Techniques
Marker type
Require prior molecular information
Mode of inheritance
Degree of polymorphism
Major applications
Discoverer
Non PCR-based techniques
Restriction fragment length polymorphism
Yes
Mendelian, codominant
Low
Linkage mapping, Physical mapping
Bostein et al. (1980)
PCR-based techniques
Random amplified polymorphic DNA
No
Mendelian, dominant
Intermediate
Fingerprinting for population study, Gene tagging, Hybrid identification
Williams et al. (1990)
Amplified fragment length polymorphism
No
Mendelian, dominant
High
Linkage mapping, Gene tagging, population study
Vos et al. (1995)
Microsatellite, SSR
Yes
Mendelian, codominant
High
Linkage mapping, population study, Genetic diversity, Paternity analysis
Litt and Lutty (1989)
Minisatellite, VNTR
No
Mendelian, codominant
High
DNA fingerprinting for population study
Jeffrey (1985)
Single nucleotide polymorphism
Yes
Mendelian, codominant
High
Linkage mapping, population study
Ching et al. (2002)
An ideal molecular marker technique should (1) be polymorphic and evenly distributed throughout the genome, (2) provide adequate resolution of genetic differences, (3) generate multiple, independent and reliable markers, (4) be simple, quick, and inexpensive, (5) need small amounts of tissue and DNA samples (6) have linkage to distinct phenotypes and (7) require no prior information about the genome of an organism.
3.2 Molecular Marker Techniques
A vast array of DNA-based genetic markers for the detection of DNA polymorphism have been discovered since 1980 and new marker types are developed every year. There are several DNA marker types, which may be classified broadly into two groups based on their detection method, (1) Non PCR based or those based on DNA–DNA hybridization and (2) PCR based or those based on amplification of DNA sequences using the polymerase chain reaction (PCR) (Table 3.1).
3.2.1 Non PCR Based or DNA–DNA Hybridization
3.2.1.1 Restriction Fragment Length Polymorphism
Restriction fragment length polymorphism markers were the first DNA-based genetic markers developed (Botstein et al. 1980). Eukaryotic genomes are very large and there was no simple way to observe genetic polymorphisms of individual genes or sequences. The property of complementary base pairing where small piece of DNA could be used as probe allowed for methods to be developed to reveal polymorphisms in sequences homologous to the probe. The genetic system derived using this approach is called restriction fragment length polymorphism. RFLP (commonly pronounced “rif-lip”) refers to a difference between two or more samples of homologous DNA molecules arising from differing locations of restriction sites. If two organisms differ in the distance between sites of cleavage of particular Restriction endonucleases, the length of the fragments produced will differ when the DNA is digested with a restriction enzyme. The dissimilarity of the patterns generated can be used to differentiate species (and even strains) from one another. In RFLP analysis, the DNA sample broken into pieces (digested) by restriction enzymes that are separated according to their lengths on agarose gel electrophoresis . It is possible to visualize DNA within such a gel by staining it with ethidium bromide, however, due to typically so many restriction fragments of all possible sizes, discrete fragments cannot be seen. To overcome this problem, the fractionated DNA is transferred and chemically bound to a nylon membrane by a process called Southern blotting, named after its inventor E. M. Southern (1975). Specific DNA fragments are visualized by hybridizing the DNA fragments bound to the nylon membrane with a radioactively or fluorescently labeled DNA probe. Different sizes or lengths of restriction fragments are typically produced when different individuals are tested. Such a polymorphism can be used to distinguish plant species, genotypes and, in some cases, individual plants. Labeling of the probe may be performed with a radioactive isotope or with alternative non-radioactive stains, such as digoxigenin or fluorescein. These probes are mostly species-specific single locus probes of about 0.5–3.0 kb in size, obtained from a cDNA library or a genomic library. Though genomic library probes may exhibit greater variability than probes from cDNA libraries, a few studies reveal the converse (Miller and Tanksley 1990). In addition to genetic fingerprinting, RFLP is an important tool in genome mapping, localization of genes for genetic disorders, and determination of risk for disease and paternity testing.
Advantages: The RFLP markers are relatively highly polymorphic, codominantly inherited, and highly reproducible. Because of their presence throughout the eukaryotic genome, high heritability and locus specificity of the RFLP markers are considered superior. The method also provides opportunity to simultaneously screen numerous samples. DNA blots can be analyzed repeatedly by stripping and reprobing (usually 8 to 10 times) with different RFLP probes.
