Management of Hyperlipidemia
T. D’souza and S.A. Mengi*
C.U. Shah College of Pharmacy, SNDT Women’s University,
Santacruz (West), Mumbai – 400 049, India
ABSTRACT
Throughout history humans have used plants as a remedy for various disease conditions. With the advent of modern synthetic molecules, plant medicine has often been relegated to the fringe of therapeutic modalities. However, it is increasingly being recognized that the current therapeutic options have several limitations especially in chronic disease conditions such as hyperlipidemia and atherosclerosis. Research is ongoing to discover newer drugs and several novel therapeutic targets are being explored. In this context there has been a renewed interest in plant research. Bioactives from plants such as garlic (Allium sativum), Guggul (Commiphora mukul), Soy (Glycine max), Citrus fruits and Psyllium (Plantago ovata) have demonstrated a potential in the treatment of hyperlipidemia. Further, the fact that today several plant bioactives have proved to act via known and well defined molecular pathways such as inhibition of microsomal triglyceride transfer protein (MTP), Acyl coenzyme A (CoA) : cholesterol acyl transferase (ACAT), Acyl coenzyme A (CoA) : diacylglycerol acyltransferase (DGAT) and Farnesoid X Receptors (FXR) has provided an added impetus to explore the untapped potential of medicinal plants. In the present review, the plant derived bioactives or natural products that have proved to have a potential in hyperlipidemia or whose molecular mechanism of action has been elucidated will be discussed, followed by an overview of the plants that have been investigated in this area in the past decade.
Keywords:Citrus bioactives, Garlic, Guggul, Hyperlipidemia, Investigative plants, Novel targets, Polyphenols, Soy protein.
Introduction
While contagious diseases dominated the early centuries, followed by the increased incidence of various forms of cancer, today we are witnessing a dramatic increase in cardiovascular related mortality. Cardiovascular disease (CVD) has assumed epidemic proportion across the globe. One of the major contributory factors to cardiovascular disease is the growing menace of hyperlipidemia and the resulting coronary artery disease (CAD). Clinical studies have consistently demonstrated that lipid-lowering therapy can halt and even reverse the progression of atherosclerosis and reduce CVD events (Lipid Research Clinics Program, 1984; Blankenhorn et al., 1993; Scandinavian Simvastatin Survival Study Group, 1994; Waters et al., 1994; Levine et al., 1995). Epidemiological studies have confirmed that hyperlipidemia has a central role in atherogenesis. It is of significance that studies have demonstrated that when total cholesterol levels were maintained below 160 mg/dl, CHD risk was markedly attenuated even in the presence of other risk factors (Grundy et al., 1998). This can be attributed to the fact that lipid lowering actively opposes the events occurring right from the genesis of the atherosclerotic plaque to its rupture.
Although there are highly effective drugs available in this area of therapeutics, research is ongoing to discover drugs that overcome the limitations of the existing therapy. New drug discovery continues to be a challenge and successful outcomes are becoming increasingly rare. Indeed, in the area of hyperlipidemia, experts believe that the research pipeline is weak (Opar, 2007). Dramatic lifestyle changes in the past few decades and the high levels of stress in modern life often necessitate the concomitant use of several drugs, leading to adverse effects that then require further drug intervention. This cascade of subjecting the body to several drugs results in stress on vital organ systems. In this context there has been a renewed interest in plant research. Plants can be likened to experienced synthetic chemists producing a wide range of biologically active compounds. Of the large number of higher plants it is estimated that only around 6 per cent have been screened for biological activity and 15 per cent have been evaluated phytochemically (Lahlou, 2007). The recognition of the potential of plant derived drugs is amply reflected in recent publications of prominent scientific journals both debating and discussing the scope of plants in drug discovery (Balachandran and Govindarajan, 2007; Lahlou, 2007; Littleton, 2007; Mukherjee et al., 2007). Further, the fact that today several plant bioactives have proved to act via known and well defined molecular pathways has provided an added impetus to explore the untapped potential of medicinal plants.
Lipid homeostasis is a complex process that is controlled mainly by events occurring at the intestine and the liver. Additionally, several nuclear receptors act as lipid sensors in these processes. Plant derived bioactives have proved to modulate various facets of lipid homeostasis at multiple levels. Further, plants are renowned for their potent antioxidant properties which contribute to the attenuation of atherosclerosis. An understanding of these factors requires a description of the events occurring at the intestine and liver and identification of the potential therapeutic targets.
Intestinal Absorption of Lipids
Dietary lipid comprises mainly of neutral fat or triglycerides, phospholipids, sterols like cholesterol, some minor lipids and fat-soluble vitamins. In order for the virtually insoluble lipids in the intestine to be absorbed, the large aggregates of dietary lipids need to be broken down into smaller droplets. The bile acids produced by the liver and secreted into the intestine are amphipathic molecules that effectively emulsify the dietary lipids into micelles resulting in the solubilization and transport of lipids in the aqueous environment of the intestine. The micelles are cylindrical in shape with the polar lipids arranged radially with their hydrophilic heads facing outward toward the aqueous phase (Figure 14.1A) (Hofmann, 1999). As the intestinal contents are mixed, micelles come in contact with the brush border of small intestinal enterocytes, and the lipids are taken up into the epithelial cells.
