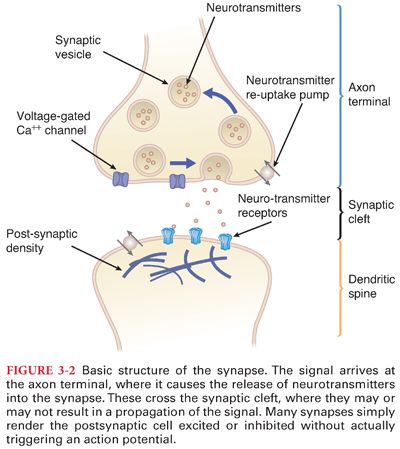
B. Classification of Afferent Nerve Fibers
1. Nerve fibers are called afferent if they transmit impulses from peripheral receptors to the central nervous system (CNS) and efferent if they transmit impulses from the CNS to the periphery. Afferent nerve fibers are classified as A, B, and C on the basis of fiber diameter and velocity of conduction of nerve impulses (Table 3-1).
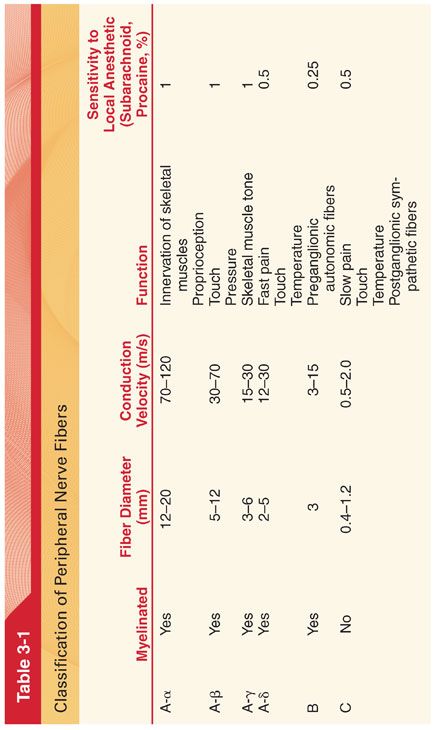
2. Myelin that surrounds type A and B nerve fibers acts as an insulator that prevents flow of ions across nerve membranes. Type C fibers are unmyelinated. The myelin sheath is interrupted approximately every 1 to 2 mm by the nodes of Ranvier (see Fig. 3-1).
3. This successive excitation of nodes of Ranvier by an action potential that jumps between successive nodes is termed saltatory conduction. Saltatory conduction allows for a 10-fold increase in the velocity of nerve transmission.
C. Evaluation of Peripheral Nerve Function
1. Nerve conduction studies are useful in the localization and assessment of peripheral nerve dysfunction.
2. Electromyographic testing is helpful in determining the etiology of neurologic dysfunction that may occur after surgery.
D. Action Potential
1. Electrical potentials exist across nearly all cell membranes, reflecting principally the difference in transmembrane concentrations of sodium and potassium ions.
2. The resulting voltage difference across the cell membrane is called the resting membrane potential. The cytoplasm is electrically negative (typically –60 to –80 mV) relative to the extracellular fluid (Fig. 3-3).
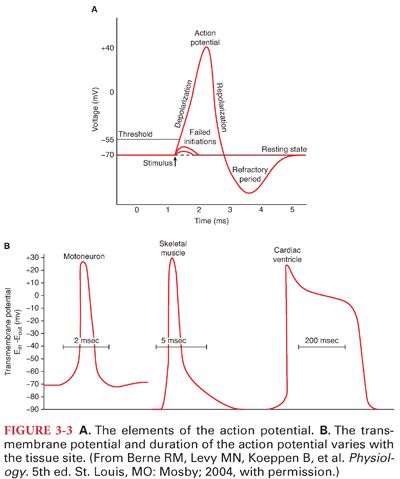
3. An action potential is the rapid change in transmembrane potential due to the opening of sodium channels (depolarization) and rapid influx of sodium ions down the concentration gradient, reversing the net negative charge within the cell. The membrane resting potential is restored by the closing of the sodium channels and the opening of potassium channels (repolarization) after the action potential has passed.
