Nutrition and Metabolism
OVERVIEW OF NUTRITION AND METABOLISM
Nutrition and metabolism are words that are often used together—but what do they mean? Nutrition refers to the foods that we eat and the nutrients they contain. The Council on Food and Nutrition of the American Medical Association defines nutrition broadly as “the science of food; the nutrients and the substances therein; their action, interaction, and balance in relation to health and disease; and the process by which the organism (i.e., body) ingests, digests, absorbs, transports, utilizes, and excretes food substances.”
Healthy nutrition requires a balance of different nutrients in healthy amounts. Malnutrition is a deficiency or imbalance in the consumption of food, vitamins, and minerals. As a matter of convenient communication, many nutrition experts divide the essential (required) nutrients into two major categories:
We have already stated that healthy nutrition requires a balance of nutrients in the proper amounts. What do we mean by “proper balance” of nutrients? That is a puzzle that scientists continue to work on unraveling. The answer to the puzzle will certainly be complicated because we now know that a complex interaction of slight differences in individual genetic codes and individual lifestyles and environments affect how nutrients affect our bodies. Until this puzzle is completely solved, if it ever is, we fortunately have some advice that we can rely on to help us make healthy choices. For example, the United States government makes use of an individually customized food plate as a general nutrition guide (Figure 30-1). The Canadian government uses a food rainbow to advise eating a healthy, balanced diet (Figure 30-2).
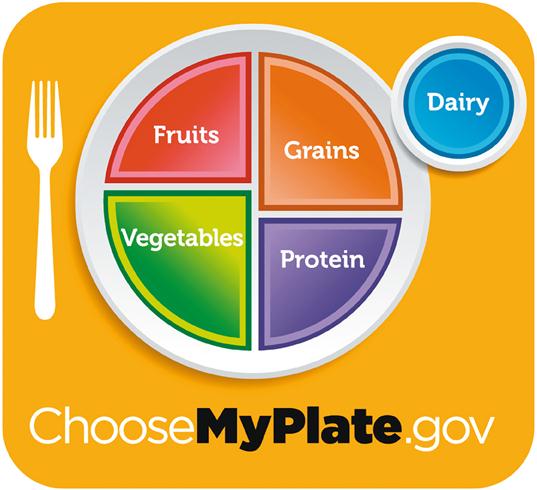
As we proceed through this chapter, we will discuss most of the major nutrients in more detail and their roles in maintaining the body.
Metabolism refers to the complex, interactive set of chemical processes that make life possible. A good phrase to remember in connection with the word metabolism is “use of foods” because basically this is what metabolism is—the use the body makes of foods after they have been digested, absorbed, and circulated to cells.
Your body cells use nutrients from food in several ways: as fuel (energy), as material for growth and maintenance, and for regulation of body functions. Before they can be used in these different ways, nutrients have to be assimilated. Assimilation occurs when nutrient molecules enter cells and undergo many chemical changes.
Metabolism is a complex process made up of many other processes. Two of the major metabolic processes are catabolism and anabolism. Each of these processes, in turn, consists of a series of enzyme-catalyzed chemical reactions known as metabolic pathways.
Catabolism breaks food molecules down into smaller molecular compounds and, in so doing, releases energy from them. Anabolism does the opposite. It builds nutrient molecules up into larger molecular compounds and, in so doing, uses energy. Catabolism is a decomposition process. Anabolism is a synthesis process. Both catabolism and anabolism take place inside cells. Both processes go on continually and concurrently.
Catabolism releases energy in two forms: heat and chemical energy. The amount of heat generated is relatively large—so large, in fact, that it would hard-boil cells if it were released in one large burst. Fortunately, this does not happen. Catabolism releases heat in frequent, small bursts. Heat is practically useless as an energy source for cells because they cannot use it to do their work. However, this heat is important in maintaining the homeostasis of body temperature. In contrast, chemical energy released by catabolism is more obviously useful. It cannot, however, be used directly for biological reactions. First, it must be transferred to the high-energy molecule of adenosine triphosphate (ATP) (Box 30-1).
ATP is one of the most important compounds in the world. Why? It supplies energy directly to the energy-using reactions of all cells in all kinds of living organisms, from one-celled plants to trillion-celled humans. ATP functions as the universal biological currency. It pays the energy bills for all cells and is as important in the world of cells as money is in the world of contemporary society.
