Figure 6.1:Morphological Features of Chicory (MiCichorium intybus L.)
Chicory Production
Around 1960, the chicory roots were first used as a coffee substitute in Holland followed by many other countries like Sweden, France, etc. During 1806-1813, the cultivation and use of roasted chicory roots as coffee substitute have spreaded over the whole European continent. In the 19th century, the main centers of cultivation were north east France, Belgium, England, Russia, Holland, Australia, etc. Subsequently, it was cultivated in large scale in Poland, Hungary, Germany, Switzerland and India. However, presently efforts have been made for large scale cultivation in United States of America, Australia, New Zealand, etc. Presently over 1/5th of world’s consumption take place in eastern Europe especially in Poland (6 per cent), followed by Russia, Hungary, Romania, Czechoslovakia, and Germany. According to percentage, it was reported that 12 per cent to be consumed in South Africa, 9 per cent in India and 2 per cent in United States of America (Clarke and Macrae, 1987).
In India, chicory crop is mainly cultivated in Gujarat and Uttar Pradesh. It is mainly cultivated in the Jamnagar, Kheda, Anand, and Mehsana districts of Gujarat. These districts have about 40 per cent share in the total production of chicory used in the manufacturing of coffee. In Uttar Pradesh, it is cultivated in the districts of Etah, and Aligarh, where an area of about 4000 ha. has come under its cultivation during last four–five years. The estimated production of chicory (Cichorium intybus L. Sativum D.C.) in India is about 20000 metric tones in roasted form. The average productivity of the chicory roots ranges between: 30-32.5/ha. with an average sale price of Rs. 1500/tone. The cost of cultivation and net return per hector works out to be respectively Rs. 12000/ha and 47000/ha, with net profit of Rs. 35000/ha (Chandra and Kumari, 2005).
Phytochemical Constituents
Fresh chicory roots contained a considerable quantity of water (70-80 per cent). The roots were distinctive in containing the soluble dietary fiber–Inulin, a polymer of fructose with β-(2-1) glycosidic linkages (Figure 6.2); belonging to the fructans family (Mac Grogor and Greenwood, 1980). From the culinary viewpoint, the inulin is particularly interesting. Upon roasting, inulin is converted to oxymethylfurfurol, a compound with a coffee like aroma. During storage the inulin present in roots is converted into inulide and finally into fructose due to the presence of an enzymes inulocoagulase, which has been reported in the expressed root juice. Chicory roots also contain a cytokine, ribosylzeatin, a nucleotide sugar, uridine-5’-diphosphoglucose.
Besides inulin, the chicory also contains bitter sesquiterpene lactones (Figure 6.3), belonging to three major classes: Guaianolides, Eudesmanolides and Germacranolides (Table 6.1). The Sesquiterpene lactones occur throughout the plant, though at highest level in the roots (0.42 per cent dry weight). Among these, lactucopicrine and dihydrolactucopicrine were found to be more bitter than quinine hydrochloride (van Beek et al., 1990). These bitter Sesquiterpene lactones were accumulated mainly as glycosides (Blaschek et al., 1998; Kisiel and Zielinska, 2001). Some of the guaianolides isolated from chicory play a role in chemical defense of chicory plant as antifeedent (Ress and Harborne, 1985) and phytoalexins (Monde et al., 1990; Grayer and Harborne, 1994). The (+)-Costunolide was considered as a parent compound, which on hydroxylation followed by cyclization converted into Germenacrene-A. The sesquiterpene lactones of chicory have been reported to have cytotoxic activity towards cultured cancer cells (Hladon’ et al., 1978; Seto et al., 1988). The chicory root extract showed antiinflammatory activity and hepatoprotective activity (Zafar and Ali, 1998; Ki et al., 1999). Recently, a molecular mechanism of antiinflammatory action of sesquiterpene lactones via inhibition of transcription factors NF-kB have been proposed (Rungeler et al., 1999; Han et al., 2001). The accumulation of sesquiterpene lactones in hairy root cultures of chicory have been also studied (Malarz et al., 2002).