E. Propagation of action potentials along the entire length of a nerve axon is the basis of rapid signal transmission along nerve cells. The size and shape of the action potential varies among excitable tissues (see Fig. 3-3).
F. Abnormal Action Potentials
1. A deficiency of calcium ions in the extracellular fluid (hypocalcemia) prevents the sodium channels from closing between action potentials (tetany).
2. Low potassium ion concentrations in extracellular fluid increase the negativity of the resting membrane potential, resulting in hyperpolarization and decreased cell membrane excitability.
3. Local anesthetics decrease permeability of nerve cell membranes to sodium ions, preventing achievement of a threshold potential that is necessary for generation of an action potential.
G. Neurotransmitters and Receptors
1. Neurotransmitters are chemical mediators that are released into the synaptic cleft in response to the arrival of an action potential at the nerve ending. Neurotransmitter release is voltage dependent and requires the influx of calcium ions into the presynaptic terminals (see Fig. 3-2).
2. Neurotransmitters may be excitatory or inhibitory, depending on the ion selectivity of the protein receptor (Table 3-2).
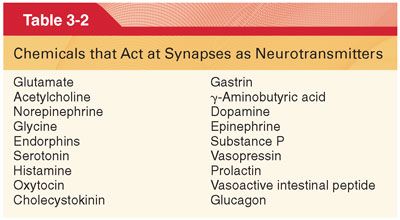
3. Volatile anesthetics produce a broad spectrum of actions, as reflected by their ability to modify both inhibitory and excitatory neurotransmission at presynaptic and postsynaptic loci within the CNS. The precise mechanism of these effects remains uncertain. It is likely that volatile anesthetics interact with multiple neurotransmitter systems by a variety of mechanisms.
H. G Protein–coupled Receptors (Fig. 3-4). The recognition site faces the exterior of the cell membrane to facilitate access of water-soluble endogenous ligands and exogenous drugs, whereas the catalytic site faces the interior of the cell.
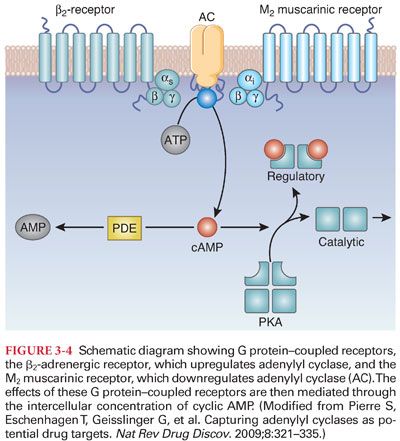
1. Gα proteins can either be stimulatory, promoting a specific enzymatic reaction within the cell, or inhibitory, depressing a specific enzymatic reaction.
a. β-adrenergic receptors couple with stimulatory Gαs proteins and increase the activity of adenylyl cyclase.
b. Opioid receptors are associated with inhibitory Gαi proteins that decrease the activity of adenylyl cyclase.
c. By regulating the level of activity of adenylyl cyclase, the β-adrenergic and opioid receptors modulate the internal level of cyclic adenosine monophosphate (cAMP), which functions as an intercellular second messenger (see Fig. 3-4).
2. Many hormones and drugs act through G protein–coupled receptors, including catecholamines, opioids, anticholinergics, and antihistamines.
a. Dopamine represents more than 50% of the CNS content of catecholamines, with high concentrations in the basal ganglia. Dopamine can be either inhibitory or excitatory, depending on the specific dopaminergic receptor that it activates. Dopamine is important to the reward centers of the brain and plays a key role in addiction and drugs.
b. Norepinephrine is present in large amounts in the reticular activating system and the hypothalamus, where it plays a key role in natural sleep and analgesia.
c. Substance P is an excitatory neurotransmitter co-released by terminals of pain fibers that synapse in the substantia gelatinosa of the spinal cord.
d. Endorphins are endogenous opioid peptide agonists (act through the μ opioid receptor, the same receptor responsible for the effects of administered opioids).
