Chapter Twenty-Five. Muscle—the pelvic floor and the uterus
Introduction 349
Smooth muscle 357
Uterine muscle during pregnancy 357
Uterine shape and position: uterine growth 358
Hormonal influences on the uterus in pregnancy 358
The uterus at term 358
The decidua 358
The myometrium 359
The perimetrium 359
Cervical changes 359
Uterine blood flow 360
Innervation of the human uterus 360
Changes in the vagina in pregnancy 361
Uterine activity in pregnancy 361
Introduction
Three basic muscle types can be identified on the basis of structure, contractile properties and control mechanisms: skeletal muscle, smooth muscle and cardiac muscle. Most skeletal muscle is attached to bone and is responsible for supporting and moving the skeleton. Smooth muscle is found in the walls of the hollow viscera of the gastrointestinal, genitourinary and respiratory tracts and the specialised cardiac muscle propels blood through the circulation. Although there are significant differences in these types of muscle, the force-generating mechanism is similar in all of them.
All muscle cells are elongated and therefore referred to as fibres and they all contain two kinds of protein filaments: actin and myosin. Muscles have four functions: they produce movement, maintain posture, stabilise joints and generate heat (Marieb & Hoehn 2008). They have four properties: excitability, which is the ability to receive and respond to a stimulus; contractility, or the ability to shorten when stimulated; extensibility, or the ability to be stretched or extended beyond its resting length; and elasticity, or the ability of muscle to recoil back to its resting length.
Skeletal muscle
Skeletal muscles, as the name implies, are attached to and cover the bony skeleton. The longest muscle fibres are found in skeletal muscle. One striking feature of the fibres is obvious bands or striations, hence the term striated muscle. Another term used for skeletal muscle is voluntary as this is the only muscle type under voluntary control. Skeletal muscle can contract rapidly but tires easily and must be rested after short bursts of activity. Each skeletal muscle is a discrete organ made up of multiple muscle fibres. Other tissues found in the individual muscles include connective tissue, blood vessels and nerve fibres. Muscle fibres are gathered into functional units by a network of fibrous connective tissue. This connective tissue condenses into the tendons which form the muscular origins and insertions onto bone.
The activity of skeletal muscle depends on its rich blood supply and nerve supply. While the other muscle types can contract without nerve stimulation, each skeletal muscle fibre is supplied with a nerve ending. The blood supply is essential to deliver the large amounts of oxygen and nutrients and to remove equally large amounts of metabolic waste. The smaller blood vessels are long and winding, which permits the changes in muscle length to occur.
Microscopic anatomy of a skeletal muscle fibre
Skeletal muscle fibres are long cylindrical cells which taper at both ends. The plasma membrane is called the sarcolemma and there are multiple oval nuclei arranged just below the surface. Skeletal muscle fibres are huge. The fibre length is variable, ranging from a few millimetres (mm) in short muscles to lengths of 300 mm in longer muscles (1 ft in length). Their diameter ranges from 10 to 100 μm which is up to 10 times that of other body cells. The presence of multiple nuclei indicate that each muscle fibre is a syncytium (fusion of many cells) formed during embryonic development. The cytoplasm in muscle cells is called sarcoplasm and the endoplasmic reticulum is referred to as sarcoplasmic reticulum. The sarcoplasm contains large amounts of stored glycogen and a unique oxygen-binding protein called myoglobin similar to haemoglobin (Marieb & Hoehn 2008).
Myofibrils
Each muscle fibre contains a large number of rod-like myofibrils extending the full length of the cell and parallel to each other (Fig. 25.1). Myofibrils are densely packed and form 80% of the cellular content. Mitochondria, the energy-producing organelles, and other organelles are packed in between the myofibrils. Myofibrils are the contractile elements of the cell and each myofibril consists of smaller contractile units called sarcomeres. Several sarcomeres are arranged along each myofibril.
Myofibrils appear to be made of alternate dark or A bands and light or I bands, giving the characteristic striped appearance under the light microscope. The bands are named according to how they refract (bend) polarised light (light waves with a definite direction): A bands ( anisotropic) refers to their ability to refract light, depending on its angle; I bands ( isotropic) refers to their ability to refract light, whatever its angle (Martini & Nath 2009).
The A band is interrupted in mid-section by the highly refractive H zone ( H stands for helle, which means bright). Each H zone is bisected by a dark line called the M line. The I bands also have a midline interruption called the Z line (Figure 25.1 and Figure 25.2). A sarcomere is the region of a myofibril that extends from one Z line to the next. Brief explanations of these other features are as follows:
• The Z line is a network of interconnecting proteins that form a point of attachment for thin filaments.