Absorption of Dietary Triglyceride by the Intestine
Dietary triglycerides (triacylglycerols) are hydrolyzed in the gut by the action of pancreatic lipase to fatty acids and monoacyl glycerol which are then absorbed separately by the intestine. In the enterocyte, the fatty acids and monoglycerides are re-esterified by the enzyme acyl-coenzyme A: diacylglycerol acyl transferase (DGAT) to form triglycerides (Figure 14.1B). Thus, pancreatic lipase inhibitors and DGAT inhibitors have a potential in the treatment of hypertriglyceridemia and obesity, wherein the absorption of dietary fats must be restricted (Shi and Burn, 2004; Chen and Farese, 2005).
Absorption of Cholesterol by the Intestine
Dietary cholesterol is mainly in the form of cholesteryl esters. In the intestine, these cholesteryl esters are acted upon by esterases, primarily secreted by the pancreatic exocrine glands. Intestinal cholesterol absorption is a multistep process that is regulated by the activity of several transporters and enzymes (Figure 14.1B). The cholesterol present in the micelles is taken up by the enterocytes via the Niemann-Pick C1 Like1 (NPC1L1) protein that is localized to the brush border of the jejunal enterocytes, which forms the interface between the intestinal lumen and the intracellular compartments (Altmann et al., 2004). The cholesterol thus absorbed into the enterocyte is then re-esterified to cholesteryl esters by the enzyme Acyl coenzyme A:cholesterol acyltransferase (ACAT), a process that is essential to the incorporation of cholesterol into lipoprotein particles. A fraction of the cholesterol that enters into the enterocyte is pumped back out of the enterocyte into the intestinal lumen by the ATP-binding cassette (ABC) transporter complex ABCG5/G8 (Figure 14.1B) (Duan et al., 2004). Thus modulation of the expression of NPC1L1, ACAT and ABCG5/G8 represents important new targets for cholesterol absorption inhibition.
Formation and Secretion of the Chylomicron on Particle by the Intestine
The triglycerides and cholesteryl esters formed in the enterocyte by the action of DGAT and ACAT respectively, are essentially insoluble in water and hence cannot be secreted or transported in the aqueous blood or lymph as free molecules. Microsomal triglyceride transfer protein (MTP) is responsible for the systematic addition of these triglycerides and cholesteryl esters to phospholipids and a protein moiety, Apolipoprotein B48 (ApoB48) to form a lipid and protein (lipoprotein) complex known as chylomicron (Figure 14.1C). MTP is a protein localized within the endoplasmic reticulum (ER) of the cells found in the intestine as well as the liver and is essential to the process of secretion of the atherogenic Apo-B containing lipoproteins by both the intestine as well as the liver (Swift et al., 2003; Hussain et al., 2003).Thus inhibitors of MTP are being investigated as a viable therapeutic option in the management of hyperlipidemia.
In the capillaries of the adipose tissue and muscle, the triglycerides of the chylomicrons are hydrolyzed by the enzyme lipoprotein lipase (Lpl) that is bound to the luminal surface of the endothelial cells. The liberated fatty acids cross the endothelium and enter the underlying adipocytes or muscle cells where they are either esterified again to triglycerides for storage or oxidized to provide energy. The triglyceride depleted chylomicron remnant is taken up by the liver via the LDL receptor or the LDL receptor related protein (LRP). The chylomicron particle then undergoes digestion in the lysosomes to release free cholesterol in the hepatocytes.
Figure 14.1:Intestinal Absorption of Lipids
(A) Solubilization of dietary lipids via interaction with bile acids to form mixed micelles.
(B) In the enterocytes, the monoacylglycerols and fatty acids are re esterified by the action of DGAT to form triglycerides while cholesterol is converted to cholesterol esters by the action of ACAT. Some of the unesterified cholesterol returns to the intestinal lumen via the ABCG5/G8 efflux transporter complex.
(C) Chylomicron particles are formed by the packaging of triglycerides, cholesteryl esters and apolipoproteins in a process mediated by MTP and secreted into the lymph.
Role of the Liver in Lipid Homeostasis
The liver is a multifunctional organ that plays a key role in cholesterol homeostasis by mediating both, the synthesis of cholesterol and the secretion of lipoproteins as well as the clearance of these lipoproteins from the circulation. The free cholesterol supplied by the chylomicron particles to the liver is esterified by the action of ACAT and packaged along with ApoB100 and triglycerides by the action of MTP in a process similar to that occurring in the intestine. This results in the secretion of VLDL particles (which are precursors to LDL) into the circulation. The secretion of VLDL particles by the liver is governed by several factors including substrate availability viz. the availability of cholesteryl esters and triglycerides as well as the functionality of ACAT and MTP (Sniderman and Cianflone, 1993; Hussain et al., 2003; Rudel et al., 2005). Besides the cholesterol brought to the liver via endocytosis of lipoproteins from the circulation, the liver is also capable of endogenous biosynthesis of cholesterol from acetate (Figure 14.2). This occurs in a multi step process in which the rate limiting enzyme is 3-hydroxy 3-methyl glutaryl coenzyme A (HMGCoA) reductase.