I. Ion Channels. There are three basic types of ion channels: (a) ligand-gated ion channels ionotropic receptors, (b) voltage-sensitive ion channels, and (c) ion channels that respond to other types of gating.
1. Ligand-gated ion channels are complexes of protein subunits that act as switchable portals for ions (involved principally with fast synaptic transmission between excitable cells).
a. Excitatory ligand-gated ion channels cause the inside of the cell to become less negative typically by facilitating the influx of cations into the cell (acetylcholine, glutamate, serotonin).
b. Inhibitory ligand-gated ion channels cause the inside of the cell to become less negative, typically by facilitating the flux of chloride into the cell. Potassium channels that facilitate the efflux of potassium ions are also inhibitory (γ-amino butyric acid [GABA]), glycine).
2. Voltage-gated ion channels are complexes of protein subunits that act as switchable portals sensitive to membrane potential through which ions can pass through the cell membrane (open and close in response to changes in voltage across cell membranes).
a. Voltage-gated sodium channels are the site of local anesthetic action (local anesthetics block neural conduction by blocking passage of sodium through the voltage-gated sodium channel).
b. The human ether-a-go-go related gene (hERG) potassium channel is sensitive to many drugs and is responsible for sudden death from drugs that predispose the patient to torsades de pointes (inhibition of the hERG potassium channel is the reason for the black box warning on droperidol).
J. Receptor Concentration
1. Receptors in cell membranes are not static components of cells.
2. Excess circulating concentrations of ligand often results in a decrease in the density of the target receptors in cell membranes (excessive circulating norepinephrine in patients with pheochromocytoma leads to downregulation of β-adrenergic receptors).
K. The Synapse
1. Structure
a. The synapse functions as a diode that transmits an action potential from the presynaptic membrane to the postsynaptic membrane across the synaptic cleft (Fig. 3-5).
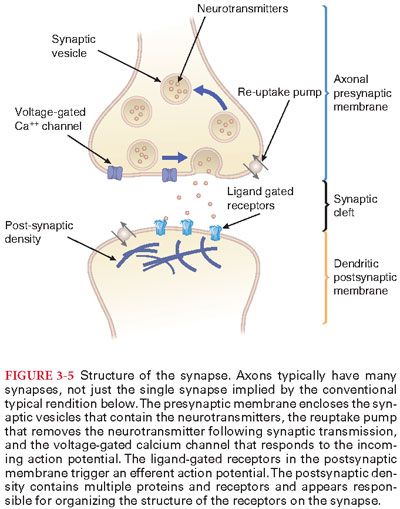
b. Calcium triggers the fusion of the vesicle to the cell membrane and the release of the neurotransmitter into the synaptic cleft through exocytosis, resulting in the extrusion of the contents of the synaptic vesicles.
2. Synaptic Modulation. The resting transmembrane potential of neurons in the CNS is about –70 mV, less than the –90 mV in large peripheral nerve fibers and skeletal muscles.
3. Synaptic delay reflects the time for release of the neurotransmitter from the synaptic varicosity, diffusion of the neurotransmitter to the postsynaptic receptor, and the subsequent change in permeability of the postsynaptic membrane to various ions.
4. Synaptic fatigue is a decrease in the number of discharges by the postsynaptic membrane when excitatory synapses are repetitively and rapidly stimulated (decreases excessive excitability of the brain as may accompany a seizure, thus acting as a protective mechanism against excessive neuronal activity).
a. The mechanism of synaptic fatigue is presumed to be exhaustion of the stores of neurotransmitter in the synaptic vesicles.
b. Synaptic fatigue is unmasked at the neuromuscular junction in myasthenia gravis when the enormous reserve for neuromuscular transmission is limited by either pre- or postsynaptic autoimmune damage.
5. Factors that Influence Neuron Responsiveness. Neurons are highly sensitive to changes in the pH of the surrounding interstitial fluids (alkalosis enhances neuron excitability and acidosis depresses neuron excitability).
II. Central Nervous System. The brain, brainstem, and spinal cord constitute the central nervous system (CNS) (Fig. 3-6).
