CONTENTS
10.2 Anatomy and Physiology of the Nasal Cavity: Implications for Drug Delivery
10.2.2 Mucus and the Mucociliary Escalator
10.3 Overview of Nasal Drug Delivery
10.3.1 Limiting Factors for Nasal Drug Delivery
10.3.1.1 Small Size of the Nasal Cavity
10.3.1.2 Constraints of Nasal Geometry: Access and Penetration Difficulties
10.3.1.3 Mucus and Mucociliary Clearance
10.3.1.8 Patient Acceptability and Compliance
10.3.2 Nasal Deposition and Clearance
10.4 Nasal Drug Delivery Devices
10.4.2 Pressurized Metered-Dose Inhalers
10.4.6 Breath Powered™ Bi-Directional™ Nasal Device: A Case Study
10.5 Nasal Formulation Factors
10.5.3 Mucoadhesives and Ciliastatics
10.5.4 Other Formulation Excipients
10.5.5 Micro- and Nanoparticulate Drug Delivery Systems
10.6 Nose-to-Brain Drug Delivery
Nasal drug delivery is ideally suited to the topical treatment of local nasal conditions, such as the common cold, allergic and nonallergic rhinitis, nasal polyps, and chronic nasal and sinus inflammations. Drugs for topical delivery include antihistamines (e.g., azelastine), anti-inflammatory corticosteroids (e.g., budesonide and fluticasone), and topical nasal decongestants (e.g., oxymetazoline and xylometazoline). The idea is that delivered locally, these drugs are effectively targeted to their site of action, which should maximize their therapeutic effect while minimizing unwanted side effects. However, as described in this chapter, current delivery devices are in fact not very effective at targeting to the posterior nasal cavity; drug delivery to this region requires optimization.
The nasal route is also increasingly used as a noninvasive route for systemic delivery, particularly when rapid systemic absorption and clinical effect are desired, for example, in the rapid relief of a migraine attack. Marketed nasal antimigraine drugs include sumatriptan (Imitrex® nasal spray), zolmitriptan (Zomig® nasal spray), and dihydroergotamine mesylate (Migranal® nasal spray). A further example is the intranasal (IN) delivery of opiates, such as fentanyl (Lazanda® and Instanyl®), when rapid pain relief is required. In addition, nasal delivery has become a useful alternative for systemic drug absorption in situations where the gastrointestinal (GI) route is unfeasible, such as for patients with nausea, vomiting, and gastric stasis (frequent in migraine patients); or patients with swallowing difficulties, such as children and elderly; or those who suffer from dry mouth.
The nasal route is suitable for drugs with poor oral bioavailability, due to, for example, GI instability, poor and delayed oral absorption, and drugs that undergo extensive first-pass effects in the gut wall or liver. A variety of peptide and protein drugs that demonstrate poor oral bioavailability are capable of systemic absorption via the nasal route, and a number of commercially available preparations are on the market, including for nafarelin (Synarel®), salmon calcitonin (Miacalcin®, Roritcal®), oxytocin (Syntocinon®), desmopressin (Desmospray®), and buserelin (Suprecur®). Similarly, “biologic” drugs such as monoclonal antibodies and antisense DNA demonstrate poor oral bioavailability and currently must be given by injection. These molecules are unlikely to realize their full clinical potential unless the patient can easily and conveniently self-administer the drug. The nasal route has emerged as a highly promising alternative epithelial route for the systemic delivery of these drugs.
The nasal route may also be used as an alternative to injections for the administration of vaccines, potentially including immunotherapeutics. For example, FluMist®, a nasal vaccine to protect against influenza, has been commercially available since 2003. A further possibility of the nasal route is as a portal of entry for drugs into the central nervous system (CNS). The barrier between the blood and the brain (the blood–brain barrier [BBB]) plays a vital role in protecting the delicate milieu of the brain, but also prevents CNS therapeutics from gaining access. Exploitation of a direct “nose-to-brain” (N2B) pathway, which bypasses the BBB, could therefore facilitate the treatment of numerous disabling psychiatric and neurodegenerative disorders, as well as brain cancers.
10.2 ANATOMY AND PHYSIOLOGY OF THE NASAL CAVITY: IMPLICATIONS FOR DRUG DELIVERY
The anatomy of the nose is shown in Figure 10.1. It extends 6–9 cm from the nostrils to the nasopharynx (throat) and is subdivided into left and right sides by a vertical partition, the nasal septum. The anterior portion of the nasal cavity, the nasal vestibule, then narrows into a triangular-shaped slit, the nasal valve, located approximately 1.5–2.5 cm from the nostril. Beyond the valve region, the posterior cavity is characterized by groove-like air passages (meatuses), formed by the scrolllike projections of the superior, middle, and inferior turbinates (conchae), which extend out from the lateral walls, almost reaching the septum.