• The H zone is only visible in relaxed muscle when the thick filaments are not overlapped by the thin filaments.
• The M line in the centre of the H zone appears darker because it is slightly thicker due to the presence of fine strands that connect adjacent thick filaments together.
![]() |
Figure 25.1 Intracellular tubular systems. (From Hinchliff S M, Montague S E 1990, with permission.) |
![]() |
Figure 25.2 Striated (A) and smooth (B) fibres. A, actin; M, myosin; FL, filaments. (Reproduced with permission from Huszar & Roberts 1982.) |
The higher magnifying power of the electron microscope shows that the A bands are formed of thick myosin filaments (Fig. 25.3). The thin filaments are formed of three different proteins: actin, troponin and tropomyosin. These run the length of the I band, overlapping the thick filaments (Fig. 25.4). In an intact muscle fibre the bands are aligned horizontally across the width of the cell. Each myosin molecule has a rod-like tail (axis) and two globular heads (cross-bridges) that interact with special sites on the thin filaments. Each thick filament within a sarcomere contains about 200 myosin molecules.
![]() |
Figure 25.3 Structure of a thick filament. (From Hinchliff S M, Montague S E 1990, with permission.) |
![]() |
Figure 25.4 Structure of a thin filament. (From Hinchliff S M, Montague S E 1990, with permission.) |
The thin filaments are mainly composed of actin. Subunits called globular or G actin bear the active sites to which the myosin cross-bridges attach themselves during muscle contraction. The backbone of each actin molecule is formed by two strands of fibrous or F actin arranged in a helical structure. Regulatory proteins include tropomyosin, which spirals around the F actin to stiffen it. In resting muscle the orientation of the tropomyosin blocks the myosin-binding sites on the actin molecules. This prevents the formation of cross-bridges. Troponin actually consists of three polypeptides: one binds to actin, another to tropomyosin helping to position it on the actin, while the third binds calcium.
Intracellular tubular systems
The muscle cell is penetrated by two tubular systems (see Fig. 25.1), both ending near the A–I band junctions. One tubular system is called the transverse or T system, extending from the cell exterior into the sarcoplasm where they branch and terminate. The other is the internal system formed by fine tubules called sarcotubules of the sarcoplasmic reticulum. These sarcotubules end in terminal sacs called the terminal cysternae. Both systems play a key role in muscle contraction. T tubules conduct electrical stimuli deep within the muscle cell to the sarcomeres. The sarcoplasmic reticulum regulates the calcium (Ca 2+) ions.
Muscle contraction
There are several theories of how muscle fibres contract but most evidence supports the sliding filament theory. The theory explains that during contraction the thin filaments slide past the thick ones, increasing the amount of overlap. This allows the thin filaments to penetrate more deeply into the central region of the A band. Overlapping is brought about by the cross-bridges of the sarcomeres acting simultaneously as a ratchet to pull the thin filaments towards the centre of the sarcomeres. This results in shortening of the muscle cell. The myosin heads are said to ‘walk up’ the actin filaments step by step from one binding site to the next (Fig. 25.5). This requires the presence of calcium, which binds to the troponin to form a complex. In the absence of calcium ions, tropomyosin blocks access to the myosin-binding site of actin. When calcium binds to troponin, the positions of troponin and tropomyosin are altered on the thin filament and myosin then has access to its binding site on actin. Thus the configuration of tropomyosin is changed to move it away from the myosin-binding sites. As calcium is removed by the sarcoplasmic reticulum, the contraction comes to an end and the muscle cell relaxes.
![]() |
Figure 25.5 Diagrammatic representation of the sliding-filament theory showing how the thick filaments of skeletal muscle move relative to one another as cross-bridges are formed and broken. (From Hinchliff S M, Montague S E 1990, with permission.) |
Regulation of contraction
Skeletal muscles contract in response to nerve stimulation, which results in an action potential being sent along the sarcolemma. This electrical event results in a rise in intracellular calcium (Ca 2+) ion levels that triggers off the contraction. The axon of a lower motor neuron branches profusely as it enters the muscle and each mound-shaped unmyelinated axonal terminal forms a junction with a single muscle fibre, approximately in the middle of the cell. This is called a neuromuscular junction or motor end plate (Fig. 25.6). The plasma membrane of the axonal ending does not actually touch the muscle fibre; between them is a small fluid-filled extracellular space called the synaptic cleft. The action potential must be transmitted across the space and this happens by the release of the neurotransmitter substance acetylcholine (ACh) from small membranous sacs in the axon terminal called synaptic vesicles.