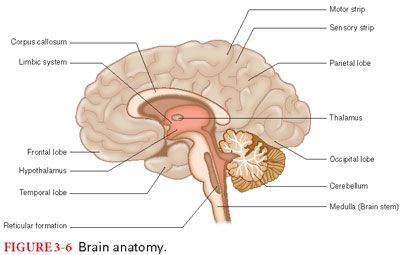
A. Cerebral Hemispheres. The two cerebral hemispheres, known as the cerebral cortex, constitute the largest division of the human brain. Regions of the cerebral cortex are classified as sensory, motor, visual, auditory, and olfactory, depending on the type of information that is processed. Frontal, temporal, parietal, and occipital designate anatomic positions of the cerebral cortex (Fig. 3-7).
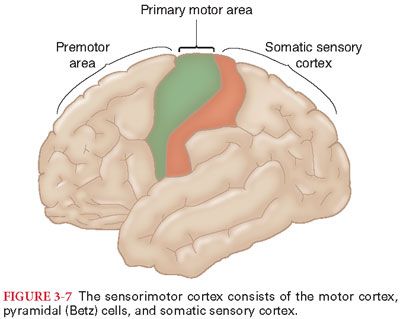
B. Anatomy of the Cerebral Cortex
1. Topographic Areas. The area of the cerebral cortex to which the peripheral sensory signals are projected from the thalamus is designated the somesthetic cortex (see Fig. 3-7). The motor cortex is organized into topographic areas corresponding to different regions of the skeletal muscles.
2. Corpus Callosum. The two hemispheres of the cerebral cortex, with the exception of the anterior portions of the temporal lobes, are connected by fibers in the corpus callosum. The corpus callosum and anterior commissure make information processed or stored in one hemisphere available to the other hemisphere.
C. Dominant versus Nondominant Hemisphere. Language function and interpretation is typically localized in the dominant cerebral hemisphere, whereas spatiotemporal relationships (ability to recognize faces) are localized in the nondominant hemisphere. Destruction of the dominant cerebral hemisphere in adults results in loss of nearly all intellectual function.
D. Memory. The cerebral cortex, especially the temporal lobes, serves as a storage site for information that is often characterized as memory.
1. Short-Term Memory. The favored explanation for short-term memory is posttetanic potentiation (tetanic stimulation of a synapse for a few seconds causes increased excitability of the synapse that lasts for seconds to hours).
2. Long-Term Memory. Long-term memory depends on stable synaptic changes that are induced by experience. The stability of this system is evidenced by total inactivation of the brain by hypothermia or anesthesia without detectable significant loss of long-term memory.
a. Long-term memory is thought to rely on long term synaptic potentiation mediated by structural changes.
b. The mechanism often involves increased expression of N-methyl-D-aspartate (NMDA) receptors and voltage-gated calcium channels in the postsynaptic neuron.
E. Postoperative cognitive dysfunction (impaired memory) persisting after months has been described in 10% of elderly patients receiving general anesthesia without known arterial hypoxemia or systemic hypotension.
F. Awareness and Recall During Anesthesia
1. The incidence of awareness with recall (conscious memory) following general anesthesia has been estimated at between 1 and 5 in 1,000 general anesthetics, depending on the risk group.
2. Although the incidence of conscious recall of intraoperative events is rare and the development of post-traumatic stress disorder is even more uncommon, the fact that approximately 20 million general anesthetics are administered annually in the United States would correspond to 26,000 cases of awareness (0.13% of approximately 20 million) each year.
3. The use of neuromuscular blockade is a risk factor for awareness under general anesthesia, particularly, awareness that is associated with memories of pain and complicated by posttraumatic stress disorder.
4. Many cases of conscious awareness during surgery can be attributed to intentionally or unintentionally low concentrations of administered anesthetic.
5. Recognizing Awareness. Despite a variety of monitoring methods, awareness may be difficult to recognize in real time. Indicators of awareness (heart rate, blood pressure, and skeletal muscle movement) are often masked by anesthetic and adjuvant drugs (β-adrenergic blockers) and/or neuromuscular-blocking drugs. Several different monitors based on analysis of electroencephalogram and somatosensory evoked potentials (SSEP) patterns have been introduced in hopes of addressing this issue.