Disadvantages: The technique is not very widely used because it is time consuming, expensive, and only one out of several markers may be polymorphic, which is highly inconvenient especially for crosses between closely related species. The utility of RFLPs has been hampered due to the large quantities of purified, high molecular weight genomic DNA requirement for DNA digestion as well as Southern blotting. The use of radioactive isotope makes the analysis relatively expensive and hazardous. The requirement of prior sequence information for probe generation increases the complexity of the methodology. Their inability to detect single base changes restricts their use in detecting point mutations occurring within the regions at which they are detecting polymorphism.
Applications: Restriction Fragment length polymorphism is a powerful tool for the identification of organisms to the level of species, strains, varieties, or individuals. RFLPs have been widely used in population genetics to determine slight differences within populations, and in linkage studies to generate maps for quantitative trait locus (QTL) analysis. They also have been used to investigate relationships of closely related taxa or intraspecific level, as fingerprinting tools for diversity studies, and for studies of hybridization. They have been used in criminal and paternity tests, localization of genes for genetic disorders, and determination of risk for disease.
3.2.2 PCR-Based Marker
After the invention of polymerase chain reaction (PCR) technology (Mullis and Faloona 1987), a large number of approaches for generation of molecular markers based on PCR were detailed, primarily due to its apparent simplicity and high probability of success. Before PCR, the analysis of a specific DNA fragment generally required cloning of the fragment and amplification in a plasmid or compatible vector. PCR enables the production of a large amount of specific DNA sequence without cloning, starting with just a few molecules of the target sequence. Usage of random primers overcame the limitation of prior sequence knowledge for PCR analysis and facilitated the development of genetic markers for a variety of purposes.
3.2.2.1 Amplified Fragment Length Polymorphism
Amplified fragment length polymorphism PCR (or AFLP-PCR or just AFLP) is a PCR-based tool used in genetics research, DNA fingerprinting and in the practice of genetic engineering. Developed by Vos et al. (1995), AFLP is essentially an intermediate between RFLP and PCR. AFLP selectively amplifies a subset of restriction fragments from a complex mixture of DNA fragments obtained after digestion of genomic DNA with restriction endonucleases. Polymorphisms are detected from differences in the length of the amplified fragments by polyacrylamide gel electrophoresis (PAGE) or by capillary electrophoresis . AFLP uses restriction enzymes to cut genomic DNA, followed by ligation of adaptors to the sticky ends of the restriction fragments. A subset of the restriction fragments are then amplified using primers complementary to the adaptor and part of the restriction site fragments. The amplified fragments are visualized on denaturing polyacrylamide gels either through autoradiography or fluorescence methodologies (Vos et al. 1995). AFLPs are DNA fragments (80–500 bp) obtained from digestion with restriction enzymes, followed by ligation of oligonucleotide adapters to the digestion products and selective amplification by the PCR. AFLPs, therefore, involve both RFLP and PCR. The AFLP banding profiles are the result of variations in the restriction sites or in the intervening region. The AFLP technique simultaneously generates fragments from many genomic sites (usually 50–100 fragments per reaction) that are separated by polyacrylamide gel electrophoresis and that are generally scored as dominant markers.
Advantages: There are many advantages of AFLP, they are produced in greater amount and have higher reproducibility, resolution, and sensitivity at the whole genome level and also has the capability to amplify between 50 and 100 fragments at one time. In addition, no prior sequence information is needed for amplification. As a result, AFLP has become extremely beneficial in the study of taxa including bacteria, fungi, and plants, where much is still unknown about the genomic makeup of various organisms. AFLPs can be analyzed on automatic sequencers, but software problems concerning the scoring of AFLPs are encountered on some systems. The use of AFLP in genetic marker technologies has become the main tool due to its capability to disclose a high number of polymorphic markers by single reaction (Vos et al. 1995).
Disadvantages: Disadvantages of this technique are that alleles are not easily recognized, have medium reproducibility, labor intensive, and have high operational and developmental costs. AFLP needs purified, high molecular weight DNA, the dominance of alleles and the possible non-homology of co-migrating fragments belonging to different loci.
