1.
Corneal preservation media
2.
Tattooing solution
3.
Acid and alkali burn
4.
Vitreolytic agents and replacement agent
5.
Ocular use of immunosuppressants
6.
Local anesthetics
7.
Contact lens solution
8.
Drug preservatives used in ophthalmic solution
9.
Irrigating solutions
17.2 Corneal Preservation Media
Santosh Patnaik4
(4)
Ocular Pharmacology and Pharmacy, Dr. Rajendra Prasad Center for Ophthalmic Sciences, All India Institute of Medical Sciences, New Delhi, India
Corneal blindness is the second most common preventable blindness in the world after cataract. In developing countries like India, out of 1% total corneal blind cases, half can be corrected by corneal transplant. Vitamin A deficiency, infections, trauma, improper use of contact lenses and inappropriate use of home remedies are the common causes of preventable blindness (Acharya et al. 2012). Worldwide approximately 45 million people are suffering from bilateral blindness and 135 million have severe bilateral vision impairment (Whitcher et al. 2001). As stated above, corneal transplant is a potential mean to correct treatable blindness. For the successful achievement of the transplant, a good cornea is of paramount importance. Previously corneas were stored in the moist chamber maintained at 4 °C. This technique was having a drawback of the endothelial insult due to the presence of the metabolic waste and other materials in aqueous humor. Corneal preservation witnessed a major breakthrough in 1965 when Capella, Kaufman and Robbins developed the technique of corneal tissue cryopreservation. This technique could able to maintain corneas for more than 1 year in transplantable state. Still the technique was suffering from few shortcomings like requirement of special equipment, trained technician and donor tissue removal in less than 6 h postmortem. In 1968, stocker developed a method to store the corneas in the homologous serum. This technique led him to transplant corneas stored for more than 4 days. This technique had a specific requirement of the serum of acceptor of the cornea (McCarey and Kaufman 1974). In 1968, Mizukawa and Manabe developed a preservation solution in which whole excised eyeball could be submerged. The medium was formulated using chondroitin sulfate, inosine, adenine, adenosine, penicillin and streptomycin. Almost 600 successful keratoplasties were done using this technique (Mizukawa and Manabe 1968). This revolutionary work became the basis for the development of future chondroitin sulfate-containing media. In March 1974, two scientists, Bernard E. McCarey and Herbert E. Kaufman, developed a storage medium which affected the storage of corneas in a greater way. The most important factor involved with this media was the non-requirement of the specialized techniques stated earlier. This modified tissue culture medium was given the name MK medium by them. MK medium is a mixture of medium 199 (containing cell nutrients, commercially made by Sigma Aldrich, US) and dextran with antibiotics. It is a buffered medium which has an osmolarity between 290 and 320 and a pH 7.4. After the development of medium, it was tested on the rabbit corneas where it is found to maintain them for 14 days (McCarey and Kaufman 1974). In 1984, intermediate storage medium was introduced by Kaufman and associates. Medium named K-sol contains 2.5 % chondroitin sulfate, modified medium 199 with HEPES. The medium contains gentamicin for the maintenance of the sterility. It was proposed to store and maintain them for 14 days, but acceptable storage days are from 4 to 7 days (Lindstrom 1990). After K-sol intermediate storage media Dexsol was introduced in 1988 by Chiron Ophthalmics Inc. In addition to 1% dextran, Dexsol contains 1.35 % of chondroitin sulfate (Kaufman et al. 1991). In order to study the corneal deturgescence ability of chondroitin sulfate, a study was done by Lindstrom and associates. They found that 1.5 % chondroitin sulfate was needed to maintain the normal human corneal thickness at 4 °C and 34 °C (Lindstrom 1990). In order to preserve corneas for longer time, Optisol was introduced by Chiron Ophthalmics Inc. which has 2.5 % of chondroitin sulfate along with 1 % dextran. A comparative study done between Dexsol and Optisol with human corneas found that corneas stored in Optisol were significantly less thick than with Dexsol (Kaufman et al. 1991). In the European countries, eye banks usually use organ culture medium. Organ culture method provides several advantages like long storage time, acceptance of corneas of longer postmortem time and detection of microbial contamination prior to transplant (Pels 1997; Frueh and Bohnke 2000). Components of organ culture are Eagle’s minimum essential medium (MEM) supplemented with 2 % fetal bovine serum (FBS). Few eye banks in Europe were found to use up to 8 % FBS (Armitage 2011). Several studies have shown superiority of the organ culture over hypothermic media (Armitage et al. 2014; Bohnke 1991). These days, adjoining of the two storage techniques, i.e., hypothermic and organ culture, is a current research area in the field of corneal storage (Haug et al. 2013) (Fig. 17.1).
