Morphologic Response of Cells to Injury
The purpose of diagnostic cytology is to recognize processes that cause cell changes that are identifiable under the light microscope, supplemented, when necessary, by cytochemistry, immunocytochemistry, electron microscopy, or molecular biologic techniques (see Chaps. 2, 3, and 45). In this chapter the causes and effects of various forms of injury to the cells are discussed. Benign and malignant neoplasms (tumors) will be discussed in Chapter 7.
CAUSES OF CELL INJURY
Injury to the cells may be caused by numerous agents and disease states. A brief listing of the most significant sources of recognizable cell abnormalities observed in diagnostic cytology is as follows:
Physical and Chemical Agents
Heat
Cold
Radiation
Drugs and other chemical agents
Infectious Agents
Bacteria
Viruses
Fungi
Parasites
Internal Agents
Inborn, sometimes hereditary genetic defects of cell function
Storage diseases (e.g., Tay-Sachs and Gaucher’s diseases)
Metabolic disorders (e.g., phenylketonuria)
Faulty structure of essential molecules (e.g., sickle cell anemia)
Miscellaneous disorders
Diseases of the immune system
Inborn immune deficiencies
Acquired immune deficiencies
Autoimmune disorders
Disturbances of Cell Growth
Benign (self-limiting)
Hyperplasia
Metaplasia
Tumors or neoplasms (see Chap. 7)
Benign
Malignant
CELLULAR RESPONSE TO INJURY AT THE LIGHT MICROSCOPIC LEVEL
Cells have limited ability to express their response to injury. They may respond:
by dying (necrosis or apoptosis)
by undergoing a morphologic transformation that may be transient or permanent
by mitotic activity that again may be either transient or sustained, normal or abnormal, and may result in normal or abnormal daughter cells and subsequent generations of cells.
Although the mechanisms of cellular responses to injurious agents are still poorly understood because they are the result of complex molecular changes, it appears reasonable to assume that a cell will attempt to maintain its morphologic and functional integrity, either by mobilizing its own resources against injury, or by seeking assistance from other cells specializing in defensive action. The latter type of response is triggered by cell necrosis, a form of cell death, which results in an inflammatory process, with participation of leukocytes and macrophages. The significant morphologic responses of cells to various forms of injury are summarized below.
CELL DEATH
In cells, as in all other forms of life, death is an inevitable event. Death may follow a specific programmed pathway, or it may occur as an incidental event. Programmed cell death was first described and named apoptosis (from Greek, apo = from and ptosis = falling or sinking) by Kerr et al in 1972 (see also Searle et al, 1982 and Kerr et al, 1994). Apoptosis was first recognized as a purely morphologic phenomenon affecting cells, to be differentiated from necrosis, a form of cell death that occurred incidentally caused by an event or events not compatible with cell survival. The sequence of events in the two processes is compared in Figure 6-1. Within the recent years, apoptosis has received an enormous amount of attention from molecular biologists because of its importance in developmental biology and in a number of diseases, such as stroke and cancer.
Apoptosis
Morphology
The most significant studies of apoptosis in man have been conducted on cells in culture or on lymphocytes. There is comparatively little information on apoptosis in epithelial cells. Apoptotic cells are characterized by nuclear and cytoplasmic changes. The nuclear changes are a condensation of the nuclear chromatin, first as crescentic caps at the periphery of the nucleus, followed by further fragmentation and break-up of the nucleus (Fig. 6-1 top). The fragmentation of the chromatin into small granules of approximately equal sizes is known as karyorrhexis (from Greek, karyon = nucleus, rhexis = breakage), which has now been recognized as a manifestation of apoptosis (Fig. 6-2). The cytoplasm of many apoptotic cells may show shrinkage and membrane blisters. It appears, however, that the cytoplasm may remain relatively intact in squamous cells. As the next stage of cell disintegration, fragments of nuclear material with fragments of adjacent cytoplasm (that may contain various organelles) are packaged into membrane-enclosed vesicles (apoptotic bodies). These packages of cellular debris are phagocytized by macrophages, without causing an inflammatory reaction in the surrounding tissues. One important consequence of apoptosis is that the cell DNA is chopped up into fragments of variable sizes composed of multiples of 185 base pairs. When sorted out by electrophoresis, they form a “DNA ladder” of fragments of diminishing sizes. Because the breaks occur at specific points of nucleotide sequences, they can be recognized by specific probes identifying the break points in the DNA chain. The probes, either labeled with a fluorescent compound or peroxidase, allow the recognition of cells undergoing apoptosis, either by fluorescent microscopy, flow cytometry, or by microscopic observation (Li and Darzynkiewicz, 1999; Bedner et al, 1999). A so-called TUNNEL reaction is a method of documenting apoptosis in cytologic or histologic samples (Gavrieli et al, 1992; Li and Darzynkiewicz, 1999; Sasano et al, 1998).