Applications: Most AFLP fragments correspond to unique positions on the genome and have the capability to detect various polymorphisms in different genomic regions simultaneously and hence can be exploited as landmarks in genetic and physical mapping. The technique can be used to distinguish closely related individuals at the subspecies level (Althoff et al. 2007) and also for gene mapping. Applications for AFLP in plant mapping include establishing linkage groups in crosses, saturating regions with markers for gene landing efforts (Yin et al. 1999), and assessing the degree of relatedness or variability among cultivars (Mian et al. 2002). Molecular markers are more reliable for genetic studies than morphological characteristics, because the environment does not affect them. AFLP is considered more applicable to intraspecific than to interspecific studies due to frequent null alleles. AFLP markers are useful in genetic studies, such as biodiversity evaluation, analysis of germplasm collections, genotyping of individuals, and genetic distance analysis. The availability of many different restriction enzymes and corresponding primer combinations provides a great deal of flexibility, enabling the direct manipulation of AFLP fragment generation for defined applications (e.g., polymorphism screening, QTL analysis, and genetic mapping).
3.2.2.2 Random Amplified Polymorphic DNA
The basis of randomly amplified polymorphic DNA technique is differential PCR amplification of genomic DNA. It deduces DNA polymorphisms produced by “rearrangements or deletions at or between oligonucleotide primer binding sites in the genome” using short random oligonucleotide sequences (mostly 10 bases long) (Williams et al. 1990). As the approach requires no prior knowledge of the genome that is being analyzed, it can be employed across species using universal primers. In the RAPD marker system, a PCR reaction is conducted using a very small amount of template DNA (even less than 10 ng is sufficient) and a single RAPD primer. Primers are usually just 10 base pairs long (10 mers) and are of random sequence. The analysis of RAPD is based on the PCR using short (about 10 bases) randomly chosen primers singly which anneal as reverted repeats to the complementary sites in the genome. The DNA between the two opposite sites with the primers as starting and end points is amplified by PCR. The amplification products are separated on agarose gels in the presence of ethidium bromide and viewed under ultraviolet light. The banding patterns distinguish organisms according to the presence or absence of bands (polymorphism). These polymorphisms are considered to be primarily due to variation in the primer annealing sites, but they can also be generated by length differences in the amplified sequence between primer annealing sites. Each product is derived from a region of the genome that contains two short segments in inverted orientation, on opposite strands that are complementary to the primer. It is a peculiarity of RAPD analysis that it discriminates at different taxonomical level, viz., isolates and species, depending on the organism investigated and the primer used
Advantages: The main advantage of RAPDs is that they are quick and easy to assay. Because PCR is involved, only low quantities of template DNA are required, usually 5–50 ng per reaction. Since random primers are commercially available, no sequence data for primer construction are needed. Moreover, RAPDs have a very high genomic abundance and are randomly distributed throughout the genome. They are dominant markers; hence have limitations in their use as markers for mapping, which can be overcome to some extent by selecting those markers that are linked in coupling (Williams et al. 1993). RAPD assay has been used by several groups as efficient tools for identification of markers linked to agronomically important traits, which are introgressed during the development of near isogenic lines. The RAPD analysis of NILs (nonisogenic lines) has been successful in identifying markers linked to disease resistance genes in tomato (Lycopersicon sp.), lettuce (Lactuca sp.), and common bean (Phaseolus vulgaris). Due to the speed and efficiency of RAPD analysis, high-density genetic mapping in many plant species was developed in a relatively short time.
Disadvantages: The major drawback of the method is that the profiling is dependent on the reaction conditions so it may vary within two different laboratories and as several discrete loci in the genome are amplified by each primer, profiles are not able to distinguish heterozygous from homozygous individuals (Bardakci 2001).
Applications: The application of RAPDs and their related modified markers in variability analysis and individual-specific genotyping has largely been carried out, but is less popular due to problems such as poor reproducibility, faint or fuzzy products, and difficulty in scoring bands, which lead to inappropriate inferences. RAPDs have been used for many purposes, ranging from studies at the individual level (e.g., genetic identity) to studies involving closely related species and determination of genetic diversity. RAPDs have also been applied in gene mapping studies to fill gaps not covered by other markers.
3.2.2.3 Sequence Characterized Amplified Region
Sequence characterized amplified regions (SCARs) markers were developed by Michelmore et al. (1991) and Martin et al. (1991). SCARs are DNA fragments amplified by the use of PCR using specific 20–30 bp primers. The primers are designed from terminal ends of a RAPD marker. RAPD marker fragments associated with a phenotypic condition of interest are cloned and sequenced. This nucleotide sequence is then used for designing of unique primers for specific amplification of a particular locus. Use of unique primers decreases site competition for specific regions among primers, making the results less sensitive to reaction conditions. Use of longer primers also increases the reproducibility of the results by increasing specificity of template binding by the primer. PCR products obtained after amplification are analyzed for the presence of length polymorphism by gel electrophoresis. Conversion of RAPDs into SCARs may have additional advantage of obtaining a codominant marker, although dominance may be exhibited by SCARs when one or both primers partially overlap the site of sequence variation.