![]() |
Figure 25.6 The neuromuscular junction. (From Hinchliff S M, Montague S E 1990, with permission.) |
When a nerve impulse reaches the end of an axon, voltage-regulated calcium channels open and calcium flows in from the extracellular fluid (ECF). The entry of the calcium causes some of the synaptic vesicles to fuse with the plasma membrane and release ACh into the synaptic cleft. This process is called exocytosis. ACh diffuses across the synaptic cleft and attaches itself to ACh receptors on the sarcolemma. All plasma membranes are polarised with a voltage gradient (membrane potential) across the membrane. The inside of the cell is negative. This attachment of ACh molecules opens chemically regulated ion gates. The positively charged sodium ion (Na +) passes from its higher concentration in the ECF fluid down a gradient into the cell, leading to a slight decrease in the negative potential. This event, called depolarisation, allows a muscle cell action potential to be generated and to pass in all directions across the sarcolemma.
Repolarisation of the sarcolemma occurs following the wave of the muscle action potential when sodium channels close and potassium channels open. Potassium ions (K +) rapidly diffuse out of the cell into the ECF down a gradient to restore the negativity inside the cell. The normal ionic balance of sodium and potassium is restored during the refractory period when the muscle cannot respond to stimuli. After the release of ACh and its binding to the ACh receptors, it is quickly destroyed by the enzyme acetylcholinesterase. This prevents the muscle contraction lasting longer than the stimulus requires.
Excitation–contraction coupling
This refers to the process whereby the generation of an action potential is followed by activation of the contractile machinery in the myofibrils. It involves the exposure of the binding sites on the actin to allow the formation of cross-bridges. Energy released from ATP is used to fuel the muscle contraction. As action potentials continue to arrive, the process is repeated many times, allowing sustained muscle contraction. Calcium ions are continuously released and taken up by troponin. This also requires energy from ATP. However, muscles store very little ATP and the supply is soon exhausted. If contraction is to continue, ATP must be regenerated (Martini & Nath 2009). This can occur in three ways:
1. By the interaction of ADP with creatine phosphate.
2. By aerobic respiration.
3. By lactic acid fermentation.
The pelvic floor
The bony pelvis provides protection to the pelvic organs while the pelvic floor holds them in position. The pelvic floor is primarily composed of soft tissues which fill the outlet of the pelvis. The most important of these is the strong funnel-shaped diaphragm of muscle attached to the pelvic walls. The posterior part of the diaphragm of muscles lies higher than the anterior. Through it pass the urethra, vagina and anal canal (Fig. 25.7).
![]() |
Figure 25.7 The layers of the pelvic floor. (From Henderson C, Macdonald S 2004, with kind permission of Elsevier.) |
The pelvic floor consists of six layers of tissue. From the inside outwards:
1. Pelvic peritoneum.
2. Visceral layer of pelvic fascia thickened to form pelvic ligaments which support the uterus.
3. Deep muscles encased in fascia.
4. Superficial muscles encased in fascia.
5. Subcutaneous fat.
6. Skin.
Superficial muscles
These muscles lie external to the deep muscles and provide additional strength—a little like the webbing beneath the cushion on some chairs. They consist of:
• The transverse perinei.
• The bulbocavernosus.
• The ischiocavernosus.
• The external anal sphincter.
• The external urethral meatus, sometimes called the membranous sphincter of the urethra (Fig. 25.8).
![]() |
Figure 25.8 The perineal muscles. (From Henderson C, Macdonald S 2004, with kind permission of Elsevier.) |
The transverse perinei
One muscle arises from the inner surface of each ischial tuberosity of the pelvis and passes transversely to meet its fellow, inserting into the perineal body. Some fibres pass posteriorly to blend with the anal sphincter.
The bulbocavernosus
This arises in the centre of the perineum and fibres pass on either side of the vagina and urethra, encircling them both, to insert into the corpora cavernosa (body) of the clitoris just under the pubic arch. This muscle is responsible for erection of the clitoris and contraction of the vaginal walls.
The ischiocavernosus
A muscle runs from each ischial tuberosity along the pubic arch to the corpora cavernosa of the clitoris and fibres interweave with the membranous sphincter of the urethra.
External anal sphincter
This is a circle of muscle surrounding the anus formed by merging of muscle fibres from deep and superficial layers. The sphincter is attached behind the anus to the coccyx.
External urinary meatus
The membranous sphincter of the urethra is a weak and not too important muscle. It is composed of muscle fibres passing above and below the urethra and attached to the pubic bones. It is not a true sphincter since it is not circular, but it acts to close the urethra.