The VLDL particles secreted by the liver are triglyceride rich particles that undergo LpL mediated depletion of triglycerides in the circulation to yield LDL particles. LDL has a vital role to play in delivering cholesterol to the various tissues of the body mainly for cellular membrane synthesis and steroid and hormone production. However, excess LDL is taken up by macrophages present in the subendothelial region of the vasculature to form lipid engorged macrophages known as foam cells which is the hallmark of the atherosclerotic lesion.
The liver is the only organ capable of excreting cholesterol out of the body wherein nonpolar cholesterol is degraded by the action of the hepatic enzyme, cholesterol 7α-hydroxylase, to the polar, water soluble bile acids that are then secreted back into the intestine. Additionally, the regulation of hepatic LDL-receptor expression and cycling are part of a complex process that regulates the removal of cholesterol from the blood stream (Brown and Goldstein, 1986). Approximately 75 per cent of the circulating LDL is removed by the liver in this fashion. This accounts for the tremendous success of the pharmacological modulation (upregulation) of the LDL-receptor.
Figure 14.2:Endogenous Biosynthesis of Cholesterol by the Liver
Further, the liver also plays an important role in reverse cholesterol transport. HDL particles perform the important function of transferring excess cholesterol from the vasculature and other cells back to the liver in the process termed as reverse cholesterol transport (Figure 14.3). The cholesteryl esters picked up by the HDL particle is presented to the liver via either a direct or an indirect pathway. HDL particle picks up cholesterol from the peripheral tissues and macrophages/foam cells. This cholesterol can be transported to the liver via two mechanisms: Cholesteryl ester transfer protein (CETP) mediates the exchange of cholesterol from the HDL particle to LDL and triglyceride rich lipoproteins (TRLs) i.e. The apoB containing lipoproteins. These particles are taken up by the liver via the LDL receptor. The direct pathway involves the selective uptake of cholesteryl esters by the liver via the SR-B1 receptors without endocytosis or breakdown of the HDL particle (Trigatti et al., 2003). The core of the HDL particle is effectively depleted of cholesteryl esters and then released into the circulation for further interaction with the lipid laden macrophages (foam cells). Thus, the liver serves as an acceptor of cholesterol carried by the HDL particles, improving the functionality of the reverse cholesterol transport process. The cholesterol thus delivered to the liver becomes available for conversion to bile acids and excretion into the intestine.
Figure 14.3:Transport of Cholesteryl Esters from the Peripheral Tissues and Macrophages to the Liver
HDL particle picks up cholesterol from the peripheral tissues and macrophages/foam cells. This cholesterol can be transported to the liver via two mechanisms:
(1) The Indirect pathway: this involves CETP mediated transfer of cholesteryl esters from HDL to Apolipoprotein B containing lipoproteins such as VLDL and their subsequent uptake by the LDL receptors of the liver.
(2) The Direct pathway: the HDL particle directly transfers its cholesteryl esters to the liver via interaction with the SRB1 receptors.
Role of Oxidized Lipoproteins in Atherosclerosis
Atherosclerosis can be defined as a complex multifactorial disease characterized by the presence of lesions due to the accumulation of lipids in the walls of large and small arteries accompanied by a loss of elasticity of the affected arteries (Singh et al., 2002). Several research workers have demonstrated that the oxidation of lipids, more specifically the oxidation of LDL, plays a central role in these events (Witztum, 1994). The oxidative modification of LDL has several ramifications and actively stimulates atherosclerotic plaque development and rupture by promoting inflammatory processes (Figure 14.4) (Libby, 2002). It has been proposed that inhibiting this process could significantly attenuate the atherogenecity of the lipids and hence antihyperlipidemic agents possessing antioxidant activity is considered desirable.
Figure 14.4:Schematic Representation of the Activating Effect of LDL Infiltration and Oxidative Modification on Inflammation in the Artery
Oxidatively modified LDL aids in the recruitment of monocytes into the subendothelial space (intima) by s timulating th e end oth elial c ells to exp ress ad hes io n molecu les s uch as VCA M-1 and chemoattractants such as MCP-1. It is also readily taken up by the macrophages to form the lipid laden foam cells that release a variety of proinflammatory mediators such as cytokines, ROS, MMPs and growth factors.
VCAM: Vascular cell adhesion molecule-1, MCP: Monocyte chemoattractant protein, ROS: Reactive oxygen species, MMPs: Matrix metalloproteinases.
