UREA
The NPN compound present in highest concentration in the blood is urea (Fig. 12.1). Urea is the major excretory product of protein metabolism.4 It is formed in the liver from amino groups (−NH2) and free ammonia generated during protein catabolism.5 This enzymatically catalyzed process is termed the urea cycle. Since historic assays for urea were based on the measurement of nitrogen, the term blood urea nitrogen (BUN) has been used to refer to urea determination. Urea nitrogen (urea N) is a more appropriate term.6
FIGURE 12.1 Structure of urea.
Biochemistry
Protein metabolism produces amino acids that can be oxidized to produce energy or stored as fat and glycogen. These processes release nitrogen, which is converted to urea and excreted as a waste product. Following synthesis in the liver, urea is carried in the blood to the kidney, where it is readily filtered from the plasma by the glomerulus. Most of the urea in the glomerular filtrate is excreted in the urine, although some urea is reabsorbed by passive diffusion during passage of the filtrate through the renal tubules. The amount reabsorbed depends on the urine flow rate and extent of hydration. Small quantities of urea (<10% of the total) are excreted through the gastrointestinal (GI) tract and skin. The concentration of urea in the plasma is determined by the protein content of the diet, the rate of protein catabolism, and renal function and perfusion.7
Clinical Application
Measurement of urea is used to evaluate renal function, to assess hydration status, to determine nitrogen balance, to aid in the diagnosis of renal disease, and to verify adequacy of dialysis.4
Measurements of urea were originally performed on a protein-free filtrate of whole blood and based on measuring the amount of nitrogen. Current analytic methods have retained this custom, and urea is often reported in terms of nitrogen concentration rather than urea concentration. Urea N concentration can be converted to urea concentration by multiplying by 2.14, as follows:
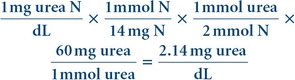
In the International System of Units (SI), urea is reported in units of millimoles per liter. Urea N concentration in milligrams per deciliter may be converted to urea concentration in millimoles per liter by multiplying by 0.36.8
Analytical Methods
Several analytic approaches have been used to assay urea. Enzymatic methods are used most frequently in clinical laboratories.9 The enzyme urease (urea amidohydrolase, EC 3.5.1.5) catalyzes hydrolysis of urea in the sample, and the ammonium ion () produced in the reaction is quantified.10 The most common method couples the urease reaction with glutamate dehydrogenase (GLDH, EC 1.4.1.3), and the rate of disappearance of nicotinamide adenine dinucleotide (reduced, NADH) at 340 nm is measured (Fig. 12.2).11
FIGURE 12.2 Enzymatic assay for urea.
Ammonium from the urease reaction can also be measured by the color change associated with a pH indicator. This approach has been incorporated into instruments using liquid reagents, a multilayer film format, and reagent strips.12, 13, 14 A method that uses an electrode to measure the rate of increase in conductivity as ammonium ions are produced from urea is in use in approximately 15% of laboratories in the United States.9,15 Because the rate of change in conductivity is measured, ammonia contamination is not a problem as it is in other methods.
A reference method using isotope dilution mass spectrometry (IDMS) has been developed.16 Analytic methods are summarized in Table 12.2.