Figure 6.2:Chemical Structure of Inulin
Table 6.1:Major Sesquiterpene Lactones Reported in Chicory
Guaianolides | Eudesmanolides | Germacranolides |
Lectucin, | Chicoriolide-A | Sonchuside-A, |
8-deoxylectucin, | Sonchuside-C | Chicorioside-B,C |
Lactucopicrin, | Germacrene-A | |
11(S),13-dihydrolectucin, | ||
11(S),13-dihydro-8-deoxylectucin, | ||
11(S),13-dihydro-8-deoxylectucin | ||
11(S),13-dihydrolactucopicrin |
Phenolic acids having antioxidant activity were reported in the chicory roots such as L-chicoric acid, caffeic acid, dihydrocaffeic acid, chlorogenic acid, neochlorogenic acid, isochlorogenic acid, rosamaric acid, and ferulic acid (Figure 6.4). A number of flavonoids were reported namely esculatine, esculin, scopoletin, kaempferol, chicorin, jacquinelin, umbelliferone. Some other constituents reported are amino acids (lysine, leucine, methionine, threonine, tryptophan, isoleucine, histidine, arginine, valine, phenylalanine), vitamins (β-carotene, cholin, inositol, niacin, thiamine, riboflavine), and fatty acids (stearic acid, plasmatic acid, lenoleic acid, alpha-lenoleic acid, oleic acid, myristic acid) as well as organic acids (valilic acid, p-hydroxybenzoic acid). Besides these, chicory also contains coumarins, occurring as glycosides. Hairy root culture of witloop chicory was found to produce coumarins, esculin and esculatin (Bais et al., 1999).
Figure 6.3:Chemical Structure of Sesqueterpene Lactones of Chicory
Figure 6.4:Chemical Structure of Organic Acids Present in Chicory
Pharmacological Activities
Dietary Fibers and Lipid Lowering Activity
According to dietary fiber technical committee of the American association on cereal chemists (AACC), “Dietary fiber is the edible part of plants or analogous carbohydrates that are resistant to digestion and absorption in the human small intestine with complete or partial fermentation on the large intestine. Dietary fiber includes polyssacharides, oligosaccarides, lignin and associated plant substances. Dietary fibers promote beneficial physiological effects including laxation and or blood cholesterol attenuation and/or blood glucose attenuation”. Some 36,000 plants from a variety of genera contain inulin as an energy source or as an osmoregulator assuring cold resistance. Many of commonly consumed foods were found to contain inulin (Table 6.2) (van Loo et al., 1995). The food industries were able to utilize few plant species for large scale production of inulin such as Agave (Agave azul tequllana), Jerusalem artichoke (Hellanthus tuberous) and Chicory (Cichorium intybus). The major polysaccharide of chicory root extract was inulin–a polymer of fructose with β(2-1) glycosidic linkage, belonging to fructans family. Since inulin is soluble in water and not hydrolyzed by the digestive enzymes (Knudsen and Hessov, 1995), it was classified as a soluble dietary fiber (Roberfroid, 1993). In vitro hydrolysis of chicory inulin, yielded fructose and glucose. In vitro Inulin hydrolysis to fructose have been reported with variety of substances such as exo-inulinase or endo-inulinase (Yun et al., 2000), catalysts (Motsumoto and Yurmazulkl, 1986), Zeolite (Jacob and Hinnekens, 1989; Abasaeed and Lee, 1995, 1996), activated carbon (Heinen et al., 2001) or commercial inulinase (Obo’n et al., 2000) like Fructozyme-L.
Table 6.2:Inulin Content (per cent of fresh weight) of Plants that are Commonly Used in Human Nutrition
Source | Edible Parts | Dry solids Content | Inulin Content |
Onion | Bulb | 6–12 | 2–6 |
Jerusalem artichoke | Tuber | 19–25 | 14–19 |
Chicory | Root | 20–25 | 15–20 |
Leek | Bulb | 15–20 | 3–10 |
Garlik | Bilb | 40–45 | 9–16 |
Artichoke | Leaves-heart | 14–16 | 3–10 |
Banana | Fruit | 24–26 | 0.3–0.7 |
Rye | Cereal | 88–90 | 0.5–1 |
Barley | Cereal | NA | 0.5–1.5 |
Dandelion | Leaves | 50–55 | 12–15 |
Burdock | Root | 21–25 | 3.5–4.0 |
Camas | Bulb | 31–50 | 12–22 |
Murnong | root | 25–28 | 8–13 |
Yacon | Root | 13–31 | 3–19 |
Salsify | Root | 20–22 | 4–11 |
NA: Data not available.