Advantages: Due to the application of PCR, low amounts of genomic or template DNA is required (10–50 ng per reaction) for analysis of the marker. The major advantage of SCARs is the ease of their use, and requirement of relatively less time for the analysis. SCAR markers are locus specific and the results obtained from analysis of SCARs are highly reproducible.
Disadvantages: Major limitation lies in the fact that analysis of SCARs can be done only for organism, species, or DNA fragment with known sequence; as knowledge of sequence data is required for designing of the PCR primers.
Applications: Major application of SCAR makers has been found in gene mapping studies as they are locus specific. They are also used in marker assisted selection, where presence of a specific genotype and expression of its corresponding phenotype can be correlated to the presence or absence of a SCAR marker (Paran and Michelmore 1993).
3.2.2.4 Minisatellites, Variable Number of Tandem Repeats
The term ‘minisatellite’ was introduced by Jeffrey et al. (1985). These loci contain tandem repeats that vary in the number of repeat units between genotypes and are referred to as variable number of tandem repeats (VNTRs) (i.e., a single locus that contains variable number of tandem repeats between individuals) or hypervariable regions (HVRs) (i.e., numerous loci containing tandem repeats within a genome generating high levels of polymorphism between individuals). Minisatellites are a conceptually very different class of marker. They consist of chromosomal regions containing tandem repeat units of a 10–50 base motif, flanked by conserved DNA restriction sites. A minisatellite profile consisting of many bands, usually within a 4–20 kb size range, is generated by using common multi locus probes that are able to hybridize to minisatellite sequences in different species. Locus specific probes can be developed by molecular cloning of DNA restriction fragments, subsequent screening with a multi locus minisatellite probe and isolation of specific fragments. Variation in the number of repeat units, due to unequal crossing over or gene conversion, is considered to be the main cause of length polymorphisms. Due to the high mutation rate of minisatellites, the level of polymorphism is substantial, generally resulting in unique multilocus profiles for different individuals within a population.
Advantages: The main advantages of minisatellites are their high level of polymorphism and high reproducibility.
Disadvantages: Disadvantages of minisatellites are similar to RFLPs due to the high similarity in methodological procedures. If multilocus probes are used, highly informative profiles are generally observed due to the generation of many informative bands per reaction. In that case, band profiles cannot be interpreted in terms of loci and alleles and similar sized fragments may be nonhomologous. In addition, the random distribution of minisatellites across the genome has been questioned (Schlötterer 2004).
Applications: The term DNA fingerprinting was introduced for minisatellites, though DNA fingerprinting is now used in a more general way to refer to a DNA-based assay to uniquely identify individuals. Minisatellites are particularly useful in studies involving genetic identity, parentage, clonal growth and structure, and identification of varieties and cultivars and for population-level studies. Minisatellites are of reduced value for taxonomic studies because of hyper variability.
3.2.2.5 Simple Sequence Repeat or Microsatellites
The simple sequence repeats (SSR) are also referred to as microsatellites. ‘Microsatellites’, coined by Litt and Lutty (1989), consist of tandemly repeating units of DNA ubiquitous in prokaryotes and eukaryotes, scattered throughout most eukaryotic genomes (Powell et al. 1996). Microsatellites can comprise repetition of mono-, di-, tri-, tetra- or penta-nucleotide units. The variation in number of repeat units of a microsatellite resulting in length polymorphisms is mainly attributed to Polymerase slippage during DNA replication, or slipped strand mispairing. Function of such microsatellites is largely unknown, though they can occur in protein-coding as well as noncoding regions of the genome. Microsatellite sequences are especially exploited to differentiate closely related species or genotypes. Due to the high degree of variability present in microsatellites, they are preferred in population studies (Smith and Devey 1994) and to distinguish closely related plant cultivars.
