Potential limitations of this review include the facts that few prospective or randomized trials of vancomycin monitoring were available and that most of the published literature regarding vancomycin monitoring described observational studies in patients with S. aureus infection. Vancomycin monitoring in pediatric patients is beyond the scope of this review.
Overview of Vancomycin Pharmacokinetic and Pharmacodynamic Properties
Sophisticated pharmacokinetic techniques such as Bayesian and noncompartmental modeling have been used to derive pharmacokinetic parameters for vancomycin. The serum vancomycin concentration–time profile is complex and has been characterized as one-, two-, and three-compartment pharmacokinetic models. In patients with normal renal function, the α-distribution phase ranges from 30 minutes to 1 hour, and the β-elimination half-life ranges from 6 to 12 hours. The volume of distribution is 0.4–1 L/kg.14–18
While reports of the degree of vancomycin protein binding have varied, a level of 50–55% is most often stated.19,20 Penetration of vancomycin into tissues is variable and can be affected by inflammation and disease state. For example, with uninflamed meninges, cerebral spinal fluid vancomycin concentrations ranging from 0 to approximately 4 mg/L have been reported, whereas concentrations of 6.4–11.1 mg/L have been reported in the presence of inflammation.21 Penetration into skin tissue is significantly lower for patients with diabetes (median, 0.1 mg/L; range, 0.01–0.45 mg/L) compared with nondiabetic patients based on the median ratio of tissue vancomycin to plasma vancomycin concentrations (median, 0.3 mg/L; range, 0.46–0.94 mg/L).21 Vancomycin concentrations in lung tissue ranging from 5% to 41% of serum vancomycin concentrations have been reported in studies of healthy volunteers and patients.5,6,22,23 Epithelial lining fluid (ELF) penetration in critically injured patients is highly variable, with an overall blood:ELF penetration ratio of 6:1.23,24
Selection of Pharmacokinetic and Pharmacodynamic Monitoring Parameters
A variety of pharmacokinetic and pharmacodynamic monitoring parameters have been proposed for vancomycin, including time (t) the concentration of vancomycin remains above the minimum inhibitory concentration (MIC), the ratio of the area under the serum drug concentration-versus-time curve and the MIC, and the ratio of the maximum serum drug concentration (Cmax) and the MIC. These parameters are abbreviated t>MIC, AUC/MIC, and Cmax/MIC, respectively. Reviews of pharmacokinetics and pharmacodynamics have recommended the AUC/MIC as the preferred parameter based in part on data from animal models, in vitro studies, and limited human studies.6,25–28 Studies by Ackerman et al.,29 Löwdin et al.,30 and Larsson et al.31 demonstrated that vancomycin kills S. aureus and Staphylococcus epidermidis in a concentration-independent fashion. By simulating free vancomycin peak concentrations of 40, 20, 10, and 5 mg/L in an in vitro chemostat model with a normal vancomycin terminal half-life of six hours, Larsson et al.31 found no difference in the corresponding bacterial kill curves for S. aureus.
Using neutropenic mouse models, investigators have concluded that the AUC/MIC is the pharmacodynamically linked parameter for measuring vancomycin’s effectiveness in treating S. aureus, including methicillin-susceptible S. aureus (MSSA), MRSA, and vancomycin-intermediate S. aureus (VISA) strains.6,25 (Note: The total AUC/MIC and the free vancomycin AUC/MIC [AUC × 50% protein binding/MIC] have been interchangeably reported for vancomycin. Unless designated fAUC/MIC, this consensus review refers to total AUC/MIC.)
Craig and Andes32 recently evaluated the use of free vancomycin AUC0–24hr/MIC (fAUC/MIC) as the primary parameter for predicting vancomycin activity against VISA, heteroresistant VISA (hVISA), and MSSA in the murine neutropenic mouse model. They found that the fAUC/MIC requirement varied depending on the vancomycin MIC and was a function of bacterial density at the site of infection, with a lower fAUC/MIC needed for a lower bacterial inoculum. Of interest, the dose required for a 2 log colony-forming unit/g kill was 2.5 times higher for hVISA strains than for VISA and vancomycin-susceptible S. aureus strains. The researchers concluded that vancomycin dosages of 500 mg every 6 hours or 1 g every 12 hours provide fAUC/MIC values of 100–250 and suggested that values around 500 may enhance the therapeutic effectiveness of vancomycin in humans.
