Nonneoplastic Diseases of Bones
Fiona M. Maclean
S. Fiona Bonar
Peter G. Bullough
This chapter discusses the three main classes of bone disease, other than neoplasms, of concern to the surgical pathologist: metabolic disease, traumatic lesions, and infections. To optimally report these diseases, it is useful to be aware of the relevant clinical history, including which bones are involved, the location of the lesion within the bone, and the radiologic features. The chapter begins with a brief discussion of bone structure and tissue preparation.
TISSUE STRUCTURE
Most of the organ systems that present to the surgical pathologist consist of cellular tissues. In contrast, the connective tissues are made up principally of an extracellular matrix, which serves a mechanical function (1). In general, most of the symptoms of connective tissue disease are a result of structural failure of the extracellular matrix (e.g., fracture).
Connective tissues (e.g., ligaments, tendons, bone, cartilage) are subjected principally to three types of applied stress: (a) compression, (b) tension, and (c) shear. The constituents of the extracellular matrix are ideally suited to resisting one or another of these types of stress. Whereas collagen resists tension, hydroxyapatite in the bone and the large globular proteoglycan aggregates in the cartilage are particularly well suited to resisting compression. Thus, the combination of matrix constituents in each type of tissue reflects the types of stresses that are applied to it.
The extracellular matrix is synthesized and, to a greater or lesser extent, broken down by its intrinsic cells (i.e., fibroblasts, osteoblasts, and chondroblasts) (2). The matrix has a very definite microarchitecture designed to resist the forces that act on the anatomic structure (3,4). For example, collagen fibers run more or less parallel to the length of the ligaments and tendons. Similarly, within the cancellous bone, the trabeculae are arranged along the principal lines of stress. The cortex is thicker in the middle of the shaft of a long bone to resist bending forces, whereas the cancellous bone is concentrated at the ends of the long bones to resist the mainly compressive forces that are common at that site. It is important for the surgical pathologist to understand these physiologic and anatomic principles because without them, it is not easy to interpret the histologic findings in diseased connective tissues.
METHODS OF EXAMINATION
Specimens received by the surgical pathologist from orthopedic operations often consist of fragments of bone and soft tissue without obvious anatomic landmarks. However, when a large piece of bone is submitted, anatomic landmarks should be carefully sought (5). The bony specimens should be cut into thin slices of 3 or 4 mm with a band saw. The cut surfaces of the bone should be gently washed and brushed with a nail brush to remove the bone dust, which otherwise may cause histologic artifacts. The color of the bone should be noted; necrotic or dead bone is likely to have an opaque and yellow appearance, whereas living bone appears translucent and pink. An obvious increase or decrease in bone density should be sought, as should localized lesions. Obviously, some experience is required to perform the examination, and a dissecting microscope, such as is used in the operating room, may be useful. Another useful adjunct to the gross examination is the preparation of radiographs of the sliced bone specimens. These can be very helpful in defining both the texture and margins of a lesion, which may not be immediately apparent on gross examination; we have found this technique to be particularly useful in identifying the nidus of an osteoid osteoma (Fig. 7.1).
The preparation of routine sections of bone after embedding requires the prior removal of calcium from the bone. As occasionally an unexpected underlying neoplastic process may be found, we routinely ensure that at least some of the tissue is decalcified in gentle decalcifying agents (ethylenediaminetetraacetic acid [EDTA] or other more recently available
commercial products), as this allows molecular techniques to be undertaken when necessary. Calcium can be removed successfully and relatively quickly if some simple guidelines are followed. First, before decalcification, the tissue must be well fixed. Second, slices, not whole specimens, should be decalcified. Third, it must be realized that as the calcium is removed by acid, the acid is neutralized and depleted. Therefore, it is necessary that an adequate amount of acid be used, that the acid solution be changed frequently, and that a shaker be used to ensure that the acid thoroughly infiltrates the specimen. Following decalcification, all of the acid must be washed out of the specimen; otherwise, poor staining may result. Washing for at least 12 hours in running water usually ensures good differentiation of the hematoxylin and eosin stain.