Nuclear Receptors Acting as Sensors in Lipid Homeostasis
The homeostatic mechanisms that regulate lipid metabolism require cellular sensors that can monitor and coordinate the enzymatic cascades and events involved in lipid synthesis and catabolism. Nuclear receptors are transcription factors that are present in abundance in the various metabolic organ systems and have the capacity to regulate genes involved in lipid and energy metabolism directly in response to varying nutrient availability. Nuclear receptors playing a prominent role in lipid metabolism and atherosclerosis include the Peroxisome proliferator activated receptors (fatty acid sensor), the Farnesoid X receptor (bile acid sensor) and the Liver X receptor (sterol sensor) (Figure 14.5) (Shulman and Mangelsdorf, 2005). Screening of compounds as ligands for these receptors has gained tremendous momentum over the past two decades. The potential for obtaining such compounds from plants has been recognized by studies indicating that Guggulipid, a plant derived antihyperlipidemic compound, mediates its effect via the farnesoid X receptor (Urizar et al., 2002).
Plants with Potential in the Management of Hyperlipidemia
Plants have served as a source of medicinal agents for thousands of years and have the distinct advantage of having been used empirically in the treatment of a wide variety of disease conditions. However, the demands of modern medicine (and rightly so) requires the evaluation of these plant bioactives using scientific methodologies and assays in order to gauge their potential in the management of the disease. In pursuit of this objective, several plant derived extracts and bioactives have been subjected to extensive preclinical and clinical studies. In the arena of hyperlipidemia, some of these plant bioactives have consistently demonstrated a potential to be used as therapeutic agents. Moreover, studies elucidating their mechanism of action have provided further proof of concept. These findings though, have not been without exception and controversies. In the following section an update will be provided on the status of these bioactives so as to be able to gauge their future potential.
Garlic: Bulbs of Allium sativum
Garlic and its preparations have been used by people of diverse cultures for thousands of years and are mentioned in several texts of traditional medicine as having an application in the treatment of cardiovascular disorders. The historical perspective of the therapeutic use of garlic and its scientific evaluation has been extensively reviewed (Rahman, 2001; Banerjee and Maulik, 2002). The most extensively studied preparation of garlic is the raw garlic homogenate or its aqueous extract, presumably because this is the commonest way garlic is consumed. Garlic oil, dried garlic powder (Kwai) and aged garlic extract (Kyolic) are other popular garlic preparations that have been investigated for therapeutic efficacy. Aged garlic extract (AGE) is prepared by soaking sliced raw garlic in aqueous ethanol solution for up to 20 months at room temperature a procedure that renders the garlic free of the odorous sulfur principles. The procedure is mentioned in the United States Pharmacopoeia/National Formulary monograph under Garlic Fluid Extract (Macan et al., 2006).
Garlic is one of the most well studied plant products in the treatment of hyperlipidemia (Yeh and Liu, 2001, Thompson et al., 2006). In preclinical studies, long term (2-9 months) feeding of garlic and garlic preparations (2 per cent garlic powder in diet) to rabbits fed a high-cholesterol diet resulted in a statistically significant reduction in atherosclerotic lesions. The chronic effects (> 4 weeks) of garlic (1-4 per cent in diet) and garlic protein on lipid metabolism in rats revealed a reduction in serum cholesterol, triglyceride and LDL cholesterol. Total lipid content and cholesterol levels in the liver were also decreased indicating that inhibition of the enzyme HMGCoA reductase could be one of the mechanisms of action. An excellent review of these studies has been compiled by Banerjee and Maulik (2002). Studies also indicate that garlic can attenuate hypercholesterolemia induced vascular alterations and prevent LDL oxidation, a process known to play a central role in atherogenesis (Slowing et al., 2001; Lau, 2006). Moreover, since hypercholesterolemic patients are also often prescribed anticoagulants for associated complications and garlic has been alleged to predispose to platelet dysfunction, studies have been conducted to investigate the interaction of the aged garlic extract (AGE/Kyolic garlic), a popular garlic preparation and warfarin. The results of the study concluded that there was no synergistic effect and AGE can be safely administered with warfarin (Macan et al., 2006).
Figure 14.5:Regulation of Cholesterol and Lipid Handling in Metabolic Organ Systems by Nuclear Receptors
Reproduced from Shulman and Mangelsdorf, 2005)
The complex nature of the components of garlic as well as the tendency of some of these components to degrade depending on the method of preparation has made it a challenge to assign the therapeutic effects of the various preparations to any one chemical constituent (Amagase, 2006). The chemistry of the Allium species is highly complex, predominantly comprising of sulfur-containing compounds that are responsible for the characteristic flavor of garlic. S-allyl-L-cysteine sulfoxides (alliin), g-glutamyl-S-allyl-L-cysteines and (1)-S-(trans-1-propenyl)-L-cysteine sulfoxide are some of the abundantly present sulfur compounds. These sulfoxides are converted into thiosulfinates such as allicin (purported to be the active component) by the action of the enzyme alliinase when raw garlic is cut or crushed. Additionally, garlic also contains several volatile as well as water soluble organosulfur compounds. S-methyl cysteine sulfoxide obtained from another Allium species viz. Allium cepa (Onion) also significantly ameliorated the hyperlipidemic condition in rats maintained on a high cholesterol diet (Kumari and Augusti, 2007). Additionally, a variety of components including nonsulfur compounds such as steroidal saponins are believed to contribute to the therapeutic efficacy of garlic (Amagase, 2006).