Moise-Broder et al.33 explored the use of AUC/MIC in predicting clinical and microbiological success in treating ventilator-associated S. aureus pneumonia. These investigators suggested an average AUC/MIC of 345 for a successful clinical outcome and a ratio of 850 for a successful microbiological outcome. For pathogens with an MIC of 1 mg/L, an AUC/MIC of approximately 250 can be achieved in most patients with 1 g every 12 hours based on a patient with an actual body weight (ABW) of 80 kg and normal renal function (i.e., creatinine clearance [CLcr] = 100 mL/min), but obtaining a target AUC/MIC of 850 would require much higher dosages for most patients.
Summary: Based on these study results, an AUC/MIC ratio of ≥400 has been advocated as a target to achieve clinical effectiveness with vancomycin. Animal studies and limited human data appear to demonstrate that vancomycin is not concentration dependent and that the AUC/MIC is a predictive pharmacokinetic parameter for vancomycin.
Impact of Dosing Strategies on Pharmacokinetic and Pharmacodynamic Parameters
The initial clinical dosing strategies for vancomycin were developed in the late 1950s before the emergence of antibiotic pharmacodynamics.34 Published data on the pharmacodynamics of vancomycin against specific bacterial pathogens or infections are very limited, with much of the available data generated from in vitro or animal models. This is partly due to the drug’s generic status, which discourages manufacturers from conducting well-controlled scientific investigations that would provide additional and clarifying pharmacodynamic data. It is recommended that dosages be calculated based on ABW. There are limited data on dosing in obese patients; however, initial dosages should be based on ABW and adjusted based on serum vancomycin concentrations to achieve therapeutic levels.17
Vancomycin is ideally suited from a pharmacokinetic and pharmacodynamic perspective for intermittent administration based on the usual susceptibility of staphylococci and streptococci (MIC values of ≤1 mg/L), the most commonly used dosage regimen for vancomycin (1 g every 12 hours), and the concentration-independent nature of the drug. As a result, the likelihood of maintaining free or unbound serum vancomycin concentrations in excess of the bacterial MIC for the entire dosing interval is usually 100% with standard intermittent i.v. infusions for typical staphylococci and streptococci.
Despite the absence of clinical data supporting t>MIC as a predictive parameter for clinical effectiveness, continuous-infusion strategies have been suggested as a possible means to optimize the serum vancomycin concentration and improve effectiveness. Using a randomized crossover study design in intensive care unit (ICU) patients, James et al.35 found no significant difference between intermittent and continuous administrations when measuring killing activity in vitro, although the ability to maintain serum bactericidal titers above 1:8 was better with a continuous infusion. In a similarly designed study in healthy subjects, Lacy et al.36 found virtually no difference in activity as measured by bactericidal titers between continuous and intermittent infusions. Further, in a randomized study, Wysocki et al.37,38 evaluated 160 patients with severe staphylococcal infections. No difference in patient outcome was observed between those receiving intermittent or continuous infusion vancomycin. Vancomycin differs from β-lactam antibiotics, which typically have short half-lives and often require shorter dosage intervals or continuous infusion to optimize therapy. Therefore, based on the available evidence, there does not appear to be any difference in patient outcomes between vancomycin administered by continuous infusion or by intermittent administration.
Summary and recommendations: Vancomycin dosages should be calculated on ABW. For obese patients, initial dosing can be based on ABW and then adjusted based on serum vancomycin concentrations to achieve therapeutic levels. Continuous infusion regimens are unlikely to substantially improve patient outcome when compared with intermittent dosing. (Level of evidence = II, grade of recommendation = A.)
Therapeutic Vancomycin Drug Monitoring
Peak versus Trough Concentrations. Over the years, serum vancomycin concentration monitoring practices have varied. Early suggestions, such as those of Geraci,39 who recommended peak serum vancomycin concentrations of 30–40 mg/L and trough concentrations of 5–10 mg/L, likely did not appreciate the multiexponential decline in the serum vancomycin concentration-versus-time curve.