commercial products), as this allows molecular techniques to be undertaken when necessary. Calcium can be removed successfully and relatively quickly if some simple guidelines are followed. First, before decalcification, the tissue must be well fixed. Second, slices, not whole specimens, should be decalcified. Third, it must be realized that as the calcium is removed by acid, the acid is neutralized and depleted. Therefore, it is necessary that an adequate amount of acid be used, that the acid solution be changed frequently, and that a shaker be used to ensure that the acid thoroughly infiltrates the specimen. Following decalcification, all of the acid must be washed out of the specimen; otherwise, poor staining may result. Washing for at least 12 hours in running water usually ensures good differentiation of the hematoxylin and eosin stain.
TABLE 7.1 Parameters Indicative of Bone Formation | ||||||||||||||||||||||||||||||||||||||||||||||||||||||||||||||||||||||||||||||||||||||||||||||||||||||||
---|---|---|---|---|---|---|---|---|---|---|---|---|---|---|---|---|---|---|---|---|---|---|---|---|---|---|---|---|---|---|---|---|---|---|---|---|---|---|---|---|---|---|---|---|---|---|---|---|---|---|---|---|---|---|---|---|---|---|---|---|---|---|---|---|---|---|---|---|---|---|---|---|---|---|---|---|---|---|---|---|---|---|---|---|---|---|---|---|---|---|---|---|---|---|---|---|---|---|---|---|---|---|---|---|
|
Histomorphometry, which is the quantification of morphologic features at the tissue and cellular levels, can further define the metabolic diseases of bone (6). The parameters indicative of bone formation include the percentages of active osteoblastic surface, osteoid surface, and mineralizing surface. Those indicative of bone mineralization include osteoid volume and mineral apposition rate. Those indicative of bone resorption include total eroded surface and active osteoclastic surface. Table 7.1 summarizes these parameters in the various metabolic bone diseases. Pathologists often say, “I don’t need a number to make the diagnosis of osteopenia.” This is true, but morphometry helps clarify the dynamics of the disease and subtle alterations in the balance between osteoblastic formative activity and osteoclastic resorption. Morphometry enhances the assessment of metabolic bone disease by supplementing the information gained from qualitative visual examination with quantitative information. Furthermore, the discipline required to make objective measurements can only improve visual diagnostic acumen.
METABOLIC DISEASES
The quality and quantity of the extracellular matrix of the bone reflect cellular activity (7,8 and 9). Osteoblasts synthesize the collagenous matrix of the bone and regulate its mineralization. Osteoclasts, which may be multinucleated, are fewer in number than osteoblasts and are responsible for removing bone. For the amount of bone in the skeleton to remain stable, the rate of production must equal the rate of resorption; this equilibrium is often referred to as cell coupling or linkage. The rate of matrix production is reflected in the cytologic appearance of the osteoblasts, which range from flat (inactive) to plump (active) (Fig. 7.2). As bone formation proceeds, some of the osteoblasts are buried within the matrix and become osteocytes. The osteocytes maintain contact with each other and with the surface osteoblasts through cellular processes that run through a network of canaliculi within the bone matrix to form a functional syncytium, which is thought to play a major role in both mineral and structural homeostasis (Fig. 7.3). Cortical bone in adults is composed of closely applied, densely compact cylindrical units called osteons (Fig. 7.4). Primary osteons are formed in juveniles by the ingrowth of periosteal blood vessels that follow a “cutting cone” of osteoclasts, which tunnel through the existing cortex deposited by the periosteum. The tunnel,
or haversian canal, formed by the osteoclasts and containing the vessels is subsequently partially filled in by osteoblasts, which deposit concentric layers of bone matrix. Secondary and subsequent osteons are formed during the process of remodeling by the outgrowth from existing haversian systems of new vessels; these are also preceded by a cluster of osteoclasts. Cortical bone has a predominantly structural role, whereas cancellous bone prevails in day to day metabolic activity. Osteoblastic activity is probably positively influenced by increased physical activity, parathyroid hormone, and certain growth factors. Activity of the osteoblasts is suppressed by inactivity and steroid hormones (10,11 and 12). Throughout life, maintenance of normal healthy bone is dependent on adequate load.