G. Brainstem. Homeostatic life-sustaining processes are controlled subconsciously in the brainstem (control of systemic blood pressure and breathing in the medulla).
H. Limbic System and Hypothalamus. Behavior associated with emotions is primarily a function of structures known as the limbic system (hippocampus, basal ganglia) located in the basal regions of the brain.
I. Basal Ganglia
1. Many of the impulses from basal ganglia are inhibitory, mediated by dopamine and GABA. The balance between agonist and antagonist skeletal muscle contractions is an important role of the basal ganglia.
2. Whenever destruction of the basal ganglia occurs, there is associated skeletal muscle rigidity.
J. Reticular activating system is a polysynaptic pathway (excitatory and inhibitory) that is intimately concerned with electrical activity of the cerebral cortex. The reticular activating system determines the overall level of CNS activity, including nuclei important in determining wakefulness and sleep.
1. Slow-Wave Sleep. During slow-wave sleep, sympathetic nervous system activity decreases, parasympathetic nervous system activity increases, and skeletal muscle tone is greatly decreased. As a result, there is a 10% to 30% decrease in systemic blood pressure, heart rate, breathing frequency, and basal metabolic rate.
2. This form of sleep is characterized by active dreaming, irregular heart rate and breathing, and a desynchronized pattern of low-voltage β-waves on the EEG similar to those that occur during wakefulness (but not aware). Despite the inhibition of skeletal muscle activity, the eyes exhibit rapid movements (desynchronized sleep is also referred to as paradoxical sleep or rapid eye movement [REM] sleep).