TABLE 12.2 Summary of Analytic Methods—Urea
Specimen Requirements
Urea concentration may be measured in plasma, serum, or urine. If plasma is collected, ammonium ions and high concentrations of sodium citrate and sodium fluoride must be avoided; citrate and fluoride inhibit urease.8 Although the protein content of the diet influences urea concentration, the effect of a single protein-containing meal is minimal and a fasting sample is not required usually. A nonhemolyzed sample is recommended. Urea is susceptible to bacterial decomposition, so specimens (particularly urine) that cannot be analyzed within a few hours should be refrigerated. Timed urine specimens should be refrigerated during the collection period. Methods for plasma or serum may require modification for use with urine specimens because of high urea concentration and the presence of endogenous ammonia.8
Reference Intervals
Pathophysiology
An elevated concentration of urea in the blood is called azotemia. Very high plasma urea concentration accompanied by renal failure is called uremia or the uremic syndrome. This condition is eventually fatal if not treated by dialysis or transplantation. Conditions causing increased plasma urea are classified according to the cause into three main categories: prerenal, renal, and postrenal.7
Prerenal azotemia is a result of reduced renal blood flow. Less blood is delivered to the kidney; consequently, less urea is filtered. Causative factors include congestive heart failure, shock, hemorrhage, dehydration, and other factors resulting in a significant decrease in blood volume. The amount of protein metabolism also induces prerenal changes in blood urea concentration. A high-protein diet or increased protein catabolism, such as occurs in stress, fever, major illness, corticosteroid therapy, and GI hemorrhage, may increase the urea concentration.
Decreased renal function causes an increase in plasma urea concentration as a result of compromised urea excretion. Renal causes of elevated urea include acute and chronic renal failure, glomerular nephritis, tubular necrosis, and other intrinsic renal disease (see Chapter 27). Postrenal azotemia can be due to obstruction of urine flow anywhere in the urinary tract by renal calculi, tumors of the bladder or prostate, or severe infection.
The major causes of decreased plasma urea concentration include low protein intake and severe liver disease. Plasma urea concentration is decreased during late pregnancy and in infancy as a result of increased protein synthesis. The conditions affecting plasma urea concentration are summarized in Table 12.3.
TABLE 12.3 Causes of Abnormal Plasma Urea Concentration
Differentiation of the cause of abnormal urea concentration is aided by calculation of the urea nitrogen/creatinine (urea N/creatinine) ratio, which is normally 10:1 to 20:1. Prerenal conditions tend to elevate plasma urea, whereas plasma creatinine remains normal, causing a high urea N/creatinine ratio. A high urea N/creatinine ratio with an elevated creatinine is usually seen in postrenal conditions. A low urea N/creatinine ratio is observed in conditions associated with decreased urea production, such as low protein intake, acute tubular necrosis, and severe liver disease.7
CASE STUDY 12.1
A 65-year-old man was first admitted for treatment of chronic obstructive lung disease, renal insufficiency, and significant cardiomegaly. Pertinent laboratory data on admission (5/31) are shown in Case Study Table 12.1.1.
CASE STUDY TABLE 12.1.1 Laboratory Results—First Admission
Because of severe respiratory distress, the patient was transferred to the intensive care unit, placed on a respirator, and given diuretics and intravenous (IV) fluids to promote diuresis. This treatment brought about a significant improvement in both cardiac output and renal function, as shown by laboratory results several days later (6/3). After 2 additional days on a respirator with IV therapy, the patient’s renal function had returned to normal and, at discharge, his laboratory results were within normal limits (6/7).
The patient was readmitted 6 months later because of the increasing inability of his family to arouse him. On admission, he was shown to have a tremendously enlarged heart with severe pulmonary disease, heart failure, and probable renal failure. Laboratory studies on admission were as shown in Case Study Table 12.1.2. Numerous attempts were made to improve the patient’s cardiac and pulmonary function, all to no avail, and the patient died 4 days later.
CASE STUDY TABLE 12.1.2 Laboratory Results—Second Admission
Question
1. What is the most likely cause of the patient’s elevated urea nitrogen? Which data support your conclusion?
URIC ACID
Uric acid is the product of catabolism of the purine nucleic acids.17 Although it is filtered by the glomerulus and secreted by the distal tubules into the urine, most uric acid is reabsorbed in the proximal tubules and reused.18 Uric acid is relatively insoluble in plasma and, at high concentrations, can be deposited in the joints and tissue, causing painful inflammation.