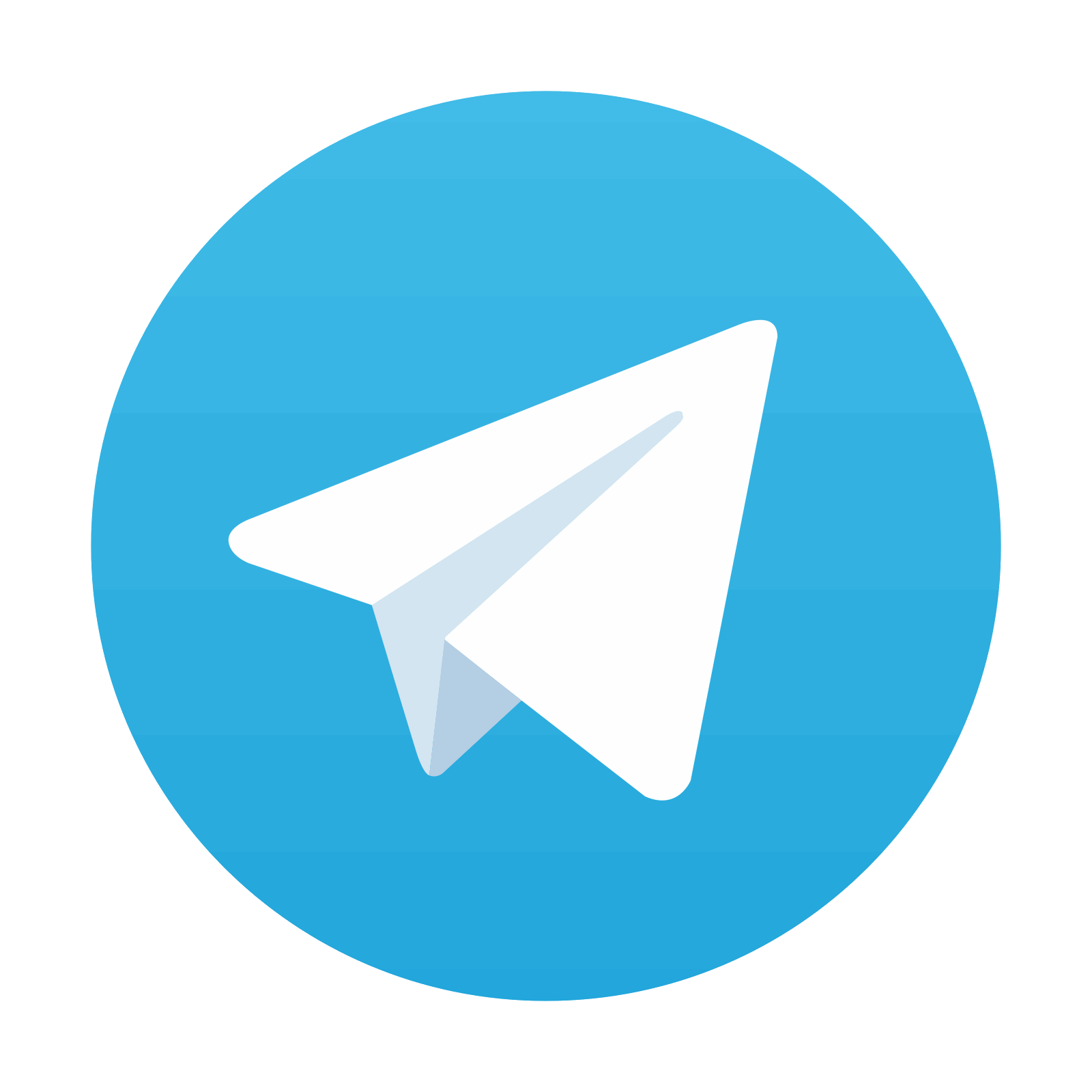
Stay updated, free articles. Join our Telegram channel

Full access? Get Clinical Tree
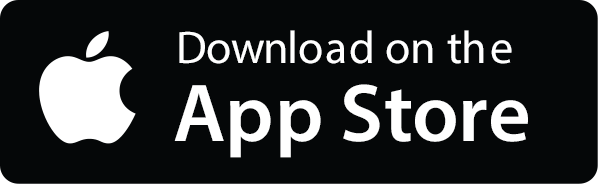
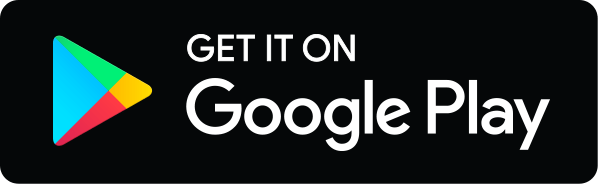