How Geraci defined peak concentration is unclear. In addition, the pharmacodynamic properties of vancomycin had not been evaluated at the time these recommendations were made. Because the AUC/MIC has been found to correlate with efficacy in experiments conducted with in vitro or animal models, this evidence has led some clinicians to question the relevance of monitoring peak serum vancomycin concentrations.6 Consequently, some clinicians have decreased the extent of pharmacokinetic monitoring for this antibiotic.40 However, because it can be difficult in the clinical setting to obtain multiple serum vancomycin concentrations to determine the AUC and subsequently calculate the AUC/MIC, trough serum concentration monitoring, which can be used as a surrogate marker for AUC, is recommended as the most accurate and practical method to monitor vancomycin.
Summary and recommendation: Trough serum vancomycin concentrations are the most accurate and practical method for monitoring vancomycin effectiveness. Trough concentrations should be obtained just before the next dose at steady-state conditions. (Level of evidence = II, grade of recommendation = B.) (Note: Steady-state achievement is variable and dependent on multiple factors. Trough samples should be obtained just before the fourth dose in patients with normal renal function to ensure that target concentrations are attained.)
Optimal Trough Concentrations. While Geraci’s39 recommendation for trough concentration was not based on prospective clinical trial data, the benchmark total drug concentration of 5–10 mg/L is likely to fall short of achieving the desired overall vancomycin exposure in many types of infection and isolates with higher (but susceptible) MICs. Therefore, targeting higher trough serum vancomycin concentrations should increase the likelihood of achieving more effective overall antibiotic exposures (i.e., AUC/MIC) and assist in addressing the trend of higher vancomycin MIC values in these organisms.
In recently published guidelines for hospital-acquired, ventilator-associated, and health-care-associated pneumonia, the American Thoracic Society (ATS) suggested an initial vancomycin dosage of 15 mg/kg every 12 hours in adults with normal renal function.41 ATS acknowledged that vancomycin was a concentration-independent (time-dependent) killer of gram-positive pathogens but had lower penetration into the ELF and respiratory secretions. ATS further recommended that trough serum vancomycin concentrations be maintained at 15–20 mg/L. However, based on pharmacokinetic dosing principles for patients with a normal body weight and normal renal function, it is unlikely that vancomycin 15 mg/kg every 12 hours will produce trough concentrations of 15–20 mg/L. Furthermore, there are no data indicating that achieving these trough concentrations over time is well tolerated and safe.
In an attempt to evaluate the use of targeted trough concentrations of 15–20 mg/L, Jeffres et al.42 retrospectively evaluated 102 patients with health-care-associated MRSA pneumonia. Overall mortality was 31% (32 patients). There were no significant differences in mean ± S.D. calculated trough serum vancomycin concentrations (13.6 ± 5.9 mg/L versus 13.9 ± 6.7 mg/L) or mean ± S.D. calculated AUC (351 ± 143 mg · hr/L versus 354 ± 109 mg · hr/L) between survivors and nonsurvivors. In addition, no relationship was found between trough serum vancomycin concentrations or AUC and hospital mortality. Although no significant differences were found between survivors and nonsurvivors in terms of trough serum vancomycin concentrations and AUCs, several factors should be noted. For instance, a sample size calculation was not predetermined; therefore, the potential for a Type II error is possible. There was also large variability in both vancomycin trough concentrations (range, 4.2–29.8 mg/L) and AUCs (range, 119–897 mg · hr/L), which may account for the lack of significant findings. Time to achieve targeted serum vancomycin concentrations was not measured and may be a critical factor in determining patient outcome. In addition, because a disk-diffusion method was used for susceptibility testing, organism MIC could not be determined. Therefore, only the AUC, not the AUC/MIC, was evaluated as a potential predictor of success or failure. Although the results of this study are of interest, additional prospective studies are needed to confirm these data.