Among the several mechanisms of action that have been proposed for garlic, it is interesting to note that both in vitro as well as in vivo studies indicate that inhibition of microsomal triglyceride transfer protein (MTP), is one of the mechanisms of action (Lin et al., 2002). MTP is responsible for the packaging of triglycerides and cholesteryl esters with apolipoprotein B in the intestine and the liver to form chylomicrons and VLDL particles respectively. While MTP is the therapeutic target of several new synthetic molecules in the research pipeline, a notable hurdle in developing MTP inhibitors is that this effect in the liver leads to accumulation of fat (steatosis) as these agents block the assembly of VLDL but not the substrate (triglyceride and cholesteryl ester) accumulation in the liver (Burnett and Watts, 2007). Hence, it is encouraging to note that while in vitro studies indicate that fresh garlic decreases mRNA expression of MTP in both hepatic (HepG2) as well as intestinal (Caco-2) cell lines, in vivo studies in rats indicate that this effect is restricted to the intestine leading to increased excretion of fat (Lin et al., 2002).
While garlic seems to be one of the most promising plant-derived anti hyperlipidemic agents, there are also studies which have reported the various forms and constituents of garlic to be ineffective in hyperlipidemia (Espirito Santo et al., 2004). The results of clinical trials also demonstrate a dichotomy with respect to efficacy. While the majority of the clinical trials demonstrated a favorable effect on lipid profile, a meta-analysis of randomized clinical trials have indicated that while garlic was superior to the placebo, its effect was modest and its clinical efficacy doubtful (Stevinson et al., 2000). This observation has been countered by arguments that the cholesterol lowering activity of garlic is mainly due to allicin (which is formed from alliin by the action of the enzyme alliinase) when raw garlic is cut or crushed. On the other hand the clinical studies employed dried garlic/commercial preparations such as tablets that presume that a biologically significant amount of allicin is formed after consumption (Lawson, 2001).
This highlights the need for uniformity in the preparation and standardization of the various garlic preparations. Thus, while a plethora of evidence points to the therapeutic potential and health benefits of garlic consumption, the challenge lies in the development of a standardized and stable preparation that retains the therapeutic efficacy and safety.
Guggul: Gum resin of Commiphora mukul
Guggul is an extract from the resin of the myrrh tree Commiphora mukul. The medicinal use of guggul dates back to600 BC, when it was used for obesity, atherosclerosis, and variousinflammatory conditions. The plant sterols E- and Z-guggulsterone are believed to be the bioactive compounds. An ethyl acetate extract of this resin termed as guggulipid has been found to lower LDL cholesterol and triglyceride levels in clinical studies (Gopal et al., 1986). Guggulipid as a treatment has been developed mainly by research groups working in India. In the 1980s several clinical trials attested to the beneficial effects of guggulipid on the lipid profile (Agarwal et al., 1986; Gopal et al., 1986; Nityanand et al., 1989). It received regulatory approval in India in 1987 for use as a lipid-lowering drug and has been feted as an example of successful translation of knowledge from traditional systems of medicine (Ayurveda) to modern clinical practice.
Research on guggul received an additional boost by findings that indicated its mechanism of action to be via the farnesoid X receptors (FXR). A brief digression to highlight the role of FXR in lipid homeostasis is of order. The biosynthesis of bile acids from cholesterol by the action of the enzyme cholesterol 7α hydroxylase (CYP7A1) is the most significant pathway for the elimination of cholesterol from the body. FXR is referred to as a bile acid sensor and its activation by bile acids leads to suppression of bile acid synthesis by CYP7A1, increased biliary excretion, detoxification of bile acids and decreased import back into the hepatocytes (Figure 14.6) (Claudel et al., 2005). On the other hand, CYP7A1 expression becomes derepressed by antagonism of FXR. This leads to increased conversion of cholesterol to bile acids and subsequent upregulation of the LDL receptor with increased clearance of LDL from the plasma.
In 2002 studies demonstrated that guggulsterones strongly inhibit FXR activation by chenodeoxycholic acid (CDCA) which is the most potent of the bile acid agonist ligands by directly competing for the FXR ligand binding domain (Urizar et al., 2002). Simultaneously, other cell based assays confirmed that guggulsterones antagonize the bile acid receptor (Wu et al., 2002). Further, guggulsterone treatment decreased hepatic cholesterol in wild-type mice fed a high-cholesterol diet but was not effective in FXR-null mice indicating that its mechanism of action was FXR antagonism (Urizar et al., 2002). Subsequent studies however, have revealed that guggulipid also activates FXR controlled targets such as the bile salt export pump (BSEP), with a predominance of transactivation rather than antagonism of FXR, thereby indicating that it may be acting as a partial agonist to FXR (Cui et al., 2003; Deng et al., 2007).