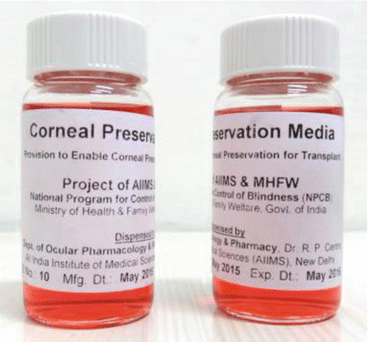
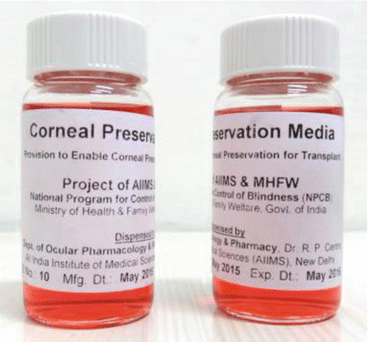
Fig. 17.1
Corneal preservation (McCareyKufman Media) media (Courtesy Dr. RPC (Pharmacy), AIIMS, N. Delhi, India)
To summarize, corneal storage media have done a long journey starting from short-term compromised storage to long-term improved storage. Still a lot is expected to happen to increase the lifespan of corneas to decrease the burden of preventable vision loss worldwide.
17.3 Tattooing Solution
Santosh Patnaik5
(5)
Ocular Pharmacology and Pharmacy, Dr. Rajendra Prasad Center for Ophthalmic Sciences, All India Institute of Medical Sciences, New Delhi, India
Tattooing solutions are used to mask the disfigured cornea. Tattooing solutions are used by ophthalmologist for cosmetically repairing the corneal opacities. There are different solutions used to tattoo the cornea. Tattooing with copper sulfate was introduced by Galen. In 1870 DeWecker used Indian ink for staining the cornea by mechanical means. In 1925 Professor Knapp introduced a method for coloring the discolored cornea by using gold chloride solution into the corneal stroma. The corneal tattooing should be done in such a way that it gives a good cosmetic effect along with permanent staining of the entire corneal opacity (Ziegler 1922; Pitz et al. 2002).
Gold or platinum chloride solutions are used for tattooing the discolored cornea. It is a simple, safe and effective method for coloring the cornea brown or black as compared to previously used Indian ink. For tattooing 2 % gold chloride or 2 % platinum chloride solution is used. The affected cornea is anesthetized by topical anesthesia and the corneal epithelium is removed from the area to be stained. Then with the help of a cotton-tipped applicator, 2 % gold chloride solution is applied to the affected area followed by 2 % hydrazine hydrate solution, till the affected area turns brown or black as desired. The tattooing technique with platinum chloride is similar to gold chloride tattooing (Duggan and Nanavati 1936; Pradhan et al. 2015) (Fig. 17.2).
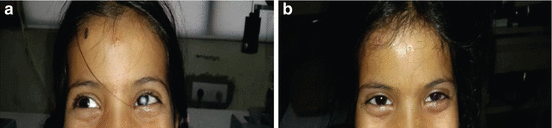
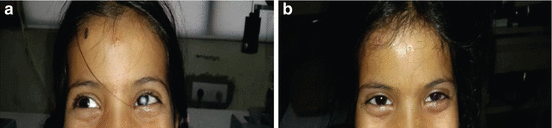
Fig. 17.2
(a) Preoperative left eye. (b) Post-tattooing left eye (Photograph courtesy Tarun Arora MD, Dr. RP Centre for Ophthalmic Sciences, AIIMS, N. Delhi)
Tattooing of the corneal opacities is a useful technique for the patients who are intolerant to cosmetic contact lenses. Tattooing of the cornea has got several advantages over wearing contact lenses. The patient is free from the maintenance of wearing a contact lens. In patient with peripheral corneal opacities with vision, corneal tattooing is an alternative to correct the cosmetic complaint without hampering the vision, whereas a colored contact lens may do so. It also gives social acceptance to the people (Pitz et al. 2002; Mannis et al. 1999).
17.4 Acid and Alkali Neutralizers
Ujjalkumar S. Das6
(6)
Ocular Pharmacology and Pharmacy, Dr. Rajendra Prasad Center for Ophthalmic Sciences, All India Institute of Medical Sciences, New Delhi, India
Chemical ocular burns constitute true ophthalmic urgencies affecting extensively the cornea, surface epithelium, conjunctiva, anterior segment and limbal stem cell system leading devastating consequences, viz., chronic pain, disfigurement and vision impairment (Spector and Fernandez 2008). It is necessary to be diagnosed and treated at the earliest to prevent from potentially blinding ocular injuries and morbidity. It needs intensive evaluation of the burn, and prompt interventions should be given immediately to restore the integrity of ocular surface and tissues. Typically its occurrence is due to accidental ingress of corrosive substances into the surface of the eye or periocular tissues. Understanding the pathogenesis of these traumatic situation leads to the development of novel agents for the treatment. The major goal of the therapy is to rejuvenate the ocular surface and corneal clarity at optimal level needed for good sight.
17.4.1 Classification of Ocular Surface Burns
Several classification systems of ocular burns do exist. Most often it is categorized either by the causative agent (physicochemical or radiation or thermal) or by assessing the extent of damage to ocular tissues particularly the corneal, limbal and conjunctival tissues at the time of injury.