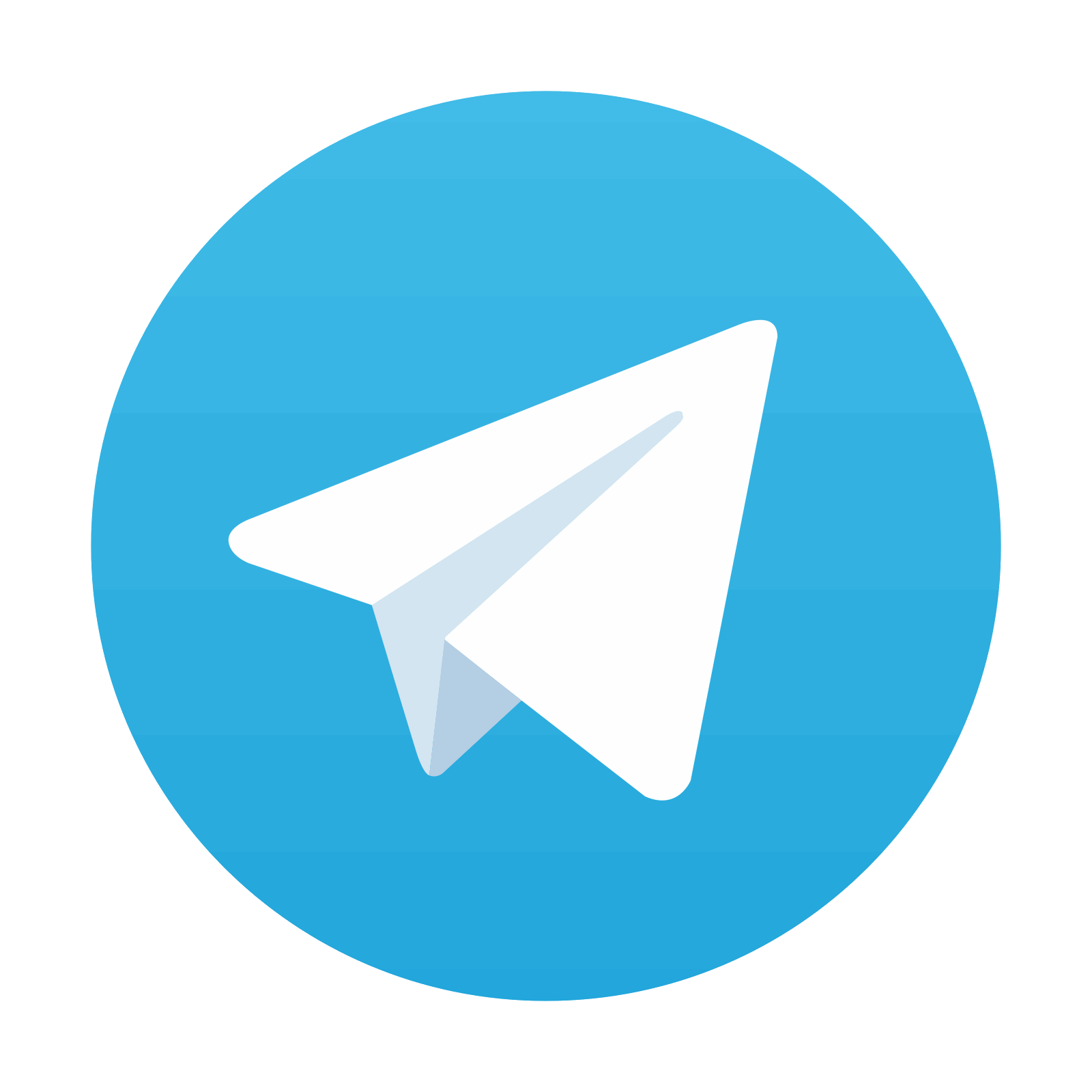
Stay updated, free articles. Join our Telegram channel

Full access? Get Clinical Tree
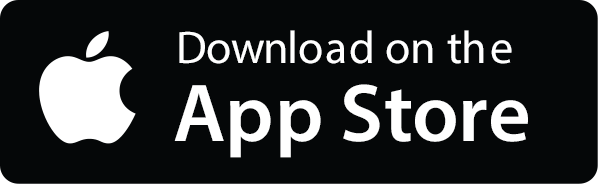
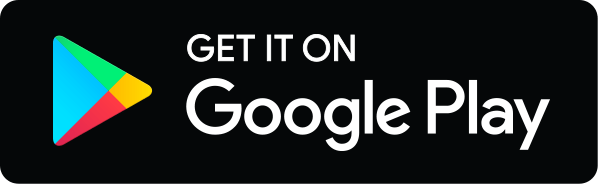