Biochemistry
Purines, such as adenine and guanine from the breakdown of ingested nucleic acids or from tissue destruction, are converted into uric acid, primarily in the liver. Uric acid is transported in the plasma from the liver to the kidney, where it is filtered by the glomerulus. Reabsorption of 98% to 100% of the uric acid from the glomerular filtrate occurs in the proximal tubules. Small amounts of uric acid are secreted by the distal tubules into the urine. Renal excretion accounts for about 70% of uric acid elimination; the remainder passes into the GI tract and is degraded by bacterial enzymes.18
Nearly all of the uric acid in plasma is present as monosodium urate. At the pH of plasma (pH ~ 7), urate is relatively insoluble; at concentrations greater than 6.8 mg/dL, the plasma is saturated. As a result, urate crystals may form and precipitate in the tissues. In acidic urine (pH < 5.75), uric acid is the predominant species and uric acid crystals may form.18
Clinical Application
Uric acid is measured to confirm diagnosis and monitor treatment of gout, to prevent uric acid nephropathy during chemotherapeutic treatment, to assess inherited disorders of purine metabolism, to detect kidney dysfunction, and to assist in the diagnosis of renal calculi.19
Analytical Methods
In higher primates, such as humans and apes, uric acid is the final breakdown product of purine metabolism.4 Most other mammals have the ability to catabolize purines to allantoin, a more water-soluble end product. This reaction is shown in Figure 12.3. Uric acid is readily oxidized to allantoin and, therefore, can function as a reducing agent in chemical reactions. This property was exploited in early analytic procedures for the determination of uric acid. The most common method of this type is the Caraway method, which is based on the oxidation of uric acid in a protein-free filtrate, with subsequent reduction of phosphotungstic acid in alkaline solution to tungsten blue.20 The method lacks specificity.
FIGURE 12.3 Conversion of uric acid to allantoin.
Methods using uricase (urate oxidase, EC 1.7.3.3), the enzyme that catalyzes the oxidation of uric acid to allantoin, are more specific and are used almost exclusively in clinical laboratories. The simplest of these methods measures the differential absorption of uric acid and allantoin at 293 nm.21 The difference in absorbance before and after incubation with uricase is proportional to the uric acid concentration. Proteins can cause high background absorbance, reducing sensitivity; hemoglobin and xanthine can cause negative interference.22
Coupled enzyme methods measure the hydrogen peroxide produced as uric acid is converted to allantoin.23,24 Peroxidase or catalase (EC 1.11.1.6) is used to catalyze a chemical indicator reaction. The color produced is proportional to the quantity of uric acid in the specimen. Enzymatic methods of this kind have been adapted for use on traditional wet chemistry analyzers and for dry chemistry slide analyzers. Bilirubin and ascorbic acid, which destroy peroxide, if present in sufficient quantity, can interfere. Commercial reagent preparations often include potassium ferricyanide and ascorbate oxidase to minimize these interferences.
HPLC (high-performance liquid chromatography) methods, typically using UV detection, have been developed.25 IDMS has been proposed as a candidate reference method.26,27 Analytic methods are summarized in Table 12.4.
TABLE 12.4 Summary of Analytic Methods—Uric Acid
Specimen Requirements
Uric acid may be measured in heparinized plasma, serum, or urine. Serum should be removed from cells as quickly as possible to prevent dilution by intracellular contents. Diet may affect uric acid concentration overall, but a recent meal has no significant effect and a fasting specimen is unnecessary. Gross lipemia should be avoided. High bilirubin concentration may falsely decrease results obtained by peroxidase methods. Significant hemolysis, with concomitant glutathione release, may result in low values. Drugs such as salicylates and thiazides have been shown to increase values for uric acid.28
Uric acid is stable in plasma or serum after red blood cells have been removed. Serum samples may be stored refrigerated for 3 to 5 days. Ethylenediaminetetraacetic acid (EDTA) or fluoride additives should not be used for specimens that will be tested by a uricase method. Urine collections must be alkaline (pH 8).8
Reference Intervals
Results expressed in conventional units of milligrams per deciliter can be converted to SI units using the molecular mass of uric acid (168 g/mol).