Relationship between trough vancomycin concentrations, resistance, and therapeutic failure. While vancomycin is considered a bactericidal antibiotic, the rate of bacterial kill is slow when compared with that of β-lactams, and vancomycin’s activity is affected by the bacterial inoculum. Large bacterial burdens in the stationary growth phase or in an anaerobic environment pose a significant challenge to the speed and extent of vancomycin’s bactericidal activity.43–46
In recent years, VISA or glycopeptide-intermediate susceptible S. aureus (GISA) and vancomycin-resistant S. aureus (VRSA) have appeared and raised questions about the overall utility of this antibiotic. (Note: The terms VISA and GISA are often used interchangeably. For the purpose of this consensus review, VISA will be used throughout.) Although infection with these organisms is infrequent, there is fear that the organisms could become more prevalent if the high rate of use and exposure pressure of vancomycin continues.47 The discovery of inducible hVISA (i.e., strains with MIC values in the susceptible range of 0.5–2 mg/L in patients whose therapy with standard dosages of vancomycin has failed) raises further questions regarding current dosing guidelines and the overall use of this antibiotic. Concerns are related to treatment failures and the inability to easily detect hVISA isolates in clinical settings.48–50
In 2006, the Clinical and Laboratory Standards Institute (CLSI) lowered the susceptibility and resistance breakpoints for the MIC of vancomycin from ≤4 to ≤2 mg/L for “susceptible,” from 8–16 to 4–8 mg/L for “intermediate,” and from ≥32 to ≥16 mg/L for “resistant.”51 The decision to move the breakpoints was primarily based on clinical data indicating that patients were less likely to be successfully treated with vancomycin if the S. aureus MIC was ≤4 mg/L.51 Despite the change in susceptibility and resistance breakpoints, two reports have suggested that patients with S. aureus isolates having vancomycin MICs of 1–2 mg/L are less likely to be successfully treated with vancomycin compared with patients with S. aureus isolates that demonstrate greater susceptibility.52,53 However, this information alone does not address whether the use of higher concentrations of vancomycin would improve overall effectiveness. Low serum vancomycin concentrations may also create problems, as there appears to be a direct correlation between low serum vancomycin levels and the emergence of hVISA, VISA, or both, at least with certain genotypes of MRSA.54 In addition, studies have suggested that trough serum vancomycin concentrations of <10 mg/L may predict therapeutic failure and the potential for the emergence of VISA or VRSA.54,55
Studies of MRSA and hVISA bacteremia have revealed significantly higher rates of morbidity in patients infected with hVISA.50,55,56 These patients were more likely to have high bacterial load infections, low initial trough serum vancomycin concentrations, and treatment failure.56 Jones57 recently reported that approximately 74% of hVISA strains and 15% of wild-type S. aureus strains were tolerant (minimum bactericidal concentration of ≥32 mg/L) to the effects of vancomycin, which contributes to a low probability of success in patients harboring these organisms.
Sakoulas et al.52 reported a significant correlation between vancomycin susceptibilities and patient outcome. Treatment of bloodstream infections caused by MRSA strains having a vancomycin MIC of ≤0.5 mg/L had an overall success rate of 55.6%, while treatment of patients infected with MRSA strains having a vancomycin MIC of 1–2 mg/L had a success rate of only 9.5% (p = 0.03). (Treatment failure was defined as persistent signs or symptoms of infection [e.g., fever, leukocytosis], new signs or symptoms of infection, or worsening of signs or symptoms of infection in patients receiving at least five days of therapy with targeted trough serum vancomycin concentrations of 10–15 mg/L). However, this was a relatively small study (n = 30) of MRSA bacteremic patients who were refractory to vancomycin therapy and were enrolled in compassionate-use drug trials. In a more recent study of patients with MRSA bacteremia (n = 34), Moise et al.58 demonstrated that patients with MRSA isolates with a vancomycin MIC of 2 mg/L had significantly higher median days to organism eradication, longer treatment with vancomycin, and a significantly lower overall likelihood of organism eradication. Hidayat et al.53 evaluated the use of high-dosage vancomycin intended to achieve unbound trough serum vancomycin concentrations of at least four times the MIC in patients with MRSA infections. Of the 95 patients evaluated with MRSA pneumonia or bacteremia, or both, 51 (54%) had vancomycin MIC values of 1.5 or 2 mg/L. Although an initial response of 74% was demonstrated in patients achieving the desired target MIC, a high percentage of patients infected with strains having an MIC of 1.5 or 2 mg/L had a poorer response (62% versus 85%) and significantly higher infection-related mortality (24% versus 10%) compared with patients infected with low-MIC strains (0.5, 0.75, or 1 mg/L), despite achieving target trough serum vancomycin concentrations of 15–20 mg/L. The data from these two studies suggest that S. aureus isolates with MICs of 1–2 mg/L that are still within the susceptible range may be less responsive to vancomycin therapy. Soriano et al.59 evaluated the influence of vancomycin MIC on outcome in a total of 414 episodes of MRSA bacteremic patients. MIC evaluations were determined by Etest methodology. Among several factors that predicted poor outcome, S. aureus isolates with an MIC of 2 mg/L were significantly associated with increased mortality. Based on the low probability of achieving an appropriate targeted vancomycin concentration exposure (AUC/MIC), the authors suggested that vancomycin should not be considered an optimal treatment approach for infection due to strains with a vancomycin MIC of >1 mg/L when using trough serum vancomycin concentrations of >10 mg/L as a target.