Look now at Figure 30-3. The structural formula at the top of the diagram shows three phosphate groups attached to the rest of the ATP molecule, two of them by high-energy bonds. Adding water to ATP yields a phosphate group (P), adenosine diphosphate (ADP), and energy, which, as the diagram indicates, is used for anabolism and other cell work. The diagram also shows that P and ADP then use energy released by catabolism to recombine and form ATP. This cycle is called the ATP/ADP system.
Metabolism is not identical in all cells. It differs mainly with regard to rate and the kind of products synthesized by anabolism. More active cells have a higher metabolic rate than do less active cells. Anabolism in different kinds of cells produces different compounds. In liver cells, for example, anabolism synthesizes various blood protein compounds. Not so in beta cells of the pancreas. Anabolism there produces a different compound—insulin.
The bulk of this chapter discusses concepts related to the many and varied metabolic pathways of the human body. Our attempt here is to build on what you learned in previous chapters, but not to cover the subject of metabolism in its entirety—if that is even possible. Our goal, therefore, is to underscore the basic concepts of nutrition and metabolism. It is important to note that our discussion, as well as the diagrams that accompany the discussion, have been simplified to facilitate understanding of these basic concepts.
CARBOHYDRATES
Dietary Sources of Carbohydrates
Carbohydrates are found in most of the foods that we eat. Complex carbohydrates—polysaccharides such as starches in vegetables, grains, and other plant tissues—are broken down into simpler carbohydrates before they are absorbed.
Cellulose, a major component of most plant tissues, is an important exception to this principle. Because humans do not make enzymes that chemically digest this complex carbohydrate, it passes through our system without being broken down. Also called dietary fiber or “roughage,” cellulose and other indigestible polysaccharides keep chyme thick enough for the digestive system to push it easily. They also help mix chyme, much like the ball inside a can of spray paint. Most biologists believe that a high-fiber diet reduces the risk of many forms of cancer, including colorectal cancer.
Disaccharides such as those in refined sugar must also be chemically digested before they can be absorbed. Monosaccharides in fruits and some “diet foods” are already in an absorbable form, so they can move directly into the internal environment without initially being processed. The monosaccharide glucose is the carbohydrate that is most useful to the typical human cell. As Figure 30-4 shows, other important monosaccharides, fructose and galactose, are usually converted by liver cells into glucose for use by other cells of the body.
Carbohydrate Metabolism
The body metabolizes carbohydrates by both catabolic and anabolic processes. Because many human cells use carbohydrates—mainly glucose—as their first or preferred energy fuel, they catabolize most of the carbohydrate absorbed and anabolize a relatively small portion of it. When the amount of glucose entering cells is inadequate for their energy needs, they may make more use of an alternative pathway and catabolize fats or proteins.
We first discussed carbohydrate metabolism in Chapter 4 when we discussed basic concepts of cell metabolism (see pp. 102–104). The subject came up again in Chapter 12 when we discussed energy production in muscle tissue (see pp. 356–359). You may want to flip back to those passages and review the material before proceeding further with this chapter.
As you read through the following sections that briefly describe the process of carbohydrate metabolism, remember the ultimate result of catabolism: the transfer of energy from a nutrient molecule to ATP. It is the continued production of ATP, the energy currency of the cell, that makes nutrient catabolism so incredibly vital to the overall process of life itself.
GLUCOSE TRANSPORT AND PHOSPHORYLATION
Carbohydrate metabolism begins with the movement of glucose through cell membranes. Immediately on reaching the interior of a cell, glucose reacts with ATP to form glucose-6-phosphate. This step, named glucose phosphorylation, prepares glucose for further metabolic reactions. Phosphorylation (fos-for-i-LAY-shun) is the process of adding a phosphate group to a molecule. In most cells of the body, glucose phosphorylation is an irreversible reaction. However, in a few cells—namely, those of the intestinal mucosa, liver, and renal cortex—glucose phosphorylation is reversible. These cells contain phosphatase, an enzyme that splits phosphate off from glucose-6-phosphate. This reverse glucose phosphorylation reaction forms glucose, which then moves out of the cells into the blood. (Glucose-6-phosphate cannot pass through cell membranes.) Depending on their energy needs of the moment, cells either catabolize (break apart) or anabolize (bind together) glucose-6-phosphate.