Pathophysiology
Abnormally increased plasma uric acid concentration is found in gout, increased catabolism of nucleic acids, and renal disease.18 Gout is a disease found primarily in men and is usually first diagnosed between 30 and 50 years of age. Affected individuals have pain and inflammation of the joints caused by precipitation of sodium urates. In 25% to 30% of these patients, hyperuricemia is a result of overproduction of uric acid, although hyperuricemia may be exacerbated by a purine-rich diet, drugs, and alcohol. Plasma uric acid concentration in affected individuals is usually greater than 6.0 mg/dL. Patients with gout are susceptible to the formation of renal calculi, although not all persons with abnormally high serum urate concentrations develop this complication. In women, urate concentration rises after menopause. Postmenopausal women may develop hyperuricemia and gout. In severe cases, deposits of crystalline uric acid and urates called tophi form in tissue, causing deformities.18
Another common cause of elevated plasma uric acid concentration is increased metabolism of cell nuclei, as occurs in patients on chemotherapy for such proliferative diseases as leukemia, lymphoma, multiple myeloma, and polycythemia. Monitoring uric acid concentration in these patients is important to avoid nephrotoxicity. Allopurinol, which inhibits xanthine oxidase (EC 1.1.3.22), an enzyme in the uric acid synthesis pathway, is used for treatment.
Patients with hemolytic or megaloblastic anemia may exhibit elevated uric acid concentration. Increased urate concentrations may be found following ingestion of a diet rich in purines (e.g., liver, kidney, sweetbreads, and shellfish) or as a result of increased tissue catabolism due to inadequate dietary intake (starvation).18
Inherited disorders of purine metabolism are associated with significant increases in physiological uric acid concentrations.18 Lesch-Nyhan syndrome is an X-linked genetic disorder (seen only in males) caused by the complete deficiency of hypoxanthine–guanine phosphoribosyltransferase (EC 2.4.2.8), an important enzyme in the biosynthesis of purines. Lack of this enzyme prevents the reutilization of purine bases in the nucleotide salvage pathway and results in increased de novo synthesis of purine nucleotides and high plasma and urine concentrations of uric acid. Neurologic symptoms, mental retardation, and self-mutilation characterize this extremely rare disease. Mutations in the first enzyme in the purine synthesis pathway, phosphoribosylpyrophosphate synthetase (EC 2.7.6.1), also cause elevated uric acid concentration. Increased uric acid is found secondary to glycogen storage disease (deficiency of glucose-6-phosphatase, EC 3.1.3.9) and fructose intolerance (deficiency of fructose-1-phosphate aldolase, EC 2.1.2.13). Metabolites such as lactate and triglycerides are produced in excess and compete with urate for renal excretion in these diseases.
Hyperuricemia as a result of decreased uric acid excretion is a common feature of toxemia of pregnancy (preeclampsia) and lactic acidosis presumably as a result of competition for binding sites in the renal tubules. Chronic renal disease causes elevated uric acid concentration because filtration and secretion are impaired.
Uric acid nephrolithiasis, the formation of kidney stones (renal calculi), may occur due to a variety of predisposing factors and conditions.29 In acidic urine, the relatively insoluble uric acid precipitates to form calculi, which can cause intense flank pain. The stones may be dissolved by alkalinization of the urine or treated by increased fluid intake and administration of xanthine oxidase inhibitors to reduce uric acid production.
Hypouricemia is less common than hyperuricemia and is usually secondary to severe liver disease or defective tubular reabsorption, as in Fanconi syndrome (a disorder of reabsorption in the proximal convoluted tubules of the kidney).30 Decreased plasma uric acid can be caused by chemotherapy with 6-mercaptopurine or azathioprine, inhibitors of de novo purine synthesis, and as a result of overtreatment with allopurinol. Some studies have shown an association between low uric acid concentrations and neurodegenerative conditions such as Alzheimer’s and Parkinson’s diseases.31
Conditions known to affect plasma urate concentrations are shown in Table 12.5.