Specific anatomical and physiological features of the nasal cavity have important implications for nasal drug delivery and targeting, and are discussed in more detail here.
The complex anatomical features of the nose are designed to facilitate its role in protecting the lower airways by filtering, warming, and humidifying the inhaled air. When air enters the nostrils, it passes first through the nasal vestibule, which is lined by the skin containing vibrissae (short, coarse hairs) that filter out large dust particles. The nasal valve is the narrowest portion of the nasal passage, with a mean cross-sectional area of only about 0.6 cm2 on each side. It is the primary regulator of airflow and resistance, accounting for up to 80% of nasal resistance, and almost half of the total resistance of the entire respiratory system. With increasing inspiratory flow rate, the action of Bernoulli forces progressively narrow the valve. The valve can even close completely with vigorous sniffing. Beyond the valve, the nasal cavity comprises a set of narrow, warrenlike passageways, the meatuses. The turbinates introduce turbulence to the airflow, forcing it through the narrow meatuses and ensuring maximal contact between the incoming air and the mucosal surface. The dense vascular capillary bed directly beneath the mucosal surface, in addition to the mucus layer and nasal secretions, ensures that the incoming air is warmed and humidified. The arrangement of conchae and meatuses also increases the available surface area of the internal nose and prevents dehydration by trapping water droplets during exhalation.
10.2.2 MUCUS AND THE MUCOCILIARY ESCALATOR
Goblet cells in the mucosa secrete mucus, a complex, thick, viscoelastic gel, containing specific glycoproteins known as mucins, which give the secretions their characteristic viscosity and elasticity. Mucos also contains proteins, lipids, and antibacterial enzymes (such as lysozyme and immunoglobulins). The secreted mucosal blanket lies over the nasal epithelium and functions as a protective barrier against the entry of pathogens. The underlying epithelial cells are ciliated, and the mucus works in tandem with the ciliated cells, in an extremely efficient self-cleansing mechanism known as the “mucociliary escalator.” In this process, inhaled particulates (dust, pollutants, microbes, etc.) become trapped in the sticky mucus layer. The underlying cilia beat in unison, via an ATP energy–dependent process, propelling the mucus layer toward the throat. The ciliary movement can be considered a form of rhythmic waving, which enables hooklike structures at the ciliary tips to propel mucus along. The ciliary beat frequency is in the range of about 100 strokes per minute. At the throat, entrapped particles are removed, either by being swallowed or expectorated.
The nasal vestibule is lined by nonciliated, stratified squamous epithelium, which gradually transitions in the valve region into a ciliated, pseudostratified, columnar epithelium with goblet cells (see also Chapter 4, Figure 4.9). Mucus secreted by the goblet cells lies over the epithelium as a protective layer, and the mucociliary escalator filters the incoming air. In addition to cilia, the epithelial cells contain microvilli, which increase the available surface area.
Another important function of the nose is olfaction and, as such, the nasal cavity also contains a specialized olfactory epithelium, located in the roof of the nasal cavity. The olfactory epithelium and a direct olfactory pathway to the brain are described further in Section 10.6, in the context of N2B delivery (Figure 10.4).
10.3 OVERVIEW OF NASAL DRUG DELIVERY
As outlined in Section 10.1, nasal drug delivery is highly versatile and can be used for a variety of delivery options, including (1) for local delivery to the nose and (2) as a noninvasive route for systemic delivery, (3) for nasal immunization and vaccine delivery, and (4) for delivery of drugs to the CNS, via N2B delivery. The nasal route offers a number of advantages for these various delivery options, outlined here.
1. An expanded surface area: The area available for absorption is enhanced by the labyrinthine passages of the nasal cavity, as well as the microvilli present on the apical surface of the epithelial cells. The total surface area of the nasal cavity is about 160 cm2 (although the effective surface area for absorption is influenced by the type of dosage form used to deliver the drug, and other factors).
2. Permeable epithelium: The epithelium of the nose is described in Chapter 4 (Figure 4.9). It is more permeable than that of the GI tract. For example, molecules larger than 500 Da are too large to permeate the GI tract via transcellular passive diffusion, whereas the cutoff molecular weight for nasal transcellular diffusion seems to be about 1000 Da. This enhanced permeability, coupled with reduced metabolic activity, accounts for the number of commercially available preparations for the systemic delivery of peptide drugs via the IN route, as listed in Section 10.1.