Sequence of Biologic Events
In paraphrasing a statement by Thornberry and Lazebnik (1998), apoptosis is reminiscent of a well-planned and executed military operation in which the target cell is isolated from its neighbors, its cytoplasm and nucleus are effectively destroyed, and the remains (apoptotic bodies) are destined for burial at sea, leaving no traces behind. Much of the original information on the sequence of events in apoptosis was obtained by studying the embryonal development events in the small worm (nematode), Caenorhabditis elegans. These studies have documented that apoptosis occurs naturally during the developmental stages of the worm to eliminate unwanted cells. It is caused by a cascade of events, culminating in the activation of proteolytic enzymes that effectively destroy the targeted cell. A somewhat similar, but not identical, sequence of events was proposed for mammalian cells.
Apoptosis in mammalian cells is triggered by numerous injurious factors, some known, such as viruses, certain drugs, radioactivity, and some still unknown (summary in Thompson, 1995; Hetts, 1998; review in Nature, 2000). For example, the loss of T4 cells by the human immunodeficiency virus in acquired immunodeficiency syndrome (AIDS) is caused by apoptosis. However, the pathway to apoptosis is extremely complicated because normal cells contain genes that prevent it and genes that promote it. This equilibrium has to be disrupted for the cells to enter the cycle of death.
In brief, it is assumed today that “death signals,” received by the cytoplasm of the cell and mediated by a complex sequence of molecules, lead to activation of proteolytic enzymes, known as caspases that destroy the cytoplasmic proteins, including intermediate filaments, and attack the nuclear lamins, causing the collapse of the nuclear DNA structure. However, the intermediate steps of this sequence of events are enormously complicated. An injury to the molecule p53 (guardian of the genome; see Chap. 3) appears to be an important event (Bennett et al, 1998). Recent studies have documented that genes located on the mitochondrial membrane play a critical role in apoptosis (Brenner and Kroemer, 2000; Finkel, 2001). These genes belong to two families: Bcl, a protooncogene, which protects the cells from apoptosis, and Bax, which favors apoptosis (Zhang et al, 2000). If the proapoptotic molecule prevails, there is damage to the mitochondrial membrane with release of cytochrome C into the cytoplasm. Cytochrome C acts to transform a ubiquitous protein molecule known as zymogen into caspases.
Numerous articles on the subject of apoptosis have appeared in recent years, each addressing a small fragment of the complex puzzle. The key recent articles are cited or listed in the bibliography. The significance of apoptosis goes much beyond a simple morphologic and molecular biologic summary. It is generally thought that the mechanisms of apoptosis, besides playing a key role during embryonal development, may play a key role in cancer and in important degenerative processes such as Alzheimer’s disease. In cancer, suppression of apoptosis may be one of the causes of cell proliferation, so characteristic of this group of disorders. This may explain the role of oncogenes, such as Bcl or Myc, as protecting the cells from apoptosis. It is considered that changes or mutations in molecules controlling DNA damage in replication (such as p53) or molecules governing events in the cell cycle (such as Rb) play a role in these events. It has been proposed that, in degenerative disorders of the brain, such as Alzheimer’s disease, apoptosis may destroy essential centers of memory and control of body functions.
Necrosis
Cells may also die as a consequence of nonapoptotic events, globally referred to as necrosis. Some of the known causes of necrosis are exposure to excessive heat, cold, or cytotoxic chemical agents. There is considerably less information on this type of cell death than on apoptosis, and the main difference is the absence of typical morphologic changes and no evidence of activation of the cascade of events characterizing apoptosis (see Fig. 6-1 bottom).