TABLE 12.5 Causes of Abnormal Plasma Uric Acid Concentration
CREATININE/CREATINE
Creatinine is formed from creatine and creatine phosphate in muscle and is excreted into the plasma at a constant rate related to muscle mass. Plasma creatinine is inversely related to glomerular filtration rate (GFR) and, although an imperfect measure, it is commonly used to assess renal filtration function.6
Biochemistry
Creatine is synthesized primarily in the liver from arginine, glycine, and methionine.5 It is then transported to other tissues, such as muscle, where it is converted to creatine phosphate, which serves as a high-energy source. Creatine phosphate loses phosphoric acid and creatine loses water to form the cyclic compound, creatinine, which diffuses into the plasma and is excreted in the urine. The structures and relationship of these compounds are shown in Figure 12.4.
FIGURE 12.4 Interconversion of creatine, creatine phosphate, and creatinine.
Creatinine is released into the circulation at a relatively constant rate that has been shown to be proportional to an individual’s muscle mass. It is removed from the circulation by glomerular filtration and excreted in the urine. Small amounts of creatinine are secreted by the proximal tubule and reabsorbed by the renal tubules.6 Daily creatinine excretion is reasonably stable.
Clinical Application
Measurement of creatinine concentration is used to determine the sufficiency of kidney function, to determine the severity of kidney damage, and to monitor the progression of kidney disease.32
Plasma creatinine concentration is a function of relative muscle mass, the rate of creatine turnover, and renal function. The amount of creatinine in the bloodstream is reasonably stable, although the protein content of the diet does influence the plasma concentration. Because of the constancy of endogenous production, urinary creatinine excretion has been used as a measure of the completeness of 24-hour urine collections in a given individual, although the uncertainty associated with this practice may exceed that introduced by use of the urine volume and collection time for standardization.33 Urinary constituents may be expressed as a ratio to creatinine quantity rather than as mass excreted per day.
Creatinine clearance (CrCl), a measure of the amount of creatinine eliminated from the blood by the kidneys, and GFR are used to gauge renal function.34 The GFR is the volume of plasma filtered (V) by the glomerulus per unit of time (t):

Assuming a substance, S, can be measured and is freely filtered at the glomerulus and neither secreted nor reabsorbed by the tubules, the volume of plasma filtered would be equal to the mass of S filtered (MS) divided by its plasma concentration (PS):

The mass of S filtered is equal to the product of its urine concentration (US) and the urine volume (VU):

If the urine and plasma concentrations of S, the volume of urine collected, and the time over which the sample was collected are known, the GFR can be calculated:
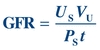
The clearance of a substance is the volume of plasma from which that substance is removed per unit time. The formula for CrCl is given as follows, where UCr is urine creatinine concentration and PCr is plasma creatinine concentration:
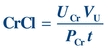
CrCl is usually reported in units of mL/min and can be corrected for body surface area (see Chapter 27). CrCl overestimates GFR because a small amount of creatinine is reabsorbed by the renal tubules and up to 10% of urine creatinine is secreted by the tubules. However, CrCl provides a reasonable approximation of GFR.34
The observed relationship between plasma creatinine and GFR and relatively constant plasma creatinine concentrations should make the analyte a good endogenous filtration marker. However, measurement of plasma creatinine does not provide sufficient sensitivity for the detection of mild renal dysfunction. Measured creatinine concentration used in combination with other variables in one of several empirically determined equations provides a better assessment of renal disease, in part because the equations estimate GFR, not CrCl.