Although its effect on FXR provides a rational and valid basis to the lipid lowering effects seen in the earlier clinical trials, in 2003, results of a double-blind, randomized clinical trial of guggulipid in an American population on a typical western diet, failed to demonstrate a beneficial effect on lipid profile. The investigators of the trial have suggested that guggulipid may have different lipid effects in different populations. A positive finding of the study was that guggulipid produced a marginal decrease in cardiovascular surrogate markers such as Lp(a) and CRP which have a role in the inflammatory component of atherosclerosis (Szapary et al., 2003). Considering all the evidence in totality, we can conclude that guggulipid is a plant bioactive with considerable potential. However, detailed clinical studies on a wider and more diverse population using guggulipid as well as guggul prepared by traditional methods could possibly provide conclusive evidence of the status of this bioactive in the management of hyplipidemia.
Figure 14.6: Role of FXR in the Regulation of Bile Acids Synthesis, Transport and Detoxification (Adapted from Claudel et al., 2005)
Cholesterol 7a-hydroxylase (CYP7A1) is the rate controlling enzyme of one of the pathways by which the liver converts cholesterol to bile acids. Activation of FXR by ligands/bile acids induces the expression of the atypical nuclear receptor small heterodimer partner (SHP). SHP represses CYP7A1 gene transcription thereby inhibiting bile acid synthesis. FXR also induces the expression of transporters such as the bile salt export pump (BSEP) and multidrug resistance-associated protein 2 (MRP-2) that enable the hepatocytes to secrete the synthesized bile acids into the bile canaliculi. On the other hand it inhibits the expression of the intestinal bile acid transporter (IBAT) which is responsible for the uptake of the bile acids from the lumen of the ileum back into the enterocytes. Further, FXR inhibits the reuptake of bile acids from the portal vein by inhibiting the expression of bile acid import transporters such as Na+ taurocholate cotransporting polypeptide (NTCP) and organic anion transporter polypeptides (OATPs).
Soy Protein and Isoflavones: Components of Soybean, Glycine max
Soybean (Glycine max) is a legume species native to East Asia and now cultivated throughout the world. Currently soy is one of the only foods approved by the US FDA (1999) that is allowed to advertise that it lowers cardiovascular risk.
In the past decade considerable interest has been generated in the use of soy products in the management of hyperlipidemia. Epidemiological studies have indicated that populations consuming a considerable amount of soy products demonstrated better plasma lipid profiles (Nagata et al., 1998; Ho et al., 2000). Several clinical trials have indicated that soy components, mainly soy protein, improve the lipid profile (Wong et al., 1998; Desroches et al., 2004; Taku et al., 2007). In contrast, there have also been clinical trials indicating that this effect is very marginal and cannot be substantiated (Sacks et al., 2006; Matthan et al., 2007). However, these investigators also agree that many soy products should be beneficial to cardiovascular and overall health because of their high content of polyunsaturated fats, fiber, vitamins, and minerals and low content of saturated fat as compared to protein from animal sources. In general, when dietary protein from animal sources is replaced by that obtained from plant sources, a beneficial effect on lipid profile is observed. In the case of soy protein, this effect has been attributed to its specific amino acid profile (Erdman, 2000). Animal experiments studying the effects of various essential amino acids on lipid profile indicate that while the amino acids lysine and methionine tended to be hypercholesterolemic, the amino acid arginine was hypocholesterolemic (Kurowska and Carroll, 1994). Hence it has been proposed that the higher arginine to lysine and methionine amino acid profile of soy protein may contribute to its hypocholesterolemic effects.
Soy also contains a significant amount of phytoestrogens viz. the isoflavonoids, genistein and daidzein and two saponins, soyasaponin A and B that contribute to its effects (Lee et al., 2005). Soy protein enriched with isoflavones compared with that depleted of isoflavones eg. by ethanol extraction, demonstrated a greater cholesterol lowering effect (Lucas et al., 2001; Taku et al., 2007). However, unexpectedly, clinical trials demonstrated that the isoflavonoid components themselves when consumed without its protein component were ineffective in lowering cholesterol and thus currently soy protein but not isoflavone pills have been recommended (Hodgson et al., 1998).
The mechanism of action of the cholesterol lowering activity of soy has been proposed to have several components (Figure 14.7). Studies have indicated that soy protein enhances the conversion of cholesterol to bile acids by the liver resulting in upregulation of LDL-C receptor and subsequent increase in clearance of LDL from the plasma. It has also been proposed that soy could increase thyroid hormones that modulate lipid metabolism (Potter, 1995).
Polyphenolic Plant Bioactives
Polyphenols are phytochemicals found in abundance in several legumes, berries, fruits such as grapes, apple and pear and vegetables such as broccoli, parsley, cabbage and celery. Additionally, polyphenols are also present in red wine, chocolate, green tea and olive oil.
In recent years the polyphenols have become the focus of much attention due to their potent antioxidant properties and possible health benefits in diseases ranging from cancer to coronary artery disease. The polyphenol class of compounds include flavonoids such as flavanols, flavones, isoflavones, anthocyanidins and proanthocyanidins, tannins such as theaflavins and gallic and ellagic acid esters as well as coumarins and stilbenes.