Lodise et al.60 evaluated the relationship between vancomycin MIC and treatment failure among 92 adult nonneutropenic patients with MRSA bloodstream infections. Vancomycin failure was defined as 30-day mortality, 10 or more days of bacteremia on vancomycin therapy, or recurrence of MRSA bacteremia within 60 days of vancomycin discontinuation. Classification and regression tree analysis found that a vancomycin MIC breakpoint of ≥1.5 mg/L was associated with an increased probability of treatment failure. The 66 patients with a vancomycin MIC of ≥1.5 mg/L had a 2.4-fold higher rate of treatment failure compared with patients with a vancomycin MIC of ≤1 mg/L (36.4% versus 15.4%, respectively; p = 0.049). Poisson regression analysis determined that a vancomycin MIC of ≥1.5 mg/L was independently associated with treatment failure (p = 0.01). Based on these findings, the investigators suggested that an alternative therapy should be considered.
An analysis of a large surveillance database of 35,458 S. aureus strains by Jones57 found that the MIC required to inhibit the growth of 50% of organisms or the MIC required to inhibit the growth of 90% of organisms (MIC90) for vancomycin is 1 mg/L.57 The Centers for Disease Control and Prevention 2005 U.S. Surveillance Network data of vancomycin susceptibility reported that 16.2% of 241,605 S. aureus isolates had an MIC of 2 mg/L.51 Regional variability exists, and an MIC90 of 2 mg/L has recently been reported by several institutions. For example, Mohr and Murray61 reported that as many as 30% of 116 MRSA blood culture isolates collected from the Texas Medical Center over a one-year period had a vancomycin MIC of 2 mg/L. There have been recent reports of significant shifts in bacterial susceptibility to vancomycin over a five-year surveillance period.62–64 Increasing S. aureus MIC values, coupled with reports of failure rates associated with a vancomycin MIC of 2 mg/L, have raised the question of whether the breakpoint for vancomycin resistance should be lowered even further.65
New information is emerging regarding the importance of the accessory gene regulator (agr), a global quorum-sensing regulator in S. aureus that is responsible for orchestrating the expression of adherence factors, biofilm production, tolerance to vancomycin, and virulence factors.66 The agr locus has been a subject of intense study because there appears to be a relationship between polymorphism in this gene cluster and patient response to vancomycin therapy. Several studies have determined that all VISA strains reported to date from the United States belong to agr group II. The agr group II includes the USA 100 MRSA clones that are predominately associated with nosocomial infections, and these strains have been associated with vancomycin treatment failure.33,67 Sakoulas et al.67,68 have determined in in vitro studies that the emergence of hVISA or VISA may occur when S. aureus isolates with a down-regulated or defective agr locus are exposed to suboptimal vancomycin concentrations. In a series of in vitro experiments, MRSA belonging to agr group II with a defective agr locus exposed to vancomycin concentrations of <10 mg/L produced heteroresistant-like characteristics similar to VISA strains with subsequent MIC increases from 1 to 8 mg/L.67 This phenomenon was recently demonstrated in a patient with chronic renal failure undergoing hemodialysis who experienced recurrent MRSA bacteremia over a 30-month period.54 The patient was treated repeatedly with vancomycin at trough serum concentrations that always exceeded 10 mg/L. Despite frequent recurrences of bacteremia with the same isolate, the isolate remained susceptible to vancomycin. The genetic background of this organism was found to be similar to other VISA strains belonging to agr group II. When the isolate was subjected to vancomycin concentrations of <10 mg/L under laboratory conditions, it quickly demonstrated characteristics similar to VISA strains with a subsequent increased MIC.