3. Rich blood supply: The nasal mucosa is highly vascularized, with specialized capacitance vessels that facilitate heat exchange and other physiological roles. The rich blood supply promotes the rapid absorption of drug molecules and is especially useful when rapid onset is desirable, for example, in the treatment of migraine attacks or breakthrough pain (BTP) in cancer.
4. Relatively low enzymatic activity: Nasal secretions possess a wide range of enzymes, including proteases such as neutral endopeptidase, aminopeptidase peroxidase, and caboxypeptidase N. Furthermore, intracellular enzymes are found in the epithelial cells lining the cavity. Nevertheless, the metabolic activity of the nose is relatively low, in particular in comparison with other epithelial sites such as the GI tract, so that enzymatic activity is not considered a substantial obstacle to nasal drug delivery. Hepatic first-pass metabolism is also avoided via this route.
5. Accessibility and compliance: The nasal route is easily accessible and nasal delivery devices (sprays, drops, etc.) tend to be unobtrusive and relatively easy to use. The route is noninvasive, with all the associated advantages over parenteral delivery, e.g., no needles/sharps and associated biohazard/disposal issues, less need for specialized medical personnel to administer the dose, nasal formulations do not normally require cold storage or have to be sterile.
6. Suitability for controlled release: Various types of nasal drug delivery systems (DDS) and formulation additives can allow for a sustained release of drug over time. Although in general, it should be noted that the nose is not particularly suited for sustained-release purposes.
7. Delivery to the CNS: The specialized olfactory epithelium of the nose offers a potential route for direct N2B drug delivery.
8. Delivery to the lymphoid tissue: As described in Section 10.7, the nasal-associated lymphoid tissue (NALT) makes the nose a highly effective immunological site, making the route an attractive one for the delivery of vaccines.
10.3.1 LIMITING FACTORS FOR NASAL DRUG DELIVERY
The same anatomical and physiological features that enable the many vital functions of the nose also impose substantial hurdles for efficient nasal drug delivery (Djupesland 2013; Djupesland et al. 2014). These barriers are considered here.
10.3.1.1 Small Size of the Nasal Cavity
The small size of the nasal cavity limits the volume of a liquid formulation that can be administered. Typically, volumes about 100–200 μL in each nostril are possible. A larger volume can drip back out of the nostril, which can cause discomfort and embarrassment. Drip-out also reduces the actual fraction of dose retained in the cavity, thereby introducing variability in the administered dose. Throat run-off is a further problem: high liquid volumes can “flood” the nasal cavity so that the dose runs off to the throat and is swallowed, resulting in drug loss and a bitter aftertaste, which can adversely affect patient compliance. Drugs with low aqueous solubility and/or requiring high doses can therefore present a problem for IN delivery.
10.3.1.2 Constraints of Nasal Geometry: Access and Penetration Difficulties
The complex geometry of the nose and the associated turbulent airflow present multiple barriers to the access and penetration of drug molecules. Initial entry is limited by the small size of the nasal vestibule. Beyond the vestibule, the narrow, slit-like, nasal valve constitutes a major, and often ignored, challenge to successful nasal drug delivery and targeting. As described earlier, the narrow valve becomes even narrower during inhalation, and it can close entirely during sniffing. Beyond the valve, the posterior nasal cavity comprises a complex labyrinthine series of tunnels and passageways, with turbulent airflow, which makes deep penetration further into the cavity very difficult.
Drug delivery from conventional nasal delivery devices (sprays, drops, pressurized metered-dose inhalers [pMDIs], nebulizers, and powder sprayers) is described in detail in the following texts, but it should be pointed out here at the outset that drug delivery from all the currently available conventional devices is suboptimal. A common feature of all these devices is their limited ability to deliver drug past the nasal valve, resulting in a large fraction of the dose being deposited in the anterior region of the nose (Aggarwal et al. 2004; Djupesland et al. 2014). Drug deposited in the anterior cavity is subject to loss via drip out and run off to the throat; further elimination occurs via sneezing, mechanical wiping, and ingestion. Anterior deposition also causes patient annoyance and discomfort or, more seriously, irritation and crusting of the tissue (Waddell et al. 2003). From a drug delivery perspective, deposition in the anterior cavity means that the drug is delivered to a limited, restricted portion of the nose, but fails to reach the much larger, highly vascular, expanded surface area of the posterior cavity.
Drug molecules that do succeed in penetrating the valve tend to enter the posterior cavity via the wider, lower part of the triangular-shaped valve, thereby gaining access to the floor of the nasal cavity. As such, the formulation tends to run off along the cavity floor to the throat but has limited exposure to the rest of the posterior cavity (Djupesland 2013).