Figure 14.7: The Mechanism of Action of the Cholesterol Lowering Activity of Soy (Adapted from Potter, 1995)
Soy increases the conversion of cholesterol to bile acids. Consequently, hepatic cholesterol metabolism shifts to provide cholesterol for enhanced bile acid synthesis. As a result cholesterol biosynthesis as well as low density lipoprotein (LDL) receptor activity is increased. The net result is increased clearance of LDL from the plasma. Additionally, the uptake of bile acids and cholesterol from the intestine to the liver is also decreased.
Some of the polyphenols that have been investigated for their effect on hyperlipidemia and atherosclerosis include polyphenols isolated from grapes, red wine, tea, citrus fruits, apple and soy. Studies have indicated that several factors contribute to the lipid lowering effects of the polyphenols (Figure 14.8) (Zern and Fernandez, 2005).
Resveratrol
Resveratrol, a stilbene polyphenol is perhaps the most well known therapeutically active polyphenol. While it is often mentioned exclusively in context of Vitis vinifera (grape) species, it was first isolated from the roots of white hellebore (Veratrum grandiflorum) in 1940, and later, in 1963, from the roots of Polygonum cuspidatum, a plant used in Oriental medicine (Baur and Sinclair, 2006). In the early 1990s resveratrol shot into prominence as the compound responsible for the cardioprotective effects of red wine (the French paradox).
Figure 14.8: Pathways Contributing to the Lipid Lowering Effect of the Polyphenols (Adapted from Zern and Fernandez, 2005)
(1) Polyphenols decrease both MTP and ACAT activity involved in lipid assembly for VLDL secretion. (2) Without sufficient lipid components, the degradation of apo B-100 is increased. (3) The significant decrease in substrate availability results in a decrease in both VLDL synthesis and secretion. (4) The delipidation cascade is further altered due to the decrease in VLDL particles in circulation. (5) Alterations to the VLDL particle, including significant decreases in apo E, may induce an increase in LPL activity, and therefore, decrease plasma TG and VLDL concentrations. (6) CETP activity may also be affected by the decrease in VLDL substrate. (7) The VLDL decrease yields a significant decrease in LDL concentrations. Due to the significant alteration in hepatic cholesterol concentrations, the LDL receptor may be upregulated to maintain hepatic cholesterol homeostasis.
Resveratrol has a multi pronged effect, addressing several aspects of atherosclerosis viz. decreased platelet aggregation, vasorelaxation, decreased expression of adhesion molecules and cytokines, reduced lipid peroxidation and improved serum cholesterol and triglyceride concentrations (Zern and Fernandez, 2005; Baur and Sinclair, 2006). Additionally, the protective effect of red wine has also been attributed to other phenolic compounds such as gallic acid, catechin and quercetin.
Tea Polyphenols
The leaves of the tea plant Camellia sinensis are a rich source of polyphenols like catechins eg. (-)-epigallocatechin-3-gallate (EGCG), (-)-epigallocatechin (EGC), (-)-epicatechin-3-gallate (ECG), (-)-epicatechin (EC), (+)-gallocatechin, and (+)-catechin as well as flavonoids such as theaflavins and thearubigins. Several studies indicate that these polyphenols reduce intestinal absorption of cholesterol possibly by interfering with micelle formation and solubility. Additionally, they are also known to upregulate the LDL receptor (Bays and Stein, 2003).
Naringenin and Hesperetin
The citrus fruits contain a diverse range of flavonoids including numerous flavanone and flavone O–and C-glycosides and methoxylated flavones. Naringenin and hesperetin belong to the class of flavonoids called flavanones. They are found in abundance as the glycosides naringin and hesperidin in grapefruit and oranges, respectively. These glycosides are hydrolyzed by intestinal bacteria to their active forms, naringenin and hesperetin.
It has been hypothesized that the hypocholesterolemic effect of the citrus juices are due to their flavonoid components. The mechanism of action of both naringenin and hesperetin is similar to the general mechanism proposed for polyphenols viz. mediating a decrease in the availability of lipids for assembly of apoB-containing lipoproteins, by reducing the activities of ACAT2 and MTP as well as promoting the expression of the LDL receptor (Wilcox et al., 2001).
Dietary Fiber
Epidemiological studies have provided evidence that a high intake of dietary fiber may reduce the relative risk of coronary heart disease. This has prompted the US FDA to allow manufacturers to claim reduced CHD risk with high fiber products such as whole oat foods.
Dietary fiber generally implies non digestible carbohydrate components of plant parts. They are classified into two different types: soluble dietary fibers such as pectins, gums, β-glucans, starches and other storage polysaccharides and insoluble dietary fibers viz. cellulose and lignins. The soluble fibers are generally present in high levels in oats, barley, nuts, fruits, beans and vegetables while whole grains and cereals are a major food source of insoluble fiber. An excellent comprehensive review of the various types of dietary fiber, their sources and functions has been compiled by Tungland and Meyer (2002).
The soluble and insoluble fibers have very different influences on normal gut physiology and disease processes. Dietary insoluble fiber such as cellulose provides bulk, gentle laxation and ease of elimination. Due to its high affinity for water, cellulose increases stool weight, decreases transit time and intraluminal pressure (Tungland and Meyer, 2002).