K. Cerebellum operates subconsciously to monitor and elicit corrective responses in motor activity caused by stimulation of other parts of the brain and spinal cord. The cerebellum is important in the maintenance of equilibrium and postural adjustments.
1. Dysfunction of the Cerebellum
a. In the absence of cerebellar function, a person cannot predict prospectively how far movements will go (results in overshoot of the intended mark referred to as past pointing).
b. In the presence of cerebellar disease, a person is unable to activate antagonist skeletal muscles that prevent a certain portion of the body from moving unexpectedly in an unwanted direction.
L. Spinal cord extends from the medulla oblongata to the lower border of the first and, occasionally, the second lumbar vertebra. Below the spinal cord, the vertebral canal is filled by the roots of the lumbar and sacral nerves, which are collectively known as the cauda equina.
1. Gray matter of the spinal cord functions as the initial processor of incoming sensory signals from peripheral somatic receptors and as a relay station to send these signals to the brain. Anatomically, the gray matter of the spinal cord is divided into anterior, lateral, and dorsal horns consisting of nine separate laminae that are H-shaped when viewed in cross-section (Fig. 3-8).
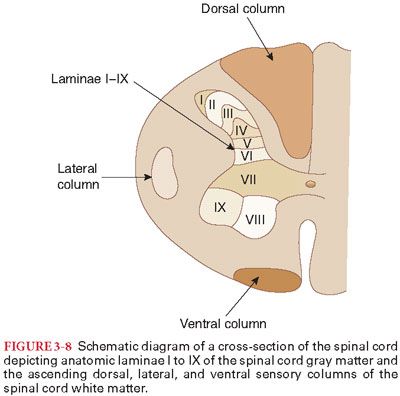
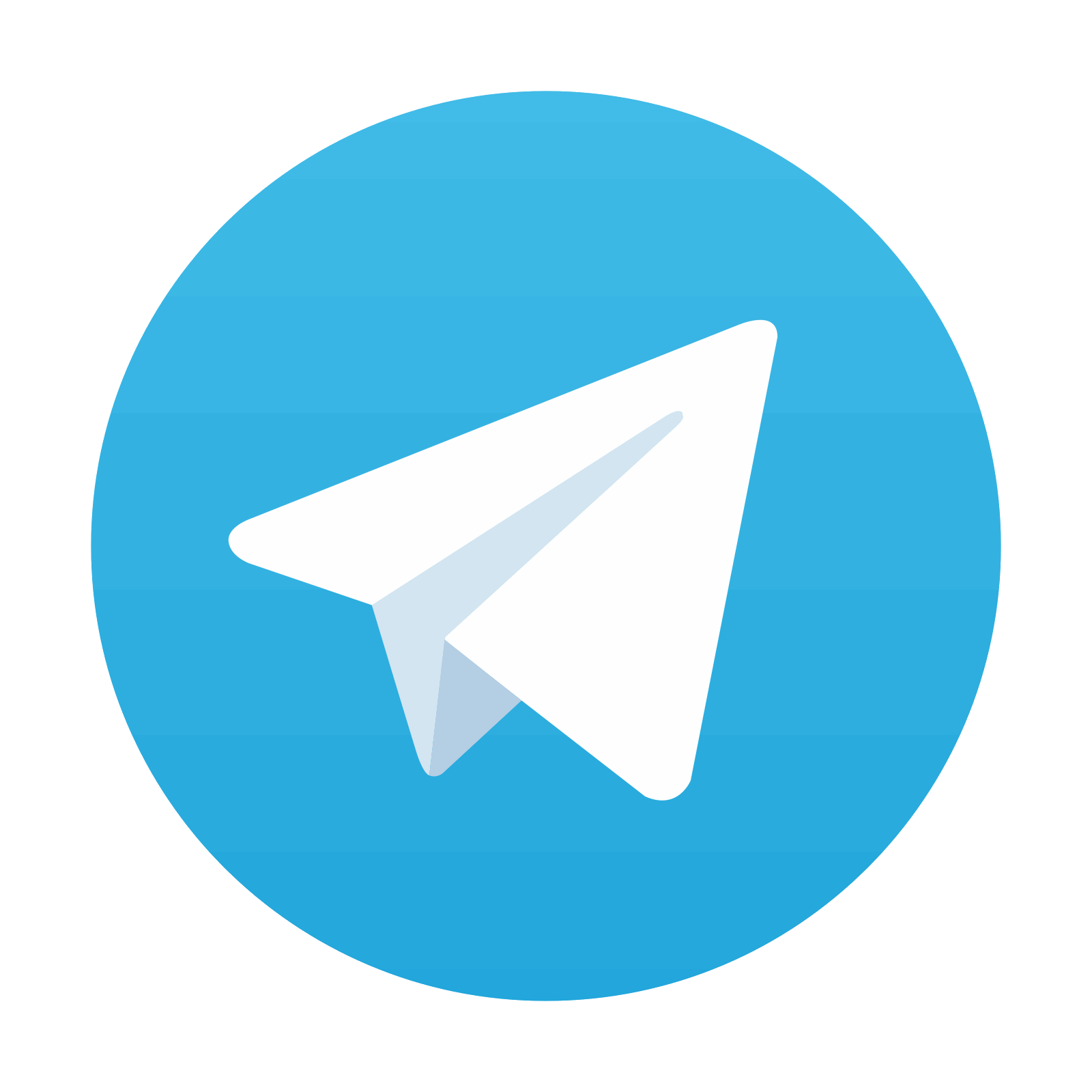
Stay updated, free articles. Join our Telegram channel

Full access? Get Clinical Tree
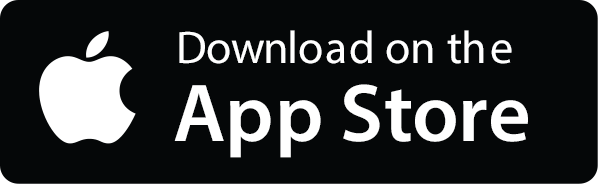
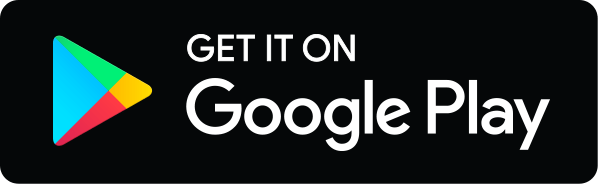