The hypolipidemic effects of soluble dietary fibers have been well established both in human (Glore et al., 1994) and animals (Overtone et al., 1994; de Deckere et al., 1995; Tokunaga et al., 1996; Roberfroid and Delzenne, 1998). The oligofructose was a non-digestible but fermentable oligomer of β-D-glucose obtained by enzymatic hydrolysis of the chicory inulin. Feeding a diet supplemented with 10 per cent oligofructose to rats have significantly lowered triglyceride (TG) and phospholipids in serum (Delzenne et al., 1993; Fiordaliso et al., 1995; Roberfroid and Delzenne, 1998). The hypolipidemic effects were likely due to decreased plasma VLDL levels (Fiordaliso et al., 1995). Hepatocytes isolated from the oligofructose fed rats have significantly lowered capacity to esterify (14C) palmitate into TG and a 40 per cent lower capacity to synthesize TG from (14C) acetate (Fiordaliso et al., 1995; Roberfroid and Delzenne, 1998). It has been suggested that there was a reduced de novo lipogenesis in liver which was a key even in reduction of VLDL triglyceride secretion in fructans fed rats. The activities of all the enzymes involved in lipogenesis like acety-CoA–carboxylase, fatty acid synthase (FAS), malic enzyme, ATP citrate lyase, glucose–6-phosphated dehydrogenase, were decreased by approximately 50 per cent in fructans fed rats. The enzyme FAS was believed to be regulated only through modification of protein and mRNA and it was the most sensitive to nutrients and hormones (Girard et al., 1997). In fructans fed rats, the liver m-RNA for FAS were reduced suggesting that dietary fibers were likely to modify lipogenic enzymes gene expression and thereby produced antilipidemic effect. It has been further supported by the fact that chronic feeding of oligofructose to rats prevented triacylglycerol accumulation induced by fructose in the liver (Kok et al., 1996).
When rats were fed with resistant starch, the serum TG level and FAS activity were reduced with concomitant lower post prandial insulinemia (Takase et al., 1994). However, the effects of inulin type of fructans on glycemic and insulinemia were not yet fully studied. In one of study, it was observed that oligofructose given to rats at a dose of 10 per cent for 30 days have reduced post prandial glycemia and insulinemia by 7 per cent and 20 per cent respectively, suggesting that inulin type fructans reduces glucose and insulin concentration and thereby decreased induction of lipogenic enzymes gene expression (Bichard, 1997), which was mediated through insulin (Girard et al., 1997). The streptozotocin-treated rats fed with a diet containing 20 per cent oligofructose for 2 months were found to show decrease in post prandial glycemia (Bichard, 1997).
Chicory inulin was fermented in large bowel into short chain carboxylic acid (SCCA) by bowel microbial flora. The production of SCCA in the large bowel of oligofructose fed rats was responsible for a more than 2 fold increase in the portal concentration of both acetate and propionate (Steinmetz and Porter, 1991; Girard et al., 1997). The propionate was reported to inhibit fatty acid synthesis in vitro (Nishina and Freeland, 1990; Wright et al., 1990; Lynn et al., 1994) where as acetate was found to be lipogenic substrate. It has been suggested that the production of SCCA especially propionate by fermentation of inulin fructans was partly responsible for antilipidemic effects of oligofructose and fructans.
It was believed that the dietary fructans modify the physiology of the large bowel wall cells and thereby decreased the synthesis and release of GIP and Glucagon-like peptide-1(GLP-1) amide which in turn decreased insulin level in blood resulting in poor expression of gene coding all lipogenic enzymes and decreased de novo synthesis of triglycerol. GIP and Glucagon-like peptide-1(GLP-1) amide were the hormonal mediators found to be responsible for post prandial insulin release from pancreatic β-cells (Morgan, 1996) and have direct anabolic insulin like action on lipid metabolism and stimulated de novo lipogenesis and increase lipoprotein lipase activity (Oben et al., 1991; Knapper et al., 1995; Zampelas et al., 1995). Thus, various mechanisms have been proposed for the hypolipidemic activity of inulin type of fructans and chicory root extract. However, none of the studies have suggested that any one mechanism was fully accounting for the hypolipidemic effect. Therefore, further investigation is required in this area, emphasizing the contribution of each mechanisms and behavior in presence of lipid lowering drugs.