Water soluble dietary fibers are known to effectively lower plasma cholesterol. The mechanism implicated is interference with bile acids absorption by biding and sequestering bile acids and reducing their reabsorption. This leads to increased diversion of cholesterol to bile acids in the liver, up regulation of lipoproteins receptors and decrease in plasma cholesterol.
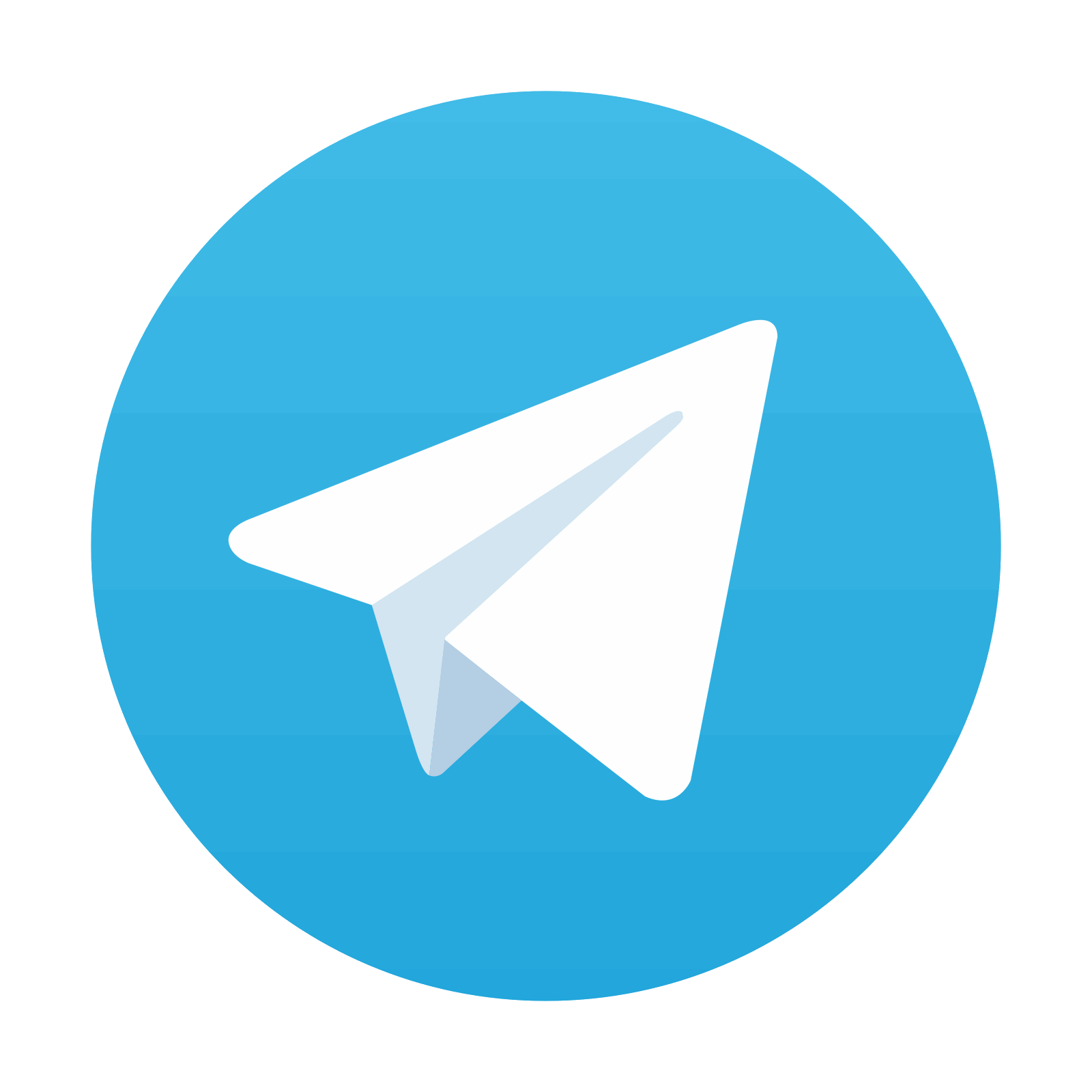
Stay updated, free articles. Join our Telegram channel

Full access? Get Clinical Tree
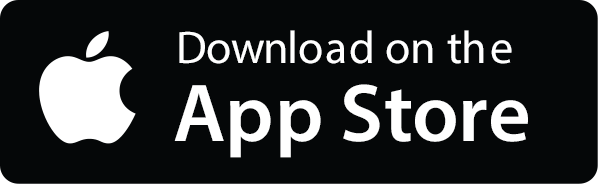
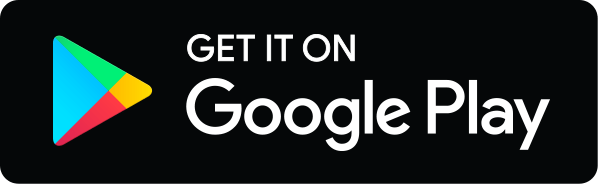