Tsuji et al.69 used an in vitro pharmacodynamic model to evaluate S. aureus agr groups I–IV exposed to optimal and suboptimal vancomycin doses over a three-day period. In this study, low vancomycin exposures equivalent to total trough serum vancomycin concentrations of 1.5–10 mg/L and an AUC/MIC of 31–264 produced increases in the MIC to the range considered to be VISA by the current CLSI vancomycin breakpoints. Although resistance was produced in both agr functional and defective strains, the likelihood of resistance was fourfold to fivefold higher in agr-defective isolates. Subsequently, the investigators determined that as many as 48% of hospital-associated MRSA had a dysfunctional agr locus, making this finding potentially clinically relevant and warranting further evaluation.70
Summary and recommendation: Based on evidence suggesting that S. aureus exposure to trough serum vancomycin concentrations of <10 mg/L can produce strains with VISA-like characteristics, it is recommended that trough serum vancomycin concentrations always be maintained above 10 mg/L to avoid development of resistance. (Level of evidence = III, grade of recommendation = B.)
Correlating dosing with optimal AUC/MIC and trough concentrations. As mentioned previously, an isolate’s vancomycin MIC is an important parameter for determining the potential success of a given dosage regimen. Therefore, an actual vancomycin MIC value should ideally be obtained from the clinical microbiology laboratory. Currently, some clinical microbiology laboratories may be limited in their ability to report vancomycin MIC values, depending on the methodology (disk diffusion or automated microdilution) used to determine antimicrobial susceptibility. In some instances, supplemental Etest methods may be used to obtain this information.
As previously stated, an AUC/MIC of ≥400 has been promoted as the target predictive of successful therapy (i.e., organism eradication). Based on this information, a simple evaluation of standard dosing practices (e.g., 1 g every 12 hours) for an individual with normal renal function (CLcr of ≥100 mL/min) and average weight (80 kg) would only yield a 24-hour drug AUC of approximately 250 mg · hr/L. Unless the pathogen had a vancomycin MIC of ≤0.5 mg/L, this dosage regimen would not generate the targeted AUC/MIC of ≥400. For a pathogen with an MIC of 1 mg/L, the minimum trough serum vancomycin concentration would have to be at least 15 mg/L to obtain the target AUC/MIC. Using the vancomycin pharmacokinetic data generated by Jeffres et al.42 in patients receiving vancomycin for the treatment of MRSA pneumonia, Mohr and Murray61 determined by Monte Carlo simulation that the probability of achieving an AUC/MIC of ≥400 would be 100% if the S. aureus MIC for vancomycin was 0.5 mg/L but 0% if the MIC was 2 mg/L. Using a similar one-compartment model of vancomycin and a Monte Carlo simulation integrating S. aureus MIC values, del Mar Fernández de Gatta Garcia et al.71 reported that a daily dosage of 3–4 g of vancomycin would be required to provide 90% probability of attaining a target AUC/MIC of 400 with an MIC of 1 mg/L. For VISA strains, a vancomycin daily dose of ≥5 g would be required to provide a high probability of target AUC/MIC attainment for this pathogen. For susceptible S. aureus, total daily doses of ≥40 mg/kg would likely be required for typical patients. Use of these larger dosages of vancomycin should be carefully monitored for the desired clinical outcome and the absence of drug-induced toxicity. The use of a nomogram is an alternative method for dosage adjustments; however, the majority of published nomograms in clinical use have been proven to be inaccurate, and most have not been clinically validated.72 In addition, no published nomogram to date has been constructed to achieve trough serum vancomycin concentrations of 15–20 mg/L.