GLYCOLYSIS
Glycolysis is the first step in the process of carbohydrate catabolism. It breaks apart one glucose molecule to form two pyruvic acid molecules. (A glucose molecule contains six carbon atoms, and a pyruvic acid molecule contains three carbon atoms. See Figure 30-6.) Glycolysis consists, as Figure 30-5 shows, of a series of chemical reactions. A specific enzyme catalyzes each of these reactions. Probably the most important facts for you to remember about glycolysis are the following:
CITRIC ACID CYCLE
As stated previously, for every glucose molecule that enters the catabolic pathway described here, two pyruvic acid molecules are produced. Before each pyruvic acid molecule can proceed into the citric acid cycle, it must be converted into an acetyl group (acetate), releasing a carbon dioxide molecule and being carried into the citric acid cycle by coenzyme A (CoA). Essentially, the citric acid cycle converts the two acetyl molecules to four carbon dioxide and six water molecules. But many chemical reactions intervene. Figure 30-6 shows that one glucose molecule is changed by glycolysis to two pyruvic acid molecules, which, by means of the citric acid cycle, yield six carbon dioxide molecules. Figure 30-7 shows the details of the citric acid cycle.
Glycolysis takes place in the cytoplasm of cells, whereas the citric acid cycle occurs in their mitochondria. Some of the enzymes needed for the many steps of the citric acid cycle are dissolved in the matrix of the mitochondrion, and some are attached to the inner membrane of the mitochondrion.
Before it can enter the citric acid cycle, each pyruvic acid molecule combines with coenzyme A (see Figure 30-7) after splitting off CO2 and a pair of high-energy electrons (with their accompanying protons, H+) from pyruvic acid, thus forming acetyl CoA. Coenzyme A then detaches from acetyl CoA, leaving a two-carbon acetyl group, which enters the citric acid cycle by combining with oxaloacetic acid to form citric acid. This is what gives the citric acid cycle its name. The cycle is also called the tricarboxylic acid (TCA) cycle because citric acid is also called tricarboxylic acid. For many years this cycle was called the Krebs cycle after Sir Hans Krebs, whose brilliant work in discovering this metabolic pathway earned him the 1953 Nobel Prize.
You probably do not need to memorize the names of the intermediate products formed during the citric acid cycle, but notice that all of them are acids. You should note that a little bit of ATP is directly generated by the citric acid cycle (in step 5). In step 5, energy is transferred first to GTP (guanosine triphosphate), a nucleotide similar to ATP, and then finally to ATP.
Observe, too, that for each pyruvic acid molecule entering this pathway, three CO2 molecules are formed and that certain reactions yield high-energy electrons. Most of the energy leaving the citric acid cycle is in these high-energy electrons. The next section describes how these high-energy electrons are used to generate ATP.
ELECTRON TRANSPORT SYSTEM AND OXIDATIVE PHOSPHORYLATION
High-energy electrons removed during the citric acid cycle enter a chain of carrier molecules, which is embedded in the inner membrane of mitochondria and is known as the electron transport system (ETS). Figure 30-8 shows that high-energy electrons (along with their accompanying protons, H+) are carried to the electron transport system by NAD and FAD. The electrons quickly move down the chain, from one membrane protein complex to the next, eventually to their final acceptor, oxygen.
As the electrons are transported, some of their energy is used to pump their accompanying protons (H+) to the intramembrane space between the inner and outer membranes of the mitochondrion. This creates a concentration gradient of protons, and the intermembrane space thus becomes a virtual reservoir of protons. As with water behind a dam, the reservoir of protons temporarily stores energy. As in a dam possessing water wheels that convert energy, the inner membrane has “proton wheels” built into it—in the form of ATP synthase. Figure 30-9 shows how protons move down their concentration gradient and into the ATP synthase structure, turning a molecular wheel that transfers energy by synthesizing ATP from ADP and phosphate.
At this time, the low-energy electrons (e−) and their protons (H+) join oxygen, forming water. As you can see, although oxygen is not needed until the very last step of aerobic respiration, its role is vital. Without oxygen to oxidize the hydrogen into water, the energy generation pathway would stop.
Oxidative phosphorylation refers to this oxygen-requiring joining of a phosphate group to ADP to form ATP—a reaction whose importance can scarcely be overemphasized.