Ocular burns are broadly classified into the following types:
1.
Thermal burns
Thermal injuries to the eye are due to exposure to direct flame, firework explosions, steam, boiling water, or molten metal (commonly aluminum). This constitutes a rare event, but most of the parts of the eye affected in this insult are eyelids, eyebrows and eyelashes due to the rapidity of lid reflex which protects the eye itself and visual acuity.
2.
Chemical burns
It is due to accidental exposure of the eye to chemical agents such as acid, alkali and other agents. Mostly the eye is affected by chemical burn and it accounts approximately 11.5–22.1 % of ocular injuries (Clare G et al. 2012). This type of insult to the eye can be accounted by the extent of damage to the cornea, conjunctiva and limbal tissue.
3.
Ocular injuries after radiation exposure
Direct exposure of light rays of variable wavelength is known to affect the eye due to its potential devastating effect. Several ocular tissues such as the corneal surface, retina and conjunctiva are recognized to absorb UV radiation (wavelengths 290–400 nm), infrared rays (wavelength >700 nm) and visible solar light (wavelengths 400–700 nm) which affect their functions after prolonged exposure time. UV damage to the corneal epithelium results in an inflammatory keratitis known as superficial punctuate keratitis (Brozen and Fromm 2006; Taylor 1989). Prolonged exposure to infrared radiation damages the anterior lens and may lead to cataract formation (e.g., glassblower’s cataracts). Prolonged exposure to visible solar light may rarely result in retinal injury and subsequent visual loss (Roberts 2001).
4.
Ocular injuries after biological exposure
Biological exposure refers to contact of the eye to human blood or body fluids (saliva, semen, urine) which can serve as a potential source of infection. Blood-borne pathogens include hepatitis B virus (HBV), hepatitis C virus (HCV) and HIV lead to infection if it makes direct contact with the mucous membrane of the eye. The probability of infection is related to the amount (volume) and concentration (viral load) of the biological exposure (Zaleznik 2007; CDC 2003).
17.4.2 Roper-Hall System of Classification of Ocular Chemical Injuries
The classification system to categorize the chemical ocular injuries is first proposed by Ballen (1964) which was later modified based on the severity of damage to the cornea, limbal (limbal ischemia) and conjunctival tissues which divides the ocular chemical injuries into 4 grades (Roper-Hall 1965). Till now there are several classification systems available, but Roper-Hall classification is widely used by clinicians for prompt recognition of the severity of disease (Table 17.2).
Table 17.2
Classification of ocular surface burns by Roper-Hall
Grade | Clinical finding | Prognosis | |
---|---|---|---|
Cornea | Conjunctiva/limbus | ||
I | Corneal epithelial damage | No limbal ischemia | Good |
II | Corneal haze, iris details visible | <1/3 limbal ischemia | Good |
III | Total epithelial loss, stromal haze, iris details obscured | 1/3–1/2 limbal ischemia | Guarded |
IV | Cornea opaque, iris and pupil obscured | >limbal ischemia | Poor |
17.4.3 Dual System of Classification (Newer Classification of Ocular Chemical Injuries)
Dua et al. (2001) proposed a new classification for ocular chemical injuries which is a modification to Roper-Hall classification. This modification has taken into account the extent of limbal involvement in clock hours and the percentage of conjunctival involvement. This system classifies chemical surface burns into six grade (Table 17.3).
Table 17.3
Newer classification of ocular surface burns
Grade | Prognosis | Clinical findings | Conjunctival involvement | Analog scale |
---|---|---|---|---|
I | Very good | 0 clock hours of limbal involvement | 0 % | 0/0 % |
II | Good | ≤3 clock hours of limbal involvement | ≤30 % | 0.1–3/1–29.9 % |
III | Good | >3–6 clock hours of limbal involvement | >30–50 % | 3.1–6/31–50 % |
IV | Good to guarded | >6–9 clock hours of limbal involvement | >50–75 % | 6.1–9/51–75 % |
V | Guarded to poor | >9 to < clock hours of limbal involvement | >75 to <100 % | 9.1–11.9/75.1–99.9 % |
VI | Very poor | Total limbus (12 clock hours) involved | Total conjunctiva (100 %) involved | 12/100 % |
17.4.4 Pathophysiology
Understanding pathogenesis of these severe ocular traumatic conditions leads to the better prognosis rate of the disease and provides treatment options. Severity and recovery both depend on the type of causative agent, volume, concentration, duration of exposure and extent of penetration into ocular tissues (Pfister and Pfister 2005a, b). The mechanism followed by acid and alkali burn is quite different and explained as below.