Hypoglycemic Activity
Insufficient dietary fibers intake was associated with increased risk of diabetes (Trowell, 1972) or increased intake of dietary fibers reduced the risk of diabetes (Jenkins et al., 1995; Chandalia et al., 2000). Well fermented viscous fibers, either as a part of food or as a supplement, have reduced glycemic response (Jenkins et al., 1977; Anderson et al., 1987; Wolever, 1990; Jenkins et al., 1999) and improved insulin sensitivity (Fukagawa et al., 1990). Experimental evidence have demonstrated that the addition of viscous fibers in diet, slowed gastric emptying rates, reduced digestion and the absorption of glucose and thereby benefited immediate postprandial glucose metabolism (Kashimura et al., 1996) and long-term glucose control (Buyken et al., 1998; Vuksan et al., 1999; Chandalia et al., 2000) in individuals with diabetes mellitus. Long term ingestion of 50 g of dietary fibers per day for 24 weeks have improved glycemic control and reduced the number of hypoglycemic events in individual with type-I diabetes (Giacco et al., 2000; Kalkwarf et al., 2001). In pregnant women with type-1 diabetes, a higher fiber intake has been found to be associated with lower daily insulin requirement (Kalkwarf et al., 2001). Studies in individuals with type-2 diabetes, have suggested that high fiber intake has diminished insulin demand (Revillese et al., 1980; Simpson et al., 1981). Oligofructose given to rats at a dose of 10 per cent for 30 days have reduced the post prandial glycemia and insulinemia by 77 per cent and 20 per cent respectively (Bichard, 1997). The mechanisms through which, dietary fibers affect insulin requirement or insulin sensitivity were not clear. Various mechanisms have been proposed for hypoglycemic action of dietary fibers like;
- Change in the viscosity of small intestine after dietary fiber intake, leads to poor glucose absorption and thereby reduce immediate post prandial glucose levels.
- Production of SCCA on fermentation of dietary fibers by microbial flora, affects the postprandial glucose levels and insulin release. Propionate is glucogenic in liver and increases blood sugar level.
- Increased expression of the gut-derived proglucagon gene and secretion of proglucagon-derived peptides, including glucagons–like-peptide-1 (GLP-1) (Reimer and McBurney, 1996; Massimino et al., 1998), which was found to reduce gastric emptying rates, promoted glucose uptake and disposal in the peripheral tissues, enhanced insulin-dependent glucose disposal, inhibited glucagon secretion and reduced hepatic glucose output in animals and humans (D’Alwessio, 2000).
Chicory inulin, being a soluble fermentable dietary fiber, was likely to improve glycemic control and reduce insulin requirement. However, experimental data on humans have shown that oral inulin did not modify insulin sensitivity (Dominique et al., 2003) and in some cases even reduced insulin levels (Jackson et al., 1999). However, these studies were carried out in subjects with obesity or dyslipidemia and not with the diabetes. Recently, it has been observed that dietary fibers like inulin and oligofructose have decreased the production of GLP-1 in the gut, which was further supported by the decreased lipogenic enzymes gene expression in liver. Limited data were available and some were contradictory regarding the effect of chicory inulin on glycemic control.
Anticancer Activity
Various historical observational and epidemiological studies have supported that high fiber intake protect against cancer incidences (Trock et al., 1990; Steinmetz and Porter, 1991; Howe et al., 1992). Prebiotic fiber sources, such as inulin, act as selective substrate for bacteria that produce specific SCFA (Green et al., 1998) and can lower the intestinal pH. The butyrate has been shown to increase apoptosis in human colonic tumor cell lines (Hague et al., 1993). Prebiotic cultures, such as lactic culture, have been shown to possess antimutagenic and anticarcinogenic properties (Goldin and Gorbach, 1980; Bodana and Rao, 1990; Lidbeck et al., 1992). The bifidogenic and prebiotic role of chicory root extract was well established. The evidences have suggested that increased number of bifidobecteria in the colon and reduced intestinal pH have a direct impact on carcinogenesis in the large intestine (Goldin and Gorbach, 1980; Koo and Rao, 1991). Different mechanisms have been proposed for anticancer activities of dietary fibers like inulin:
- A reduction in the production of carcinogenic substances by decreasing the amount of pathogenic bacteria in the colon (Rumney and Rowland, 1995).
- Lowering of colonic pH to affect pH dependent enzymatic reactions, for example, secondary bile acid formation (Buddington et al., 1996; Rowland et al., 1998).
- Reducing the amount of carcinogenic substances available to colonic mucosa by adsorption of the substances to the cell wall of the microbiota, by speeding up the gastrointestinal transit time and by increasing colonic content, by promoting SCFA-butyric acid (Hague et al., 1993; Pool-Zobel et al., 1993; Reddy and Rivenson, 1993; Verghese et al., 1998).
