgrowth of new, unstable, leaky retinal capillaries reduces visual acuity. Diabetic macular edema (DME) is caused by leaking macular capillaries, which cause fluid and protein deposits to collect on, or under, the macula of the eye, causing it to thicken and swell, and distorting central vision. Retinopathy is accelerated by hyperglycemia, hypertension, and smoking.
13.4 OVERVIEW OF DRUG DELIVERY TO THE EYE
In the search for an efficient route for ocular drug delivery to the eye, various complex interrelated factors must be considered. These considerations include the limitations generated by the anatomy and physiology of the eye, the pathology and location of the ocular disease, and the accessibility of a site for drug delivery. Thus, a number of different approaches are possible, which are summarized in Figure 13.6 and described in detail in the following texts.
Topical delivery is useful in the treatment of local conditions of the surface of the eye, for example, conjunctivitis, but it is limited by the short residence time of conventional eyedrops. Mechanisms to enhance residence time include the incorporation of mucoadhesive polymers into eyedrop formulations, as well as the use of micro- and nano-DDS, contact lenses, and intraocular inserts.
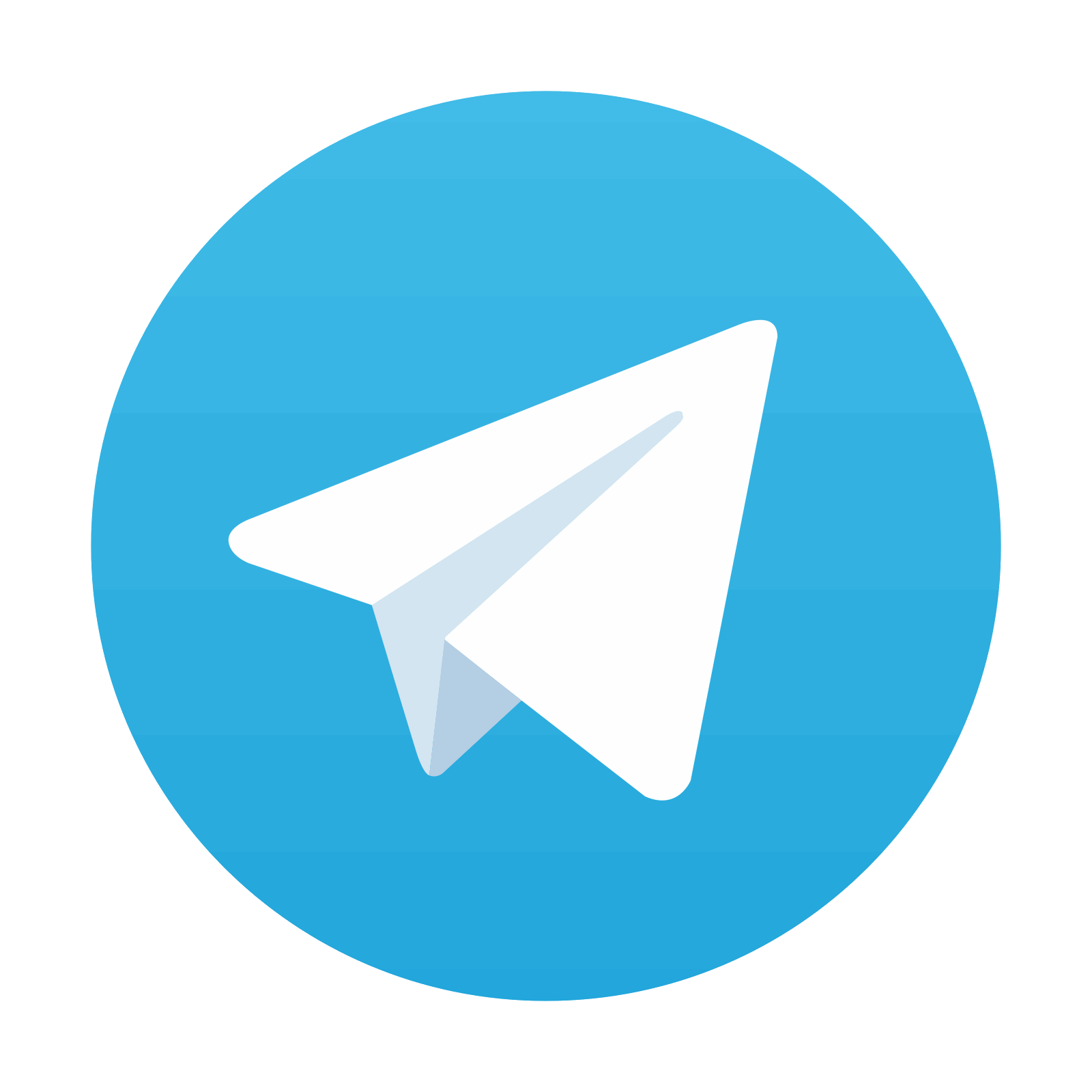
Stay updated, free articles. Join our Telegram channel

Full access? Get Clinical Tree
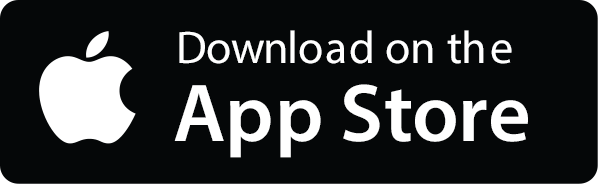
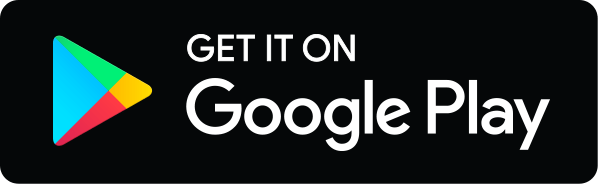