Access and penetration difficulties are compounded in those pathological states that are associated with a hypersecretion of mucus, such as in inflammatory conditions and allergies. As described in Section 10.6, the specialized olfactory region of the nose associated with N2B delivery is located far beyond the valve, up in the roof of the nasal cavity (Figure 10.4). Accessing this area for the delivery of CNS therapeutics is also a formidable challenge.
10.3.1.3 Mucus and Mucociliary Clearance
The mucus layer and the process of mucociliary clearance present a number of obstacles for nasal drug delivery. For systemically acting drugs, the mucus layer presents a diffusional barrier for a drug in transit to the epithelial surface. The rate of diffusion of a drug through mucus depends on a number of factors, including the thickness and viscosity of the mucus layer, as well as the physicochemical properties of the drug. However, nasal mucus is only a few microns thick (in contrast, for example, to the GI tract, which has a mucus thickness of about 500 μm) and so does not present as substantial diffusional barrier here as compared to the GI tract and other mucosal sites.
Mucociliary clearance may limit the contact time of a drug molecule with the epithelial surface. The drug, instead of settling locally, can instead be removed to the throat, to be swallowed or expectorated. The mucociliary escalator moves the mucus blanket toward the nasopharynx at an average speed of 6 mm/minute, so that a particle deposited in the valve region of the nose is cleared to the nasopharynx within 15–20 minutes. Limited contact time with the absorbing surface may compromise drug absorption for a systemically active drug, or shorten drug–receptor interaction time for a locally acting drug.
If systemic absorption is the aim of therapy, the epithelial barrier must also be considered. Transport across epithelial barriers is described in detail in Chapter 4. To summarize here with respect to the nasal route, drug permeation via the paracellular route (i.e., between epithelial cells) is limited because of the tight junctional complexes that are present between cells. The predominant transport pathway across nasal epithelium is usually via the transcellular route, by means of transcellular passive diffusion. The rate of absorption is governed by Fick’s law (Chapter 4, Equation 4.1). The most important physicochemical drug factors affecting nasal absorption are as follows: (1) molecular weight (nasal absorption drops off sharply for drugs with a molecular weight > 1000 Da) and (2) lipophilicity (polar, hydrophilic, or ionized molecules exhibit poor permeability).
Thus, drugs currently administered nasally for systemic action generally comprise low molecular weight, lipophilic compounds. Although some peptides do show some systemic absorption (calcitonin, oxytocin, etc., see Section 10.1), the absolute bioavailability of peptide drugs via the nasal route is still relatively low. For example, the IN bioavailability of salmon calcitonin (MW 3432 Da) is only about 3%. Low bioavailability is compensated for by the extremely high potency of these drugs, which can produce therapeutic effects even at low plasma concentrations.
Mucosal sensitivity is a natural component of the nasal defense mechanism, but it also complicates nasal drug delivery, by making this area highly susceptible to irritation and injury. Exposure to chemicals, gases, particles, temperature, and pressure changes, as well as direct tactile stimuli, may cause nasal irritation, secretion, tearing, itching, sneezing, and severe pain. Nosebleeds, crusting, and potentially erosions or perforations may arise because of factors such as direct contact of the tip of a nasal spray nozzle during actuation and/or localized concentrated drug deposition on the septum. Formulation additives such as absorption enhancers (AEs) may also cause mucosal irritation or damage (see Section 10.5.1).
The nasal cycle is an alternating cycle of congestion and decongestion that occurs every 1–4 hours and is observed in at least 80% of healthy humans. This reciprocal autonomic cycling of mucosal swelling means that at any given time, even though the total combined resistance remains fairly constant, one of the nostrils is generally considerably more congested than the other, with most of the airflow passing through the passage of lesser congestion. This may be a challenge to efficient drug delivery. Therefore, for most indications, it would seem prudent to deliver the drug to both nasal passages, when administering a given dose (Djupesland 2013).
As described further in Section 10.3.2, nasal deposition and clearance are dependent on a number of complex, interrelated factors, including the type of nasal delivery device, drug physicochemical factors, formulation factors, and physiological, anatomical and pathological factors. These factors combine to introduce considerable variability with respect to the emitted dose, the site of deposition, the resulting clearance, and ultimately, the clinical response. The variability is particularly serious for drugs with a narrow therapeutic index (e.g., opiates and hormonal drugs), and represents a limitation of the route.