17.4.4.1 Acid Burns
Acids dissociate into hydrogen ions and anion in the cornea. This usually occurs when a strong acid has a pH of less than 4. The hydrogen molecule damages the ocular surface by altering the pH, while the anion causes protein denaturation, precipitation and coagulation. Protein coagulation creates a barrier and thus generally prevents deeper penetration of acids and is responsible for the ground glass appearance of the corneal stroma following acid injury. Hydrofluoric acid is an exception; it behaves like an alkaline substance because the fluoride ion has better penetrance through the stroma, leading to the same spectrum and more extensive anterior segment disruption (Pfister and Pfister 2005a).
After entering into anterior chamber, it reacts with collagen resulting in shortening of collagen fibers which cause a rapid increase in intraocular pressure (IOP). After severe acid burns with ciliary body damage, decrease in levels of aqueous ascorbate has been demonstrated.
17.4.4.2 Alkali Burns
Alkaline substances are lipophilic and can penetrate cell membranes. They dissociate into a hydroxyl ion and a cation in the ocular surface. The hydroxyl ion saponifies cell membrane fatty acids, while the cation interacts with stromal collagen and glycosaminoglycans. This interaction facilitates deeper penetration into and through the cornea and into the anterior segment. Subsequent hydration of glycosaminoglycans results in stromal haze. Collagen hydration causes fibril distortion and shortening, leading to trabecular meshwork alterations that can result in increased intraocular pressure (IOP). Additionally, the inflammatory mediators released during this process stimulate the release of prostaglandins, which can further increase IOP (Pfister and Pfister 2005b). The damage to the corneal and conjunctival epithelium leads to the damage of the limbal stem cell causing limbal stem cell deficiency (LSCD). This in turn resulted in opacification and neovascularization in the cornea (Table 17.4).
Products | Chemical | |
---|---|---|
Acid burns | Toilet cleaner | Sulfuric acid (80 %) |
Battery fluid | Sulfuric acid (30 %) | |
Pool cleaners | Sodium or calcium hypochlorite (70 %) | |
Bleaches | Sodium hypochlorite (3 %) | |
Vinegar | Acetic acid | |
Glass polishers, rust removal agents, silicon production agents | Hydrofluoric acid | |
Food- and leather-processing compounds | Hydrochloric acid | |
Alkali burns | Lime | Calcium or magnesium carbonate |
Plaster and mortar | Calcium hydroxide | |
Oven and drain cleaner | Sodium or potassium hydroxide | |
Fireworks and sparklers | Magnesium hydroxide | |
Ammonia (chemical agents and fertilizers) | Ammonium hydroxide | |
Dishwater detergent | Sodium tripolyphosphate |
17.4.5 Therapeutic Interventions for Management
Immediate initiation of management for ocular chemical injuries influences the final outcome favorably, namely, restoration of the normal ocular surface anatomy and lid position, control of glaucoma and restoration of corneal clarity. Management of chemical burns is based on the basic mechanism of the initial incident and the subsequent inflammatory response. It can be subdivided into the following types based on the timing of the treatment.
17.4.5.1 Emergency Therapy
After an acute chemical burn, copious irrigation is necessary to wash out the offending chemicals. Irrigation is performed with isotonic saline or lactate ringer solution or Morgan Lens to change pH to physiological levels and provide medication to the cornea and conjunctiva. This is followed by removal of any remaining particles from the ocular surface with a moist cotton tip or fine-tipped forceps (Eslani et al. 2014). Thus, emergency therapy represents the first line of management of ocular injuries and whose efficacy significantly affects further prognosis of the injury.
17.4.5.2 Acute Phase Treatment
Acute phase treatment proceeds emergency treatment and is based on the ophthalmic evaluation of the injuries done at emergency stage. It basically employs broad-spectrum topical antibiotic, cycloplegic and antiglaucoma therapy to promote reepithelialization, support repair and control inflammation. The different modalities (Table 17.5) employed in the acute phase treatment aim at reestablishment and maintenance of an intact and healthy corneal epithelium, control of the balance between collagen synthesis and collagenolysis and minimizing the adverse sequelae that often follow a chemical injury (Singh et al. 2013).
Table 17.5
Different treatment modalities for acute phase of chemical injuries
Treatment | Drugs |
---|---|
Promoting reepithelialization | Tear substitutes, bandage soft contact lens, Boston scleral lens, autologous serum, retinoic acid, epidermal growth factor and fibronectin, systemic ascorbic acid |
Repair and minimizing ulceration | Ascorbate, collagenase inhibitors including cysteine, acetylcysteine, sodium ethylenediamine tetra acetic acid (EDTA), calcium EDTA, penicillamine and citrate |
Anti-inflammatory therapy | Corticosteroids like topical prednisolone 0.5 % in conjunction with topical ascorbate 10 % |
17.4.5.3 Early Reparative Phase Treatment
If an intact epithelium is not achieved by the 14th day after injury, then corticosteroids dosage is tapered and discontinued and aggressive therapy is followed through the use of lubricants, punctual plugs, punctal occlusion with cautery, bandage contact lens and tarsorrhaphy. In addition antibiotic, ascorbate, citrate and antiglaucoma therapy are continued and examination for the formation of symblepharon and corneal thinning are done (Singh et al. 2013). A symblepharon ring can be placed in the fornices to effectively prevent symblepharon formation. Proteinase inhibitors such as aprotinin and collagenase inhibitors such as cysteine, acetylcysteine and chelators such as sodium ethylenediamine tetra acetic acid (EDTA), calcium EDTA, penicillamine, citrate and especially tetracyclines have been found to prevent corneal thinning in chemically burned corneas (Wagoner 1997).