The arrangement of catabolic “machinery” within the cell, as currently viewed, is shown in Figure 30-10. Glycolytic enzymes in the cytoplasm catalyze the production of pyruvic acid, which diffuses into mitochondria. The enzymes of the citric acid cycle have been localized mostly to the matter (matrix) inside the inner mitochondrial membrane. The high-energy electrons and their accompanying protons are then carried to the cristae of the inner membrane, where the electron transport carriers and mechanism for phosphorylation are found. Because so many of the cell’s energy-releasing enzymes are located within the mitochondria, these tiny structures are aptly described as the “power plants” of the cell.
The breakdown of ATP molecules, of course, provides virtually all the energy that does cellular work. Therefore, the process that produces some 90% of the ATP formed during carbohydrate catabolism, namely, oxidative phosphorylation, is the crucial part of catabolism (Figure 30-11). This vital process depends on cells receiving an adequate oxygen supply. Why? Briefly, because only when oxygen is present in cells to serve as the final acceptor of electrons and hydrogen ions can electrons then continue moving down the electron transport chain. If oxygen becomes unavailable, the movement of electrons and hydrogen ions stops. Cessation of ATP formation by oxidative phosphorylation necessarily follows. All too soon, cells have an inadequate energy supply—a lethal condition if it persists for more than a few minutes.
We can summarize the long series of chemical reactions in glucose catabolism with one short equation:

ANAEROBIC PATHWAY
What if there is an inadequate amount of oxygen to operate the electron transport system and oxidative phosphorylation? You may recall from Chapter 12 that this often occurs in skeletal muscle cells, especially during strenuous exercise. You may remember that another pathway for the catabolism of glucose exists. It is sometimes called the anaerobic pathway because it transfers energy to ATP using only glycolysis—a process that does not require oxygen.
As Figure 30-12 shows, there are two main pathways that glucose or its derivatives can take. One is the pathway that ends with oxidative phosphorylation of ATP. This pathway, described in the previous sections, is called the aerobic pathway, or aerobic respiration, because it requires the presence of oxygen (Box 30-2). If enough oxygen is not available to operate this pathway, the cell will rely solely on glycolysis to produce ATP. Even though this process does not extract the maximum amount of energy from a glucose molecule, it is the only ATP-producing process that can operate under anaerobic conditions. Because the pyruvic acid molecule produced by glycolysis cannot enter the citric acid cycle, it is converted to lactic acid rather than acetyl CoA. Lactic acid cannot enter the citric acid cycle.
The production of lactic acid does something very important—it converts the NADH (reduced nicotinamide adenine dinucleotide) produced by glycolysis to NAD. As glycolysis proceeds, NAD is converted to NADH. If you look at Figure 30-5 carefully, you will see that there is no reaction in the glycolytic pathway to turn NADH back into NAD again. This is no problem if the aerobic pathway is in operation—NADH is converted back to NAD in the electron transport system. After a short period of anaerobic activity, however, glycolysis will turn all of the cell’s NAD into NADH and thus glycolysis will have to shut down because of a lack of free NAD. The production of lactic acid solves this biochemical dilemma because this reaction also converts NADH back to NAD.
Once oxygen becomes available again in cells that ordinarily rely on aerobic respiration, some of the lactic acid is converted back to pyruvic acid by the cell (see Figure 30-12). Notice that such reconversion requires energy from ATP. Thus reconversion in muscle cells, for example, can occur only when phosphorylation has resumed and produced enough ATP to allow reconversion to occur. Once it is converted to pyruvic acid, the molecule can follow the aerobic pathway and become completely catabolized.