10.3.1.8 Patient Acceptability and Compliance
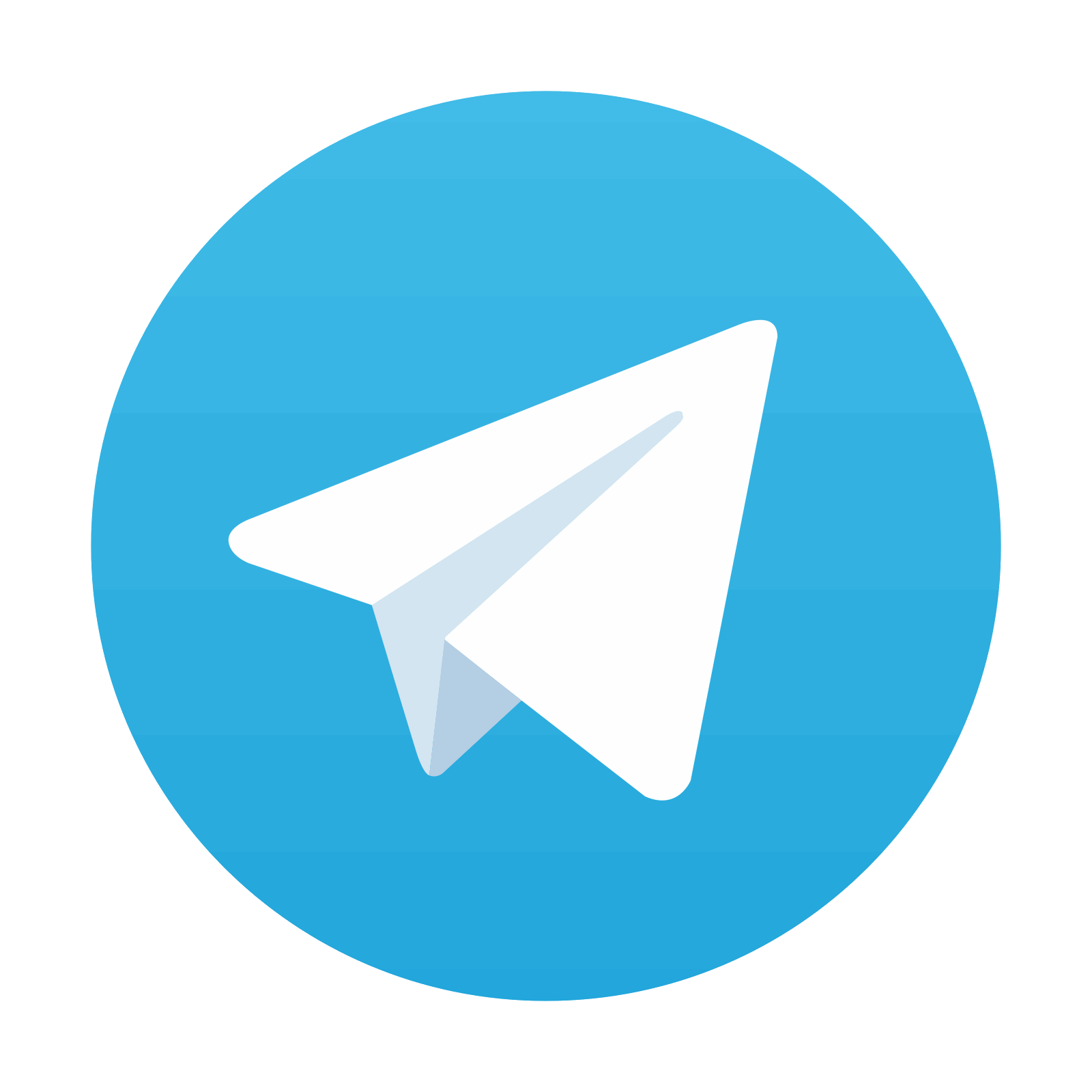
Stay updated, free articles. Join our Telegram channel

Full access? Get Clinical Tree
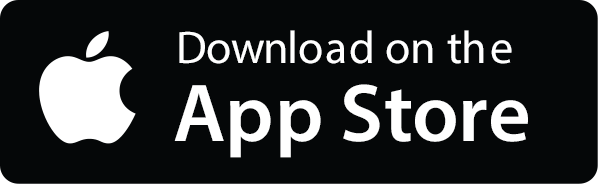
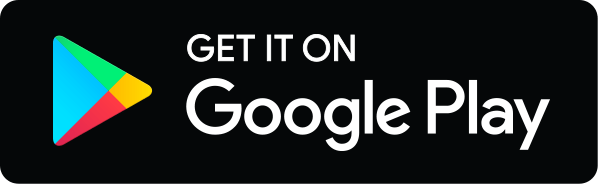