17.4.5.4 Surgical Management
Surgical management falls in the late reparative phase of treatment when an intact epithelium has not developed by the 21st day after injury leading to a significant risk of permanent vision loss (Eslani et al. 2014). The primary goal of early surgical management is to maintain the globe and promote reepithelialization. Early surgical management starts with initial debridement of the necrotic material, amniotic membrane transplantation and tectonic grafting if necessary. Late surgical interventions including limbal stem cell transplantation and keratoplasty are aimed at restoring the normal ocular surface anatomy and visual function (Singh et al. 2013).
17.5 Vitreous Substitutes
Kanuj Mishra7
(7)
Department of Biotechnology, All India Institute of Medical Sciences, New Delhi, India
The vitreous body is a natural polymeric hydrogel composed primarily of type 2 collagen and hyaluronic acid and performs the essential physiological functions of intraocular pressure maintenance and ocular nourishment. Disruptions in this delicate architecture lead to the separation of its components (syneresis), creating the conditions for vitreoretinal disease (Foster 2008). Vitreous substitutes have been in use for almost a century to repair numerous complex vitreoretinal conditions successfully, such as most retinal detachments, giant retinal tears, macular holes and complex detachments. The ideal vitreous substitute should mimic the native vitreous in both form and function and be transparent, nontoxic, elastic and biocompatible for the long term. For practical reasons, it should also be easily manipulable during surgery, easily available, stable during storage, injectable through a small syringe and available at a reasonable cost. Advances in vitreoretinal surgical techniques, instrumentation and material technology have led to an array of agents from which vitreoretinal surgeons can choose (Torsten and Caroline 2007). The vitreous substitutes can be classified based on their availability, composition and physical properties into the following types:
17.5.1 Conventional Vitreous Substitutes
17.5.1.1 Air
This was the earliest vitreous substitutes developed by Ohm in 1911 when he treated retinal detachment with injection of sterile air into the vitreous cavity. Now the gases are mainly used intraoperatively in pneumatic retinopexy, at the end of vitrectomy surgery to facilitate retinal reattachment. Air facilitates and maintains retinal reattachment through the high surface tension of the gas bubble (Foster 2008).
17.5.1.2 Expansile Gas-Based Substitutes
Expansile gases have been used since the early 1970s and are the most effective short-term vitreous substitutes for retinal detachment repair since the early 1990s. These gases are colorless, odorless, heavier than air and nontoxic to the human eye. Sulfur hexafluoride (SF6) and perfluoropropane (C3F8) are the common class of gas-based substitutes available and are used in non-expansile concentrations to fill the vitreous cavity in pneumatic retinopexy. Gas-based substitutes have the highest surface tension of all vitreous fluid replacements (70 dyn/cm) which aids them to be expansile and to maintain a tamponade effect (Torsten and Caroline 2007). They are replaced with aqueous humor, avoiding a second surgery to remove them (Kleinberg et al. 2011).
17.5.1.3 Liquids
Perfluorocarbon
Perfluorocarbon liquid (PFCL) are fluorinated, carbon-containing hydrophobic compounds that are clear, colorless and odorless. These liquids were developed as blood substitutes due to their inert nature and ability to carry oxygen. The intraoperative uses of perfluorocarbon liquids depend on their high specific gravity (1.7–2.03) by the property of which a unique gravitational force is applied on the dependent portion of retina (Azad 2012). Commonly used PFCLs are perfluoro-n-octane (C8F18), perfluorodecalin (C10F18), perfluoroperhydrophenanthrene (C14F24) and perfluorohexyloctane (C6F13C8H18). Perfluorocarbon liquids have been successfully used in the management of proliferative vitreoretinopathy (PVR), repair of detachments secondary to ocular trauma and removal of dislocated crystalline lenses. Perfluorocarbon liquids are being primarily as a short-term intraoperative vitreous substitute and long-term complications are rare (Foster 2008).
Silicone Oil (SO)
The use of silicone oil as a long-acting vitreous substitute was pioneered by Cibis in 1962 for complex retinal detachments (Yang et al. 2014). Silicone oil refers to the polymers of polydimethylsiloxane and is the only substance currently accepted for long-term vitreous replacement. The most commonly available silicone oils have viscosities of 1000 centistokes (cS) and 5000 cS and contain trimethylsiloxyl-terminated polydimethylsiloxane, but standards have yet to be established and batch variation may exist. Silicone oil is slightly lighter than water with a specific gravity of 0.97 and during substitution, a silicone globule floats on a residual aqueous base inside the eye. Silicone oil represents a more versatile replacement than air or gas and is widely used for giant retinal breaks, hypotony due to chronic uveitis, infectious retinitis and finally retinal detachments where postoperative positioning is not possible. Postsurgical and late complications of intraocular silicone oil fills are common and may require removal of the oil earlier than the desired duration (Foster 2008).