Morphology
Cells undergoing nonapoptotic forms of necrosis may show extensive cytoplasmic vacuolization (Fig. 6-3). The nuclear changes include homogeneous, dense chromatin known as nuclear homogenization or pyknosis (from Greek, pyknos = thick), nuclear enlargement, and break-down of nuclear DNA, which however, does not form the DNA ladder, characteristic of apoptosis. Necrosis may result in destruction of the cell membranes, resulting in disintegration of the cell and formation of cell debris leading to an inflammatory process. The nuclear material may form fragments or streaks, often recognizable because they stain blue with the common nuclear dye, hematoxylin. Similar events may occur by physical injury to fragile cells if they are inappropriately handled during the technical preparation of
smears or other diagnostic material. In some cancers, the presence of nuclear necrosis is widespread and may be of diagnostic value (see oat cell carcinoma in Chap. 20).
smears or other diagnostic material. In some cancers, the presence of nuclear necrosis is widespread and may be of diagnostic value (see oat cell carcinoma in Chap. 20).
![]() Figure 6-3 Radiation effect on squamous cells. Huge cytoplasmic vacuoles signify cell death. (High magnification.) |
It is often quite impossible to determine morphologically whether a cell died as a consequence of apoptosis or necrosis. There is little doubt that there may be many pathways leading to cell death. What is significant, however, is the role played by necrotic cells as a trigger of inflammatory events, whereas cells dying of apoptosis, as a rule, do not cause any inflammatory reaction.
Sequence of Biologic Events
Cell necrosis may be caused by many of the types of cell injury listed in the opening page of this chapter. Thus, there is a significant overlap between the two modes of cell death. It is not known today why the differences in the mode of dying occurs if the trigger of cell death is the same. It is generally believed that cell necrosis may begin in a manner similar to apoptosis, that is, by activation of a cell membrane molecule or a “death signal,” which is followed by mitochondrial swelling, but this differs from the events in apoptosis because it does not lead to caspase activation (Green and Reed, 1998). Obviously, much is still unknown about cell necrosis, its mechanisms, and consequences.
OTHER EXPRESSIONS OF CELL RESPONSE TO INJURY
“Reactive” Nuclear Changes
It is not uncommon to observe, in material from various sources and under a variety of circumstances, but mainly in the presence of inflammatory processes, minor nuclear abnormalities such as slight-to-moderate nuclear enlargement, slight irregularities of the nuclear contour, increase in granularity of the chromatin, and occasionally the presence of somewhat enlarged nucleoli (Fig. 6-4). Such abnormalities are often classified as “reactive nuclear changes.” Virtually nothing is known about the mechanisms of such changes and their clinical significance is often puzzling. In many situations, such nuclear abnormalities occur in tissues adjacent to cancer. In cervical smears, the terms atypia of squamous cells of unknown significance (ASCUS) or atypia of glandular (endocervical) cells of unknown significance (AGUS) have been introduced to describe such phenomena. The term AGUS is no longer used. It is known that, in some patients with such changes a malignant lesion will be observed in the uterine cervix with the passage of time (see Chap. 11). Similar abnormalities may be observed in the so-called repair reaction and in metaplasia, discussed below. Thus, the term reactive nuclear changes is rather meaningless and reflects our ignorance of events leading to such nuclear abnormalities.
Multinucleation: Formation of Syncytia
It is not known why reaction to injury results in formation of multinucleated cells. These may occur as a consequence of a bacterial or viral infection, during a regenerative process, as in injured muscle, or for reasons that remain obscure. Multinucleation may be observed in cells of various derivations, such as macrophages, cells derived from organs of mesenchymal origin, or in epithelia.
The mechanism of formation of multinucleated cells by epithelioid cells was discussed in Chapter 5. Under unknown circumstances, apparently normal epithelial cells may form multinucleated giant cells or syncytia (from Latin, syn = together and cyto = cell) by cell fusion or endomitosis, that is, nuclear division not followed by division of the cytoplasm.