Clinical laboratories have been strongly encouraged to report an estimated GFR when serum creatinine is ordered as a means to increase identification of kidney disease and improve patient care.35 Initially, the abbreviated Modification of Diet in Renal Disease (MDRD) equation was advocated.36 The equation includes four variables—serum creatinine concentration, age, gender (sex), and ethnicity—and makes the assumption that all filtered creatinine is excreted. The MDRD equation is most useful when serum creatinine results are produced in an assay that has been calibrated to be traceable to an IDMS method.37
When serum creatinine is measured using an IDMS-traceable method, the MDRD equation for estimated glomerular filtration rate (eGFR) is
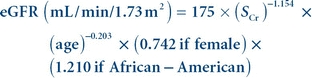
where Scr is serum (plasma) creatinine concentration in mg/dL and age is in years.38 Results are normalized to a standard body surface area (1.73 m2). The equation is valid for individuals older than 18 years and younger than 70 years.37 The formula was developed with data from nonhospitalized patients known to have chronic kidney disease and is reasonably accurate for this population, although eGFR values greater than 60 mL/min/1.73 m2 calculated using this equation are negatively biased and should not be reported.
Effectiveness of the equation in other populations is being investigated and alternate equations have been proposed.39 The Chronic Kidney Disease Epidemiology (CKD-EPI) Collaboration published an equation developed using results from healthy adults and adults with chronic kidney disease.40 The CKD-EPI equation is used to report higher values (>60 mL/min/1.73 m2) for adults 18 years and older. A modified Schwartz equation has been developed to calculate eGFR in the pediatric population.41 The equation is

Height is measured in cm and Scr is serum (plasma) creatinine concentration in mg/dL. The equation can be used to calculate eGFR for children younger than 18 years old. The original Schwartz equation overestimates the GFR by 20% to 40% for creatinine concentrations measured by a traceable method.35
Analytical Methods
Creatinine
The methods most frequently used to measure creatinine are based on the Jaffe reaction first described in 1886.42 In this reaction, creatinine reacts with picric acid in alkaline solution to form a red-orange chromogen. The reaction was adopted for the measurement of blood creatinine by Folin and Wu in 1919.43 The reaction is nonspecific and subject to positive interference by a large number of compounds, including acetoacetate, acetone, ascorbate, glucose, and pyruvate. More accurate results are obtained when creatinine in a protein-free filtrate is adsorbed onto Fuller’s earth (aluminum magnesium silicate) or Lloyd’s reagent (sodium aluminum silicate), then eluted and reacted with alkaline picrate.44 This method is time consuming and not readily automated; it is not routinely used.
Two approaches have been used to increase the specificity of assay methods for creatinine: a kinetic Jaffe method and reaction with various enzymes. In the kinetic Jaffe method, serum is mixed with alkaline picrate and the rate of change in absorbance is measured.45 Although this method eliminates some of the nonspecific reactants, it is subject to interference by α-keto acids and cephalosporins.46 Bilirubin and hemoglobin may cause a negative bias, probably a result of their destruction in the strong base used. The kinetic Jaffe method is used routinely despite these problems because it is inexpensive, rapid, and easy to perform.
In an effort to enhance the specificity of the Jaffe reaction, several coupled enzymatic methods have been developed.47,48 The method using creatininase (creatinine amidohydrolase, EC 3.5.2.10), creatinase (creatine amidinohydrolase, EC 3.5.3.3), sarcosine oxidase (EC 1.5.3.1), and peroxidase (EC 1.11.1.7) was adapted for use on a dry slide analyzer.49
IDMS is used as a reference method.37 Assays used on automated analyzers are designated as “traceable” (calibrated) to an IDMS method. Analytic methods for creatinine are summarized in Table 12.6.