The available replacements each have their specific advantages and drawbacks (Table 17.6). Hence the recent thrust is on the development of substitutes that not only serve as retinal tamponades but also satisfy biomechanical aspect of vitreous. These recently developed substitutes can be classified into two types – newer vitreous substitutes and experimental vitreous substitutes based on their stage of development.
Table 17.6
Advantages and disadvantages of different class of vitreous replacements
Class | Advantage | Disadvantage |
---|---|---|
Air | Inexpensive Readily available | Less intravitreal residence time (2–3 days) Cataract corneal endothelial damage, raised IOP |
Expansile gas-based substitutes | Good success rate, exceeding 90 % for retinopexy | Limited to treating the upper retina gas-induced cataract formation corneal endothelial changes raised IOP central retinal artery occlusion |
Perfluorocarbon | The low viscosity of PFCLs allows for tissue manipulation, injection and removal | During prolonged usage decrease in vitreous clarity, compromise of the trabecular meshwork with subsequent IOP rises and subretinal migration of droplets through residual breaks |
Silicone oil | Suppression of future neovascularization bactericidal properties has 70 % success rate in preserving anatomical integrity | Emulsification, corneal decompensation, band keratopathy, cataract and secondary glaucoma. SO removal has been accompanied by visual acuity loss, hypotony and corneal abnormalities |
17.5.2 Newer Vitreous Substitutes
17.5.2.1 Semifluorinated Alkanes
Semifluorinated alkanes (SFAs), also known as partially fluorinated alkanes (PFAs) or fluorinated alkanes, were investigated for their vitreous replacement properties in the early 2000s. SFAs were initially used as a solvent for SO and then as a temporary vitreous substitute for special cases of retinal detachment when silicone oil failed to function properly. Their low specific gravity (compared to PFCLs) and high interface tension (compared to silicone oil) are thought to produce less retinal damage and bridge larger retinal breaks (Azad 2012). Clinical trials have shown SFAs to be tolerated for extended periods of 2–3 months and they are being pioneered the first internal tamponade agents to be used beyond the intraoperative setting (Kleinberg et al. 2011).
17.5.2.2 Silicone Oil/Semifluorinated Alkanes Combinations
This combination takes advantage of the high viscosity of SO and high specific gravity of the SFAs to produce a vitreous substitute with a good tamponade effect and lesser chance of emulsification. Depending on the ratio of the two liquids, either a homogenous clear solutions (heavy silicone oils) or separated solutions (double fills) are developed (Azad 2012). Double fill (DF) was developed with the goal of having the light SO support the superior retina, while the heavier SFA supports the inferior retina. DF has been reported to have the best results in complicated surgeries with large inferior retinal breaks. Heavy silicone oil (HSO) is heavier than water and is created by combining SO and a PFA in such a way as to create a homogenous solution. HSO is being promoted as a long-term replacement agent for complex retinal detachments involving inferior proliferative vitreoretinopathy (Kleinberg et al. 2011).
17.5.3 Experimental Vitreous Substitutes
17.5.3.1 Hydrogels
Hydrogels are three-dimensional hydrophilic polymers that swell in aqueous solutions without dissolving and form a gel network through cross-linkage. They are clear viscoelastic gel that strongly resembles the natural vitreous humor (Azad 2012). Hydrogels were the first biomaterials synthesized for human use, but in vivo research on hydrogels as vitreous replacements is still in the animal model stage. Because of the early experimental nature of the materials, complications and efficacy associated with hydrogels are not well known.
17.5.3.2 Implants
Implantable devices may be able to support the retina without the need of a potentially immunoreactive intravitreally injected solution. Foldable capsular vitreous bodies (FCVB) injected with saline showed good biocompatibility and retinal support in rabbit eyes over a 180-day implantation time. However, the feasibility of a synthetic implant in the human eye is untested and longer-term animal data are first needed to assess the potential use of this approach (Kleinberg et al. 2011).
The intraocular vitreous body is a highly complex macromolecular structure and despite significant progress over the past few decades, ideal, universal vitreous substitute does not exist. Recently, in situ-forming zwitterionic hydrogels have been developed as a promising vitreous substitute (Chang et al. 2015). Thus, advancements in material sciences coupled with extensive molecular understanding of vitreous components could aid in a plethora of compound that could facilitate longer and more efficient vitreous replacement.
17.5.4 Chemical Agents for Vitreolysis
The earliest definition of vitreolytic agents was given by Sebag et al. 1998 as “agents that alter the molecular organization of vitreous in an effort to reduce or eliminate its role in disease.”