or haversian canal, formed by the osteoclasts and containing the vessels is subsequently partially filled in by osteoblasts, which deposit concentric layers of bone matrix. Secondary and subsequent osteons are formed during the process of remodeling by the outgrowth from existing haversian systems of new vessels; these are also preceded by a cluster of osteoclasts. Cortical bone has a predominantly structural role, whereas cancellous bone prevails in day to day metabolic activity. Osteoblastic activity is probably positively influenced by increased physical activity, parathyroid hormone, and certain growth factors. Activity of the osteoblasts is suppressed by inactivity and steroid hormones (10,11 and 12). Throughout life, maintenance of normal healthy bone is dependent on adequate load.
When the osteoblasts synthesize collagen, it is deposited in parallel layers to produce the lamellar pattern that characterizes normal adult bone (Fig. 7.5). Time is required to achieve this high degree of organization, and when bone is formed and deposited rapidly, as in the fetus, in fracture callus, or in certain neoplastic and hypermetabolic states, it is less obviously organized, and the collagen has a basket-like weave. This type of bone is referred to as woven or immature bone (Fig. 7.6). This is always abnormal after the age of 3 years and should alert the pathologist to look for underlying pathology. (The microscopic examination of collagen is greatly aided by the use of polarized light, which demonstrates not only the collagen fibers but also their organization.)
The distribution of calcium in bone cannot be studied by examining decalcified sections. However, it is possible to study the mineralization of the matrix if undecalcified sections are prepared. For the study of those diseases in which disturbed mineralization is suspected, this examination is essential (13). When the organic matrix is first deposited by the osteoblast, it is not mineralized; therefore, a layer of unmineralized matrix, termed osteoid, is always present at the formative surface of bone (Fig. 7.7). Normally, this layer is very thin because the time between matrix deposition and subsequent mineralization is short; however, when the rates of deposition and mineralization are unbalanced, the amount and extent of the osteoid increase, and hyperosteoidosis may develop. Hyperosteoidosis occurs basically for three reasons. First, in any condition in which bone formation is increased, the osteoid surfaces are both more extensive and thicker (e.g., fracture callus, Paget disease, hyperparathyroidism). Second, the mineralization of bone depends on the presence of many substances, including
calcium, phosphorus, vitamin D, and alkaline phosphatase. If the amount of any of these substances is inadequate, mineralization is delayed, perhaps markedly. Third, the presence in bone of certain inhibitory or toxic substances, such as aluminum, iron, and fluoride, may block mineralization, even though the amounts of calcium, alkaline phosphatase, and vitamin D in the tissues are adequate.
calcium, phosphorus, vitamin D, and alkaline phosphatase. If the amount of any of these substances is inadequate, mineralization is delayed, perhaps markedly. Third, the presence in bone of certain inhibitory or toxic substances, such as aluminum, iron, and fluoride, may block mineralization, even though the amounts of calcium, alkaline phosphatase, and vitamin D in the tissues are adequate.
![]() FIGURE 7.7 A section of undecalcified bone demonstrating a layer of unmineralized bone (osteoid) at the surface. The osteoid in this section is stained red, and the mineralized bone is black. |
Tetracycline binds to actively mineralizing surfaces and exhibits fluorescence in ultraviolet light on microscopic examination; these features have made it a valuable aid in the study of bone and bone diseases (14). Pulsed doses of tetracycline can be administered to a patient before biopsy to determine the extent of mineralization and the amount of bone formed over a given time (Fig. 7.8). Additionally, the morphology of the tetracycline labels reflects the cause of the disease process. When mineralization is blocked, especially in an aluminum-associated disease, the label is not taken up. On the other hand, in patients with vitamin D deficiency, the labels are often extensive and smudged, whereas they are sharp in persons with normal bone formation (Fig. 7.9). (It should be noted that tetracycline may be passively absorbed onto a recently resorbed surface and thus confuse interpretation.)