Regardless of mechanism of formation, such cells may be observed in the bronchial epithelium (see Chap. 19) and, occasionally, in other glandular epithelia (Fig. 6-5). In multinucleated cells caused by cell fusion, the cell membranes separating the cells from each other disappear. Multinucleation can be produced in vitro by the action of certain viruses, such as the Sendai virus, and in vivo in humans by herpesvirus and other viral infections. Thus, it is conceivable that the formation of true syncytia in epithelial cells is the reflection of a viral infection, although the causative agent may not be evident. To our knowledge, there is no known diagnostic or prognostic significance of the presence of
multinucleated epithelial cells. Such cell changes must not be confused with cell groupings or clusters, wherein cell membranes may not be visible under the light microscope, but are easily demonstrated by electron microscopy. The term syncytia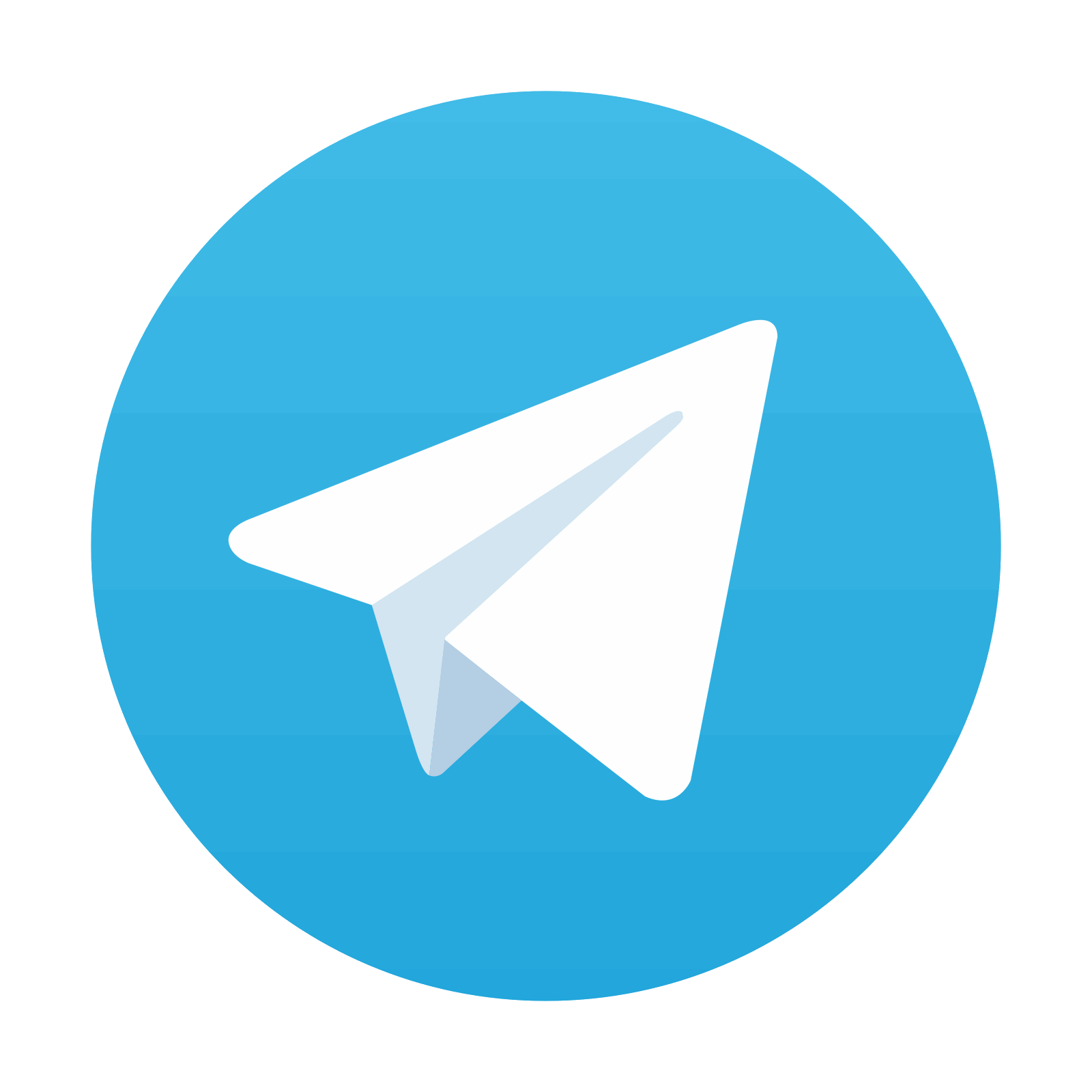
multinucleated epithelial cells. Such cell changes must not be confused with cell groupings or clusters, wherein cell membranes may not be visible under the light microscope, but are easily demonstrated by electron microscopy. The term syncytia
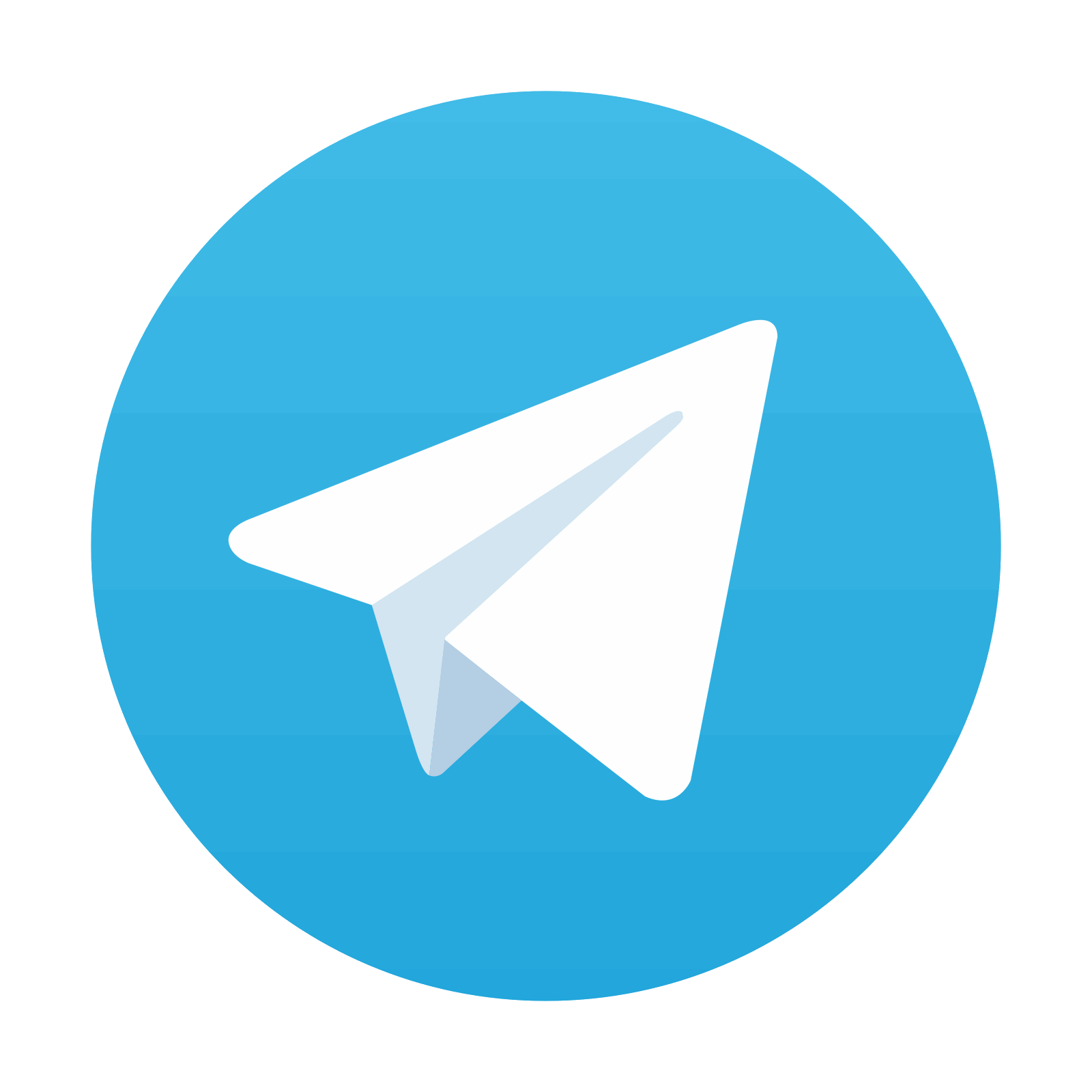
Stay updated, free articles. Join our Telegram channel

Full access? Get Clinical Tree
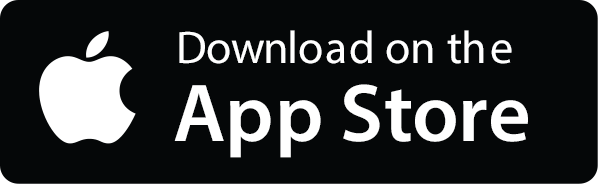
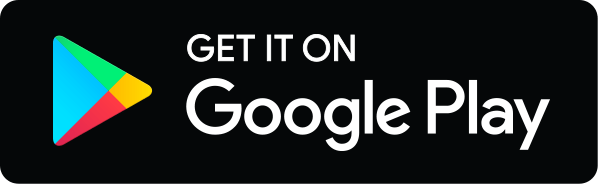