The vitreous is involved in multiple diseases when an incomplete posterior vitreous detachment (PVD) occurs. Incomplete PVD is considered pathological when associated with focal adhesions, causing vitreomacular traction (VMT) syndrome, vitreopapillary adhesion, tractional diabetic macular edema (DME), peripheral retinal breaks and rhegmatogenous retinal detachment or when associated with vitreoschisis (hyaloid delamination), predisposing to epiretinal membrane, macular hole and diabetic retinopathy neovessel proliferation and recurrence (Gandorfer 2012).
Vitreous liquefaction and posterior vitreous separation have to be done through pharmacological vitreolysis to obtain a safe and complete PVD. There are several vitreolytic agents that have been studied to date. They can be categorized as “enzymatic” or “nonenzymatic,” according to their mechanism of action and as “liquefactants (induce a vitreous liquefaction) or interfactants” (induce dehiscence at the vitreoretinal interface on the basis of their biological effect) (Table 17.7). Some of the most studied vitreolytic agents are discussed below:
Table 17.7
Classification of vitreolytic agents on the basis of their mechanism of action and biological effects
Interfactants | Liquefactants | Combination | |
---|---|---|---|
Enzymatic | Dispase | Hyaluronidase | Plasmin |
Collagenase | Microplasmin | ||
tPA/plasminogen | |||
Nattokinase | |||
Chondroitinase | |||
Nonenzymatic | RGD peptides | Vitreosolve |
17.5.4.1 Enzymatic Vitreolytic Agents
Dispase
Dispase is a protease which cleaves fibronectin, collagen IV and collagen I (to a lesser extent). Studies in animal and human models document the efficacy of dispase to cleave the attachment between the posterior hyaloid and the internal limiting membrane (ILM), with minimal morphological changes to the inner retina (Tezel et al. 1998; Oliveira et al. 2002). Despite its usefulness as an adjunct in facilitating surgical creation of PVD harmful effects including proliferative vitreoretinopathy, retinal hemorrhages, cataract, lens subluxation and retinal toxicity have been reported (Jorge et al. 2003; Wang et al. 2004; Zhu et al. 2006; Frenzel et al. 1998; Kralinger et al. 2006; Ivastinovic et al. 2012).
Hyaluronidase
Hyaluronidase is the only agent for enzymatic vitreolysis which has been evaluated in phase III clinical trials (Kuppermann et al. 2005). Hyaluronidase cleaves the glycosidic bonds of hyaluronan as well as other mucopolysaccharides resulting in dissolution of the hyaluronan and collagen complex and subsequent vitreous liquefaction. Hyaluronidase has been considered as liquefactant because of its property of dissolving the glycosaminoglycan network of the vitreous gel. But several other studies have concluded hyaluronidase alone to be ineffective in inducing PVD suggesting a combination of vitreolytic agents with hyaluronidase (Zhi-Liang et al. 2009; Wang et al. 2005; Narayanan and Kuppermann 2009).
Collagenase
Collagenase is a liquefactant obtained from Clostridium histolyticum which selectively cleaves the type II collagen that constitutes the fibrillar network of the vitreous gel. Cleaved type II collagen forms soluble proteolyzed fragments allowing for spontaneous denaturation and further degradation by nonspecific proteases. Its liquefactant action has been successfully established in animal models, and found to be associated with inner limiting membrane damage and disruption of retinal architecture (O’Neill and Shea 1973) or histological and electrophysiological toxicity (Moorhead et al. 1980, 1983).
Plasmin and Ocriplasmin
Plasmin is a nonspecific serine protease with a critical role in fibrinolysis and the most studied vitreolytic agent. It directly degrades fibrin and other extracellular matrix components including laminin and fibronectin (Liotta et al. 1981; Uemura et al. 2005; Li et al. 2002) and may also indirectly generate increased levels of other nonspecific proteases such as matrix metalloproteinases and elastase (Bandello et al. 2013). These downstream activities enhance the primary action of plasmin in weakening the vitreoretinal insertion. Thus, it is capable of degrading the vitreous gel and of cleaving further ECM structures, serving as a liquefactant as well as an interfactant. Various preclinical studies have concluded plasmin to be an excellent vitreolytic agent through ERG examinations and histological analysis at doses up to 4 U and with exposure times ranging from 30 min to 1 week in which no pathological tissue damage and functional changes were noted (Bandello et al. 2013). However, the process for isolating autologous plasmin is cumbersome and the enzymatic activity is dependent on multiple variables including the level of plasminogen in the blood. As a result, the amount of enzymatic activity obtained is variable and difficult to quantify.
Ocriplasmin, formerly known as microplasmin, is a recombinant product of plasmin which alleviated most of the problems associated with autologous plasmin (Chen et al. 2008; Sebag et al. 2007). It is a truncated form of plasmin that lacks the five kringle domains of plasmin but contains the protease domain making it a smaller molecule than plasmin (27 kD compared to 80 kD) but with similar enzymatic activity. Advantage of being recombinant is that microplasmin can be produced in large quantity supplementing the need of use in different dose-ranging studies. Animal and ex vivo studies demonstrate that microplasmin creates both posterior vitreous detachment and vitreous liquefaction without evidence of retinal toxicity.