![]() FIGURE 7.9 Photomicrograph demonstrating a smudged tetracycline label at the mineralization front in a patient with osteomalacia. |
The resorption of bone by osteoclasts, rather than reflecting physiologic calcium homeostasis, is primarily associated with maintenance of the structural integrity of the skeleton. Morphologically, osteoclasts, which are large cells containing approximately two to four nuclei, adhere to the bone surface and are seen in depressions referred to as Howship lacunae or resorption bays (Fig. 7.10). In cancellous bone, resorption is generally limited to the bone surface, and the portions of the surface that have been resorbed can be recognized by an irregular, scalloped appearance, which contrasts with the smooth surface of formative or resting bone. Eventually, the resorptive surfaces are again covered by osteoblasts, and new bone forms on them. The junction between the original resorbed surface and the new bone is marked on sections stained with hematoxylin and eosin by a sharp basophilic line referred to as a reversal front or cement line (Fig. 7.11) (15).
![]() FIGURE 7.10 The irregular eroded surface associated with osteoclastic activity. Two osteoclasts are seen at the surface lying in shallow depressions, or Howship lacunae. |
In diseases characterized by increased remodeling, such as Paget disease and osteoporosis, the osteoclastic activity, although often markedly increased, is generally confined to the surface. However, in hyperparathyroidism, whether primary or secondary, the osteoclasts characteristically tunnel into the mineralized bone matrix, creating a “wormhole” appearance referred to as dissecting resorption/tunnel resorption (Fig. 7.12). However, regardless of the cause or patterns of resorption, the architecture of the bone is distorted in all pathologic resorptive states.
ABNORMAL FORMATION OF THE ORGANIC MATRIX
The synthesis of collagen by the cells may be abnormal because of either genetic or acquired conditions. Examples of the former are osteogenesis imperfecta and Ehlers-Danlos syndrome, whereas examples of the latter are scurvy and lathyrism (a disease related to ingestion of certain legumes). Today, the condition most likely to be seen by the surgical pathologist is osteogenesis imperfecta.
![]() FIGURE 7.12 Dissection resorption/tunnel resorption with osteoclasts tunneling into the mineralized bone matrix. Bone trabecula with vigorous resorption by centrally located osteoclasts. |
Osteogenesis Imperfecta
Osteogenesis imperfecta, one of the most common of the congenital connective tissue matrix diseases, comprises several distinct syndromes, some inherited as an autosomal dominant trait and others as a recessive trait; still others occur as spontaneous mutations. All have collagen mutations, mostly of COL1A1 and COL1A2, resulting in diminished quantity and quality of collagen type I (16). Persons with these various syndromes have in common a short stature and a propensity for fracture. Many of the patients also have poorly formed dentin, hearing loss, and frequently blue sclerae. Most of their clinical problems are caused by fractures, and those more severely affected, during the course of their childhood, sustain hundreds of major and minor fractures. The fractures occur more often in the lower limbs and, in a significant number of cases, involve the growth plates around the knee joints, giving rise eventually to growth plate fragmentation. Subsequent independent growth of the cartilage fragments causes swelling of the epiphyseal end of the bone. The deformed bone end filled with nodules of cartilage has been likened, on x-ray films, to a bag of popcorn (Fig. 7.13). These disruptions of the growth plate result in disproportionate shortening of the lower limbs in affected persons (17).
Bone samples from patients with the severe congenital form of osteogenesis imperfecta characteristically lack an organized trabecular pattern. The osteocytes are crowded within the bone, reflecting diminished collagen synthesis by the crowded osteoblasts at the surface. Frequently, large areas of woven bone are seen. In less severely affected persons, the bone is generally lamellar in pattern, although even in this bone, the osteocytes are crowded and the lamellae may be thinner than those in agematched controls (Fig. 7.14). The lack of collagen synthesis by osteoblasts results in severely osteoporotic bone, which in turn leads to the numerous fractures characteristic of this condition.
ABNORMAL MINERALIZATION
The mineralization of bone depends on several factors, including vitamin D, calcium, phosphate, and alkaline phosphatase; it may be disturbed by the presence of certain metals, such as lead, strontium, iron, and aluminum.