TABLE 12.6 Summary of Analytic Methods—Creatinine
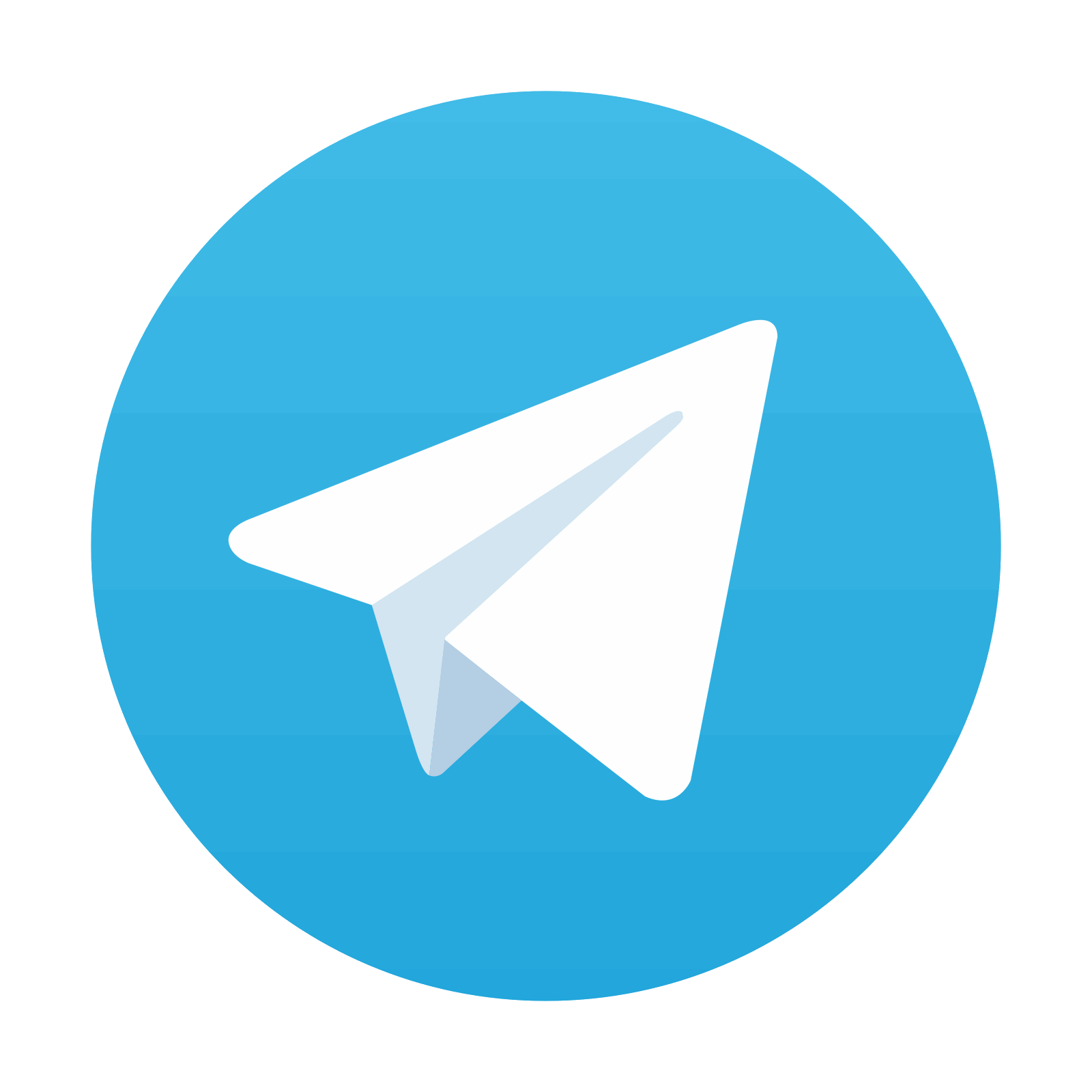
Stay updated, free articles. Join our Telegram channel

Full access? Get Clinical Tree
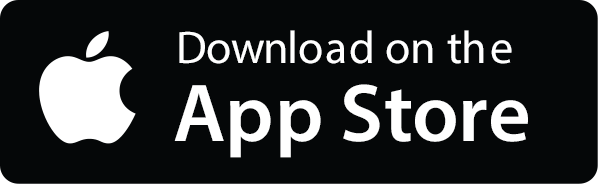
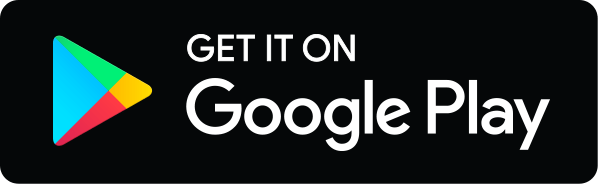