Much of the lactic acid produced during anaerobic glycolysis diffuses into the blood and is removed by the liver. Inside liver cells, ATP produced by oxidative phosphorylation is used to convert the lactic acid back into glucose. The glucose may then be stored as glycogen in the liver or be returned through the bloodstream for use by other cells. Figure 30-13
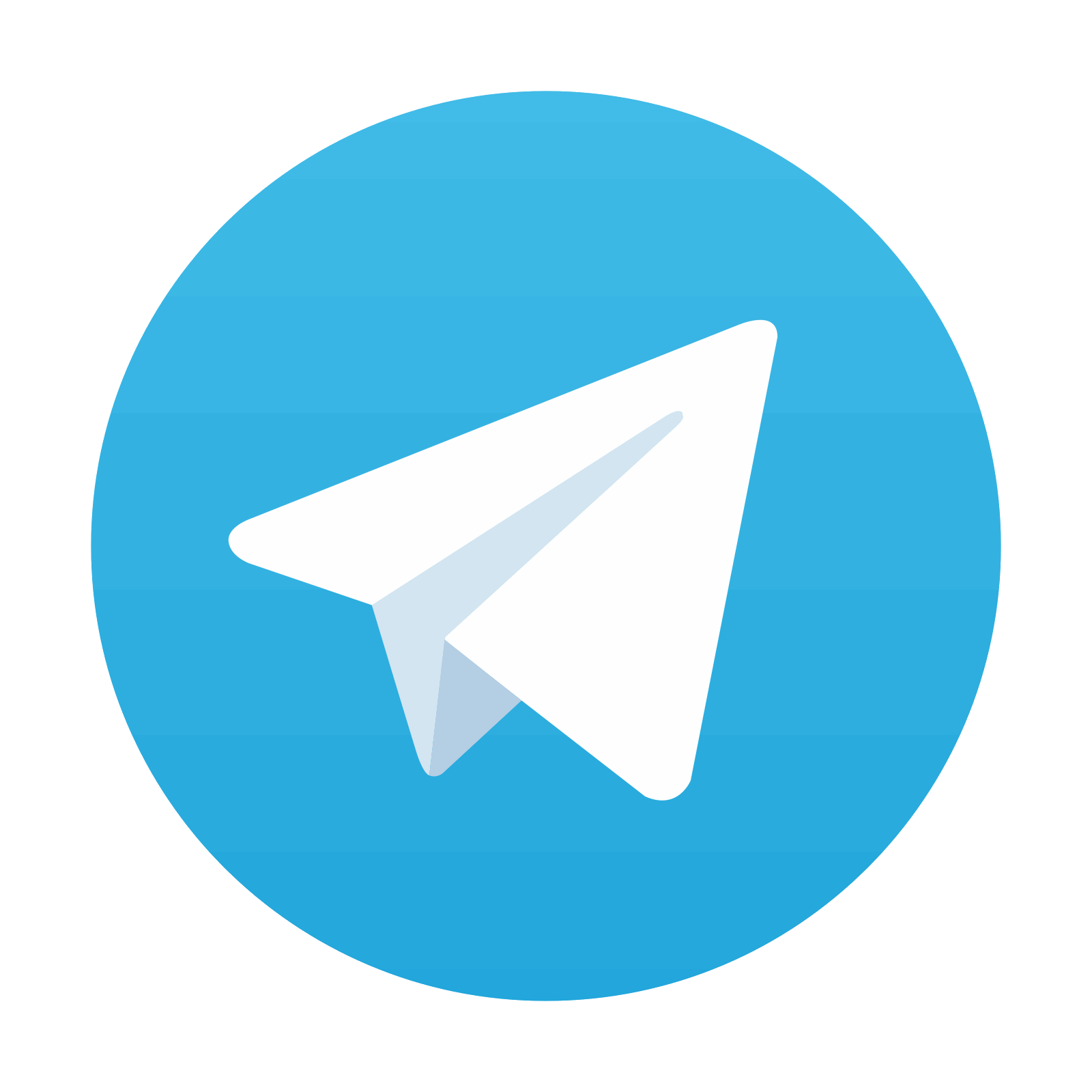
Stay updated, free articles. Join our Telegram channel

Full access? Get Clinical Tree
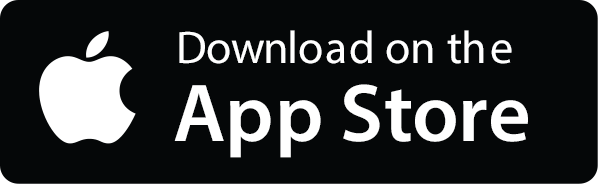
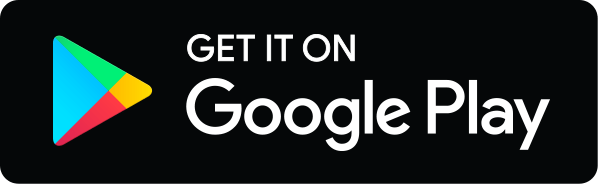