< div class='tao-gold-member'>
Only gold members can continue reading. Log In or Register a > to continue
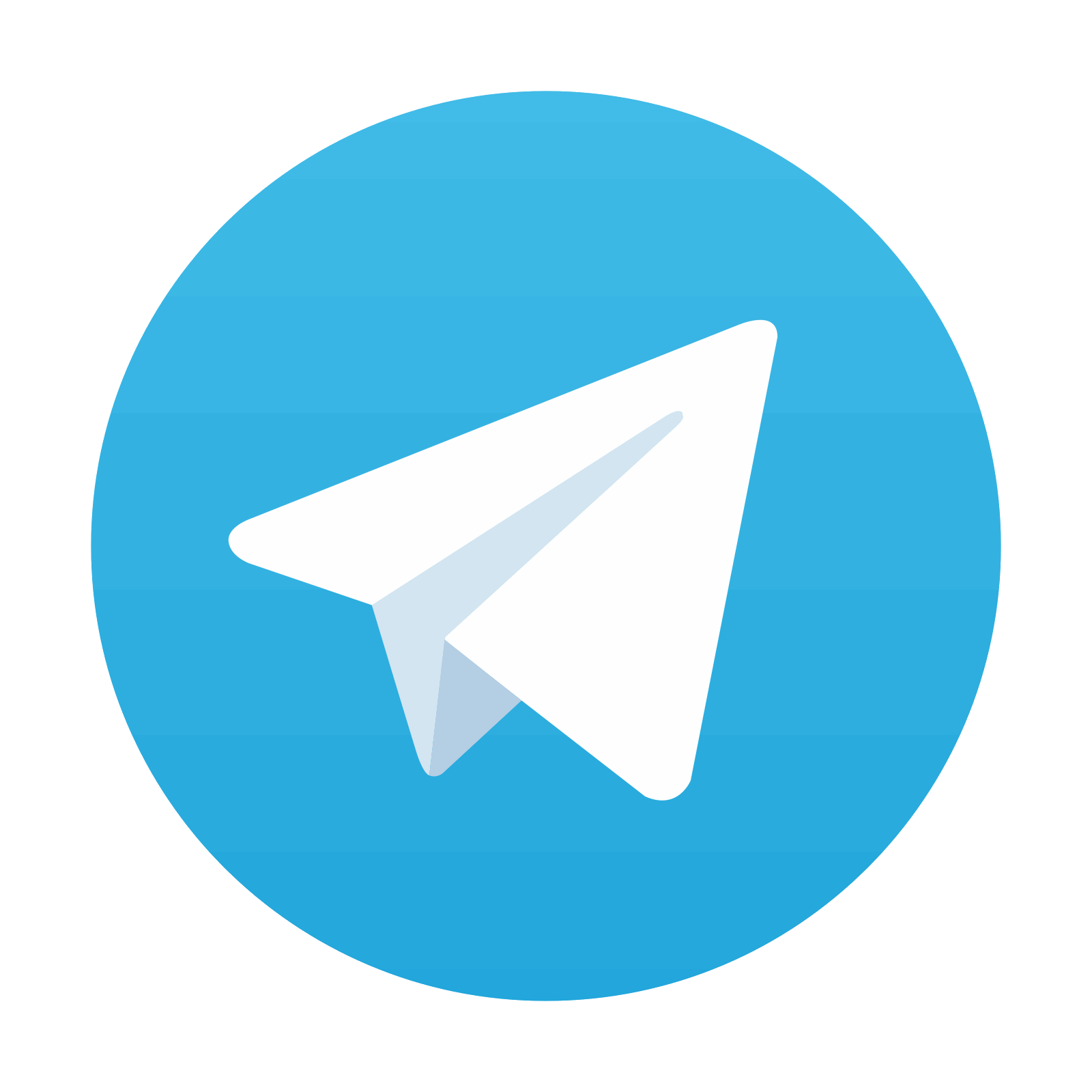
Stay updated, free articles. Join our Telegram channel

Full access? Get Clinical Tree
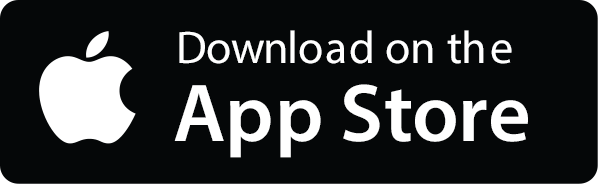
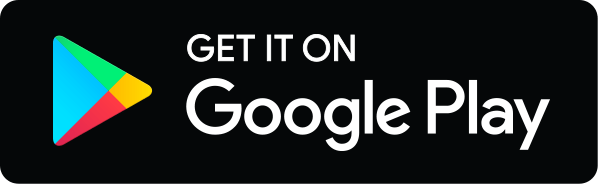