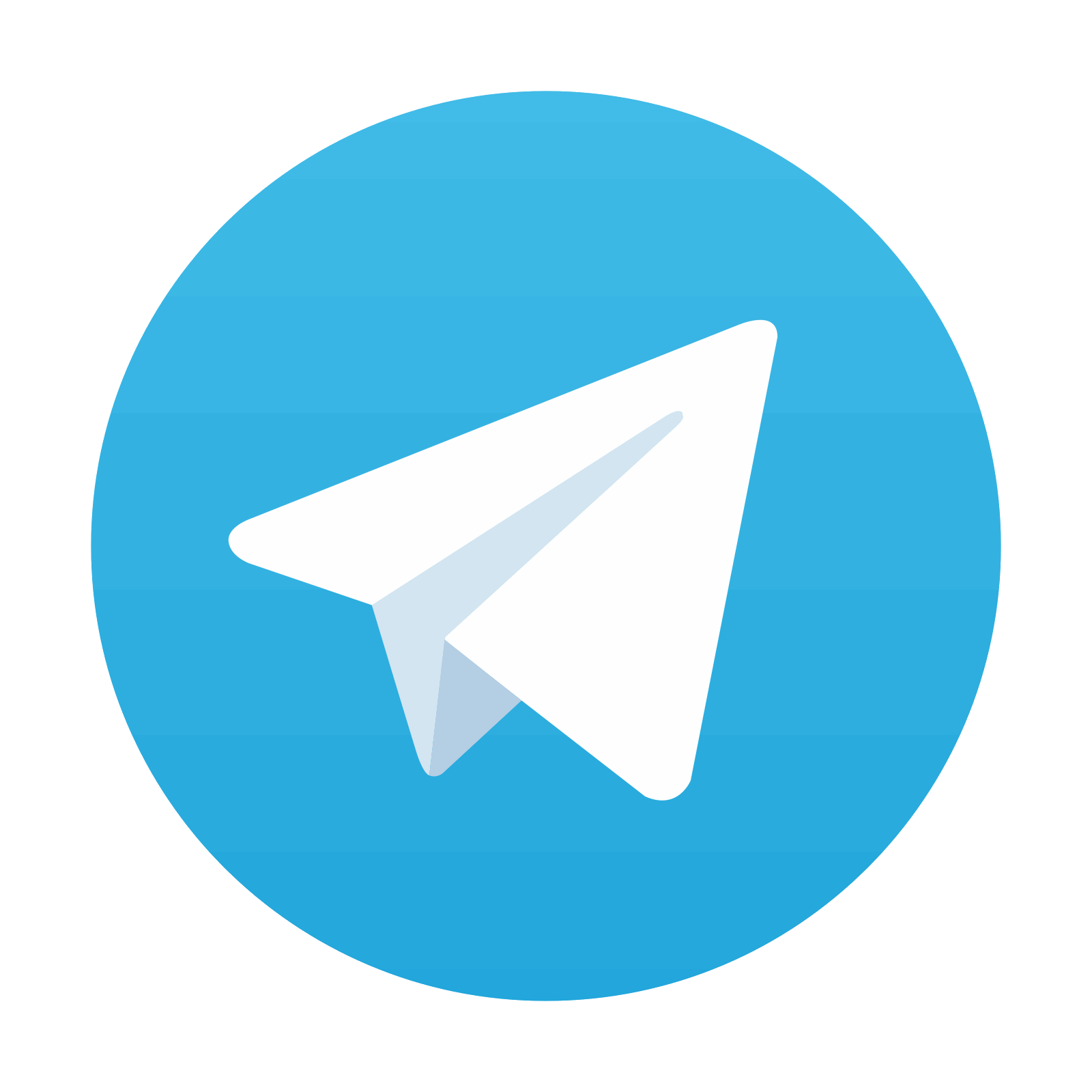
Stay updated, free articles. Join our Telegram channel

Full access? Get Clinical Tree
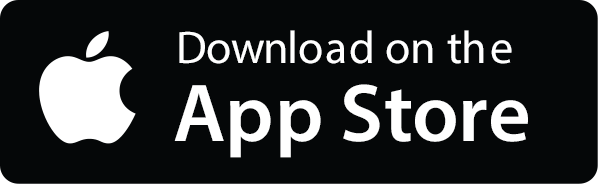
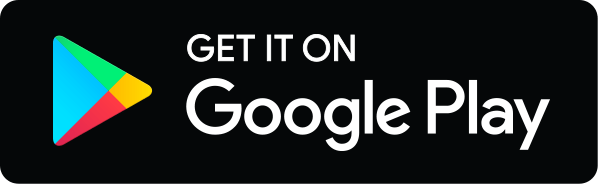