Because routine microscopic examination of bone requires decalcification of the tissue before embedding and sectioning, disease resulting from abnormal mineralization is likely to be overlooked by pathologists unless the clinician has alerted them to the possibility. Plastic embedding can be used to prepare undecalcified sections. If small pieces of only cancellous bone are used, even paraffin-embedded undecalcified tissue may provide adequate sections for diagnosis.
Osteomalacia and Rickets
In general, the terms osteomalacia (in adults) and rickets (in children) are used to describe those diseases that result from a deficiency in vitamin D, an abnormality in the metabolism of vitamin D, or a deficiency of calcium in the diet. The most common symptom of osteomalacia is bone pain, which is usually
generalized and often vague. In addition, the low calcium level may cause muscle weakness, which is often profound (18). Radiographic examination reveals generalized osteopenia with the classic finding of multiple bilateral and symmetric partial linear fractures of the bone, commonly referred to as insufficiency fractures (Fig. 7.15).
generalized and often vague. In addition, the low calcium level may cause muscle weakness, which is often profound (18). Radiographic examination reveals generalized osteopenia with the classic finding of multiple bilateral and symmetric partial linear fractures of the bone, commonly referred to as insufficiency fractures (Fig. 7.15).
On microscopic examination, the most striking abnormalities are a massive increase in the amount of unmineralized bone (up to 40% or 50% of the total bone volume) and disorganization of the trabecular architecture. The mineralization front, which is the junction between the osteoid and mineralized bone, is very irregular, granular, and fuzzy. In addition to an increase in osteoid volume, bone volume is often increased overall as a consequence of increased osteoblastic activity (Fig. 7.16).
![]() FIGURE 7.14 Polarized light photomicrograph demonstrating the fine lamellar pattern seen in patients with osteogenesis imperfecta. This photograph should be contrasted with Figure 7.5. |
Rickets is the childhood manifestation of osteomalacia. In childhood, the anatomic changes are found most characteristically around the metaphyses of the most rapidly growing bones—that is, around the knee and wrist joints. On x-ray films, the epiphyseal growth plates are irregular and broadened and have a characteristic cup shape. Microscopically, the growth plate is thickened and poorly defined, especially on its metaphyseal side, where tongues of uncalcified cartilage can be seen extending into the metaphysis. As in adults, the bone shows extensive, wide osteoid seams.
In addition to the classic forms of osteomalacia and rickets secondary to calcium and vitamin D deficiencies, severe osteomalacia may occasionally be secondary to hypophosphatemia. Usually, the hypophosphatemia is the result of increased urinary phosphate loss, which may be the consequence of a primary renal tubular defect, diuretic therapy, or hyperparathyroidism. Very rarely, hypophosphatemia is associated with FGF23-producing, phosphaturic mesenchymal tumor, which has been documented in many locations and may be very tiny, resulting in oncogenic osteomalacia. In this instance, resection of the tumor generally results in complete resolution of the osteomalacia. Therefore, it is important to look for an occult tumor in cases of osteomalacia without a clear cause (19,20 and 21).
Hypophosphatasia
Hypophosphatasia (not to be confused with hypophosphatemia) is a rare genetic disease characterized by a disturbance in the synthesis of the enzyme alkaline phosphatase (22). It takes two forms. The first is inherited as an autosomal recessive trait that manifests as severe disease in infants. In general, when hypophosphatasia is diagnosed in infants younger than 6 months of age, it follows a rapidly progressive fatal course. The second form is an autosomal dominant condition that may not become evident until adulthood. In these cases, the disease is less severe and often asymptomatic.