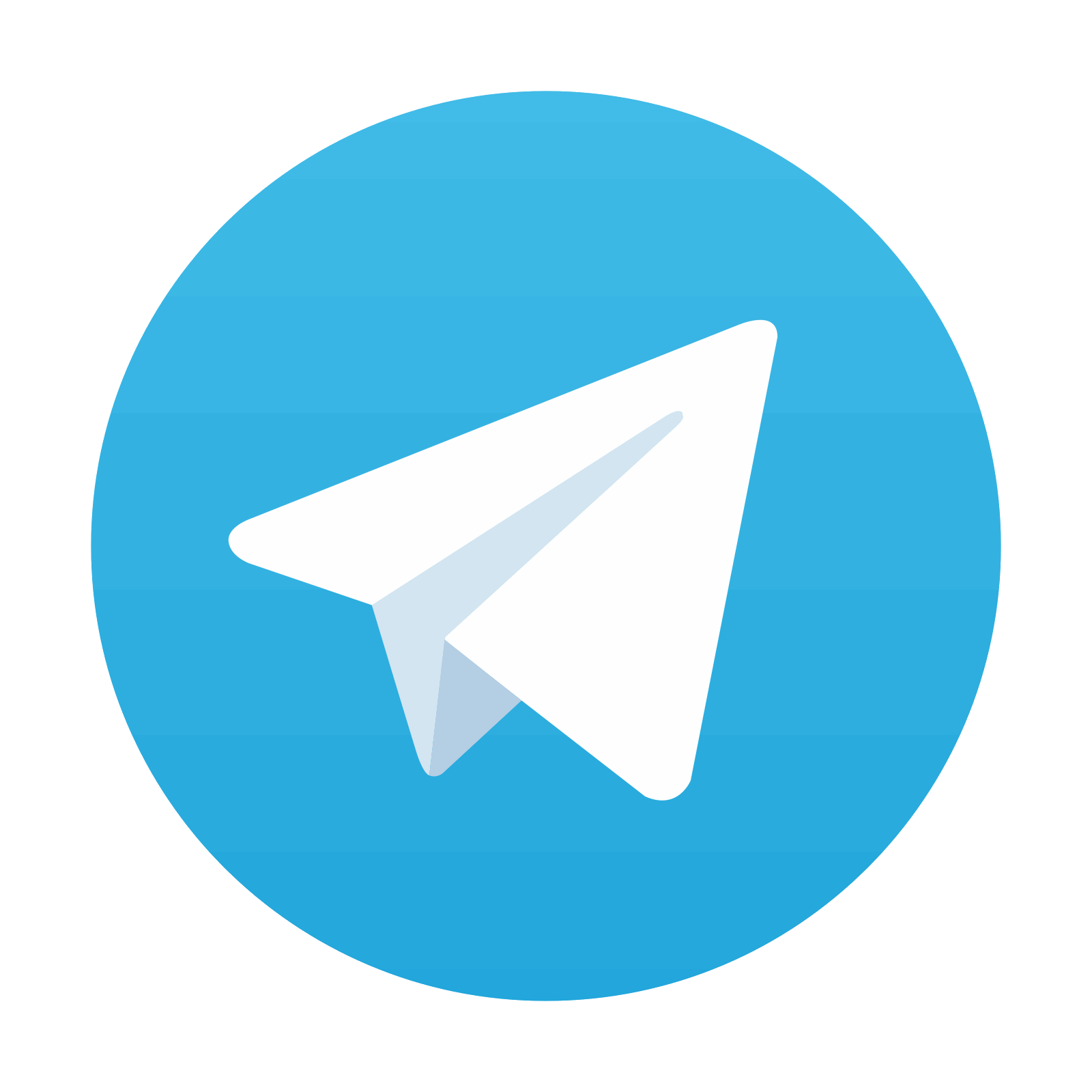
Stay updated, free articles. Join our Telegram channel

Full access? Get Clinical Tree
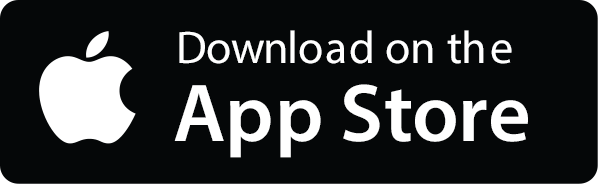
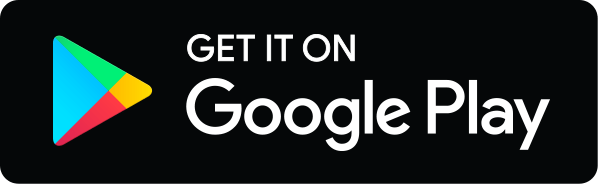