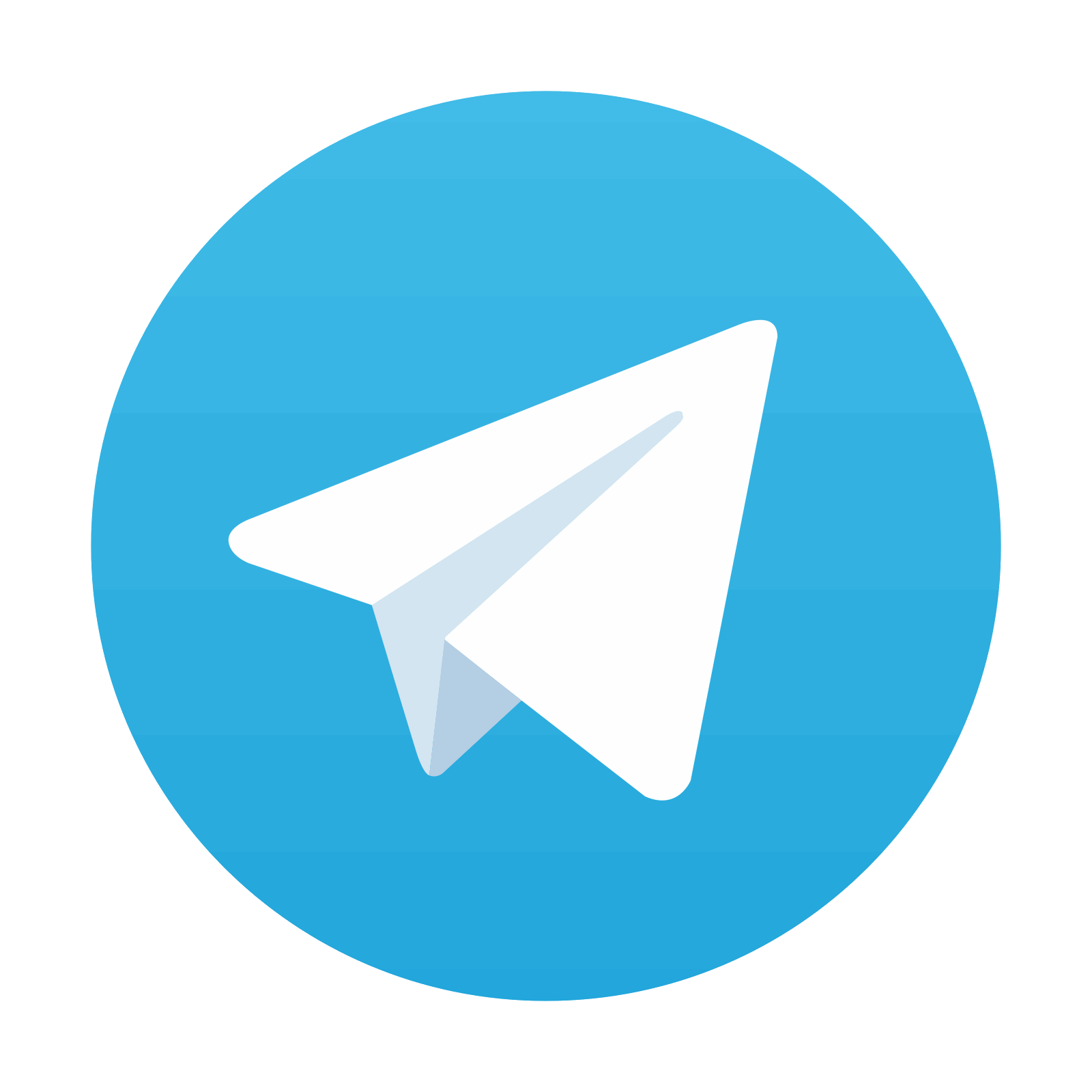
Stay updated, free articles. Join our Telegram channel

Full access? Get Clinical Tree
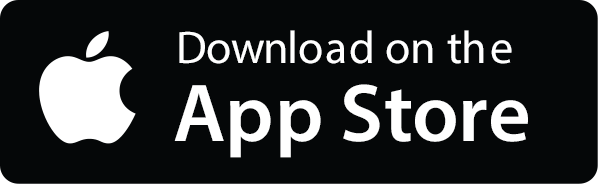
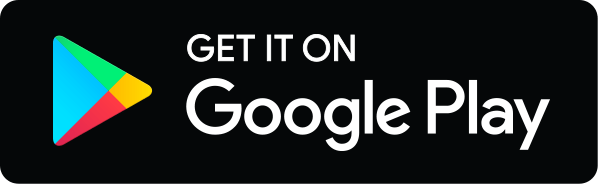
