2.1 INTRODUCTION TO METHODS USED IN VIROLOGY
Methods used in virology are introduced early in this book in order to provide an appreciation of the nature of the techniques that have been used to achieve our current level of knowledge and understanding of viruses. Virology is a huge subject and uses a wide range of methods. Many of the techniques of molecular biology and cell biology are used, and constraints on space permit us to mention only some of them. Much of the focus of this chapter is on methods that are unique to virology. Many of these methods are used not only in virus research but also in the diagnosis of virus diseases of humans, animals, and plants.
Initially this chapter could be skimmed to gain an overview of its contents and thereafter used for reference. Details of the methods outlined here, and of other methods important in virology, fill many volumes, some of which are listed at the end of the chapter.
2.2 CULTIVATION OF VIRUSES
Virologists need to be able to produce the objects of their study, so a wide range of procedures has been developed for cultivating viruses. Virus cultivation is also referred to as propagation or growth, all terms borrowed from horticulture! A few techniques have been developed for the cultivation of viruses in cell-free systems, but in the vast majority of cases it is necessary to supply the virus with appropriate cells in which it can replicate.
Phages are supplied with bacterial cultures and plant viruses may be propagated using specially cultivated plants, tissue cultures or protoplasts (plant cells from which the cell wall has been removed). Animal viruses may be propagated in whole organisms, such as mice, which may be genetically modified. Transgenic mice are used in studies of hepatitis B and hepatitis C viruses. Some vertebrate viruses are propagated in eggs containing chick embryos (Figure 2.1), while some insect viruses are propagated in insect larvae. For the most part, however, animal viruses are grown in cultured animal cells.
Figure 2.1 Cultivation of viruses in eggs containing chick embryos.
Source: Photograph courtesy of Taronna Maines and Greg Knobloch, Centers for Disease Control and Prevention.
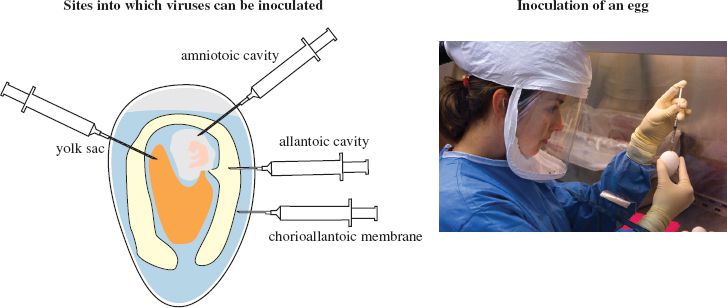
2.2.1 Animal cell culture
Animal cell culture techniques are well developed and most of the cells used are from continuous cell lines derived from humans and other animal species. Continuous cell lines consist of cells that have been immortalized, either in the laboratory or in the body (Figure 2.2); they can be subcultured indefinitely. The HeLa cell line is a widely used continuous cell line that was initiated in the middle of the twentieth century from cells taken from a cervical carcinoma.
Figure 2.2 Derivation of continuous cell lines of human and animal cells. Most types of cell taken from the body do not grow well in culture. If cells from a primary culture can be subcultured they are growing as a cell line. They can be subcultured only a finite number of times unless they are immortalized, in which case they can be subcultured indefinitely as a continuous cell line. Cancer cells are already immortalized, and continuous cell lines may be established from these without further treatment.
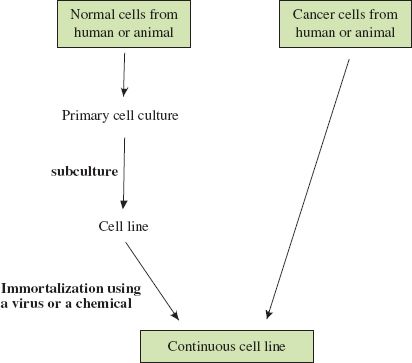
Sometimes it is difficult to find a cell line in which a virus can replicate. For many years no suitable system could be found to support the replication of hepatitis C virus, but eventually the obstacles were overcome and this virus can now be cultured in a human hepatoma cell line and in primary human hepatocytes.
Cells are cultured in media that provide nutrients. Most media are supplemented with animal serum, which contains substances that promote the growth of many cell lines. Other important roles for the medium are the maintenance of optimum osmotic pressure and pH for the cells. Viruses can be cultivated in cells growing on the surface of a variety of plastic vessels (Figure 2.3) with the cells bathed in the growth medium. Most cells grow on a plastic or glass surface as a single layer of cells, known as a monolayer. Alternatively the cells can be suspended in the medium, which is stirred to keep them in suspension.
Figure 2.3 Cell culture flasks, dishes, and plates.
Source: Photographs of TPP cell culture products courtesy of MIDSCI.
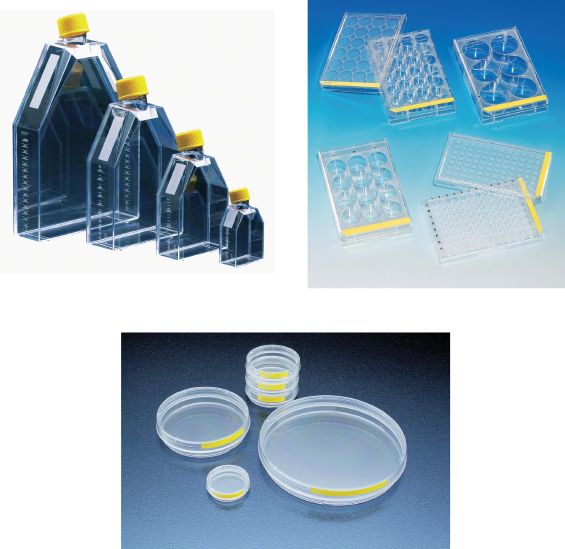
Contamination with bacteria and fungi can cause major problems in cell culture work; in order to minimize these problems work is normally done in a sterile cabinet (Figure 2.4) and most media contain antibiotics. Many cell types require a relatively high concentration of carbon dioxide, which can be supplied in a special incubator.
Figure 2.4 Cell culture work. Precautions to avoid contamination include working in a sterile cabinet and wearing gloves and mask.
Source: Courtesy of Sanofi Pasteur—Copyright.
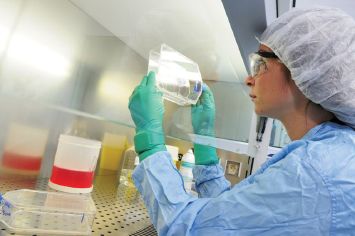
2.3 ISOLATION OF VIRUSES
Many viruses can be isolated as a result of their ability to form discrete visible zones (plaques) in layers of host cells. If a confluent layer of cells is inoculated with an amount of virus to infect a small proportion of the cells, then plaques may form where areas of cells are killed or altered by the virus infection. Each plaque is formed when infection spreads radially from an infected cell to surrounding cells.
Plaques can be formed by many animal viruses if the cell monolayers are overlaid with agarose gel to maintain the progeny virus in a discrete zone (Figure 2.5). Plaques can also be formed by phages in lawns of bacterial growth (Figure 2.6).
Figure 2.5 Method for production of plaques by animal viruses.
Source: Photograph of influenza virus plaques from Ma et al. (2011) Virology, 410, 1. Reproduced by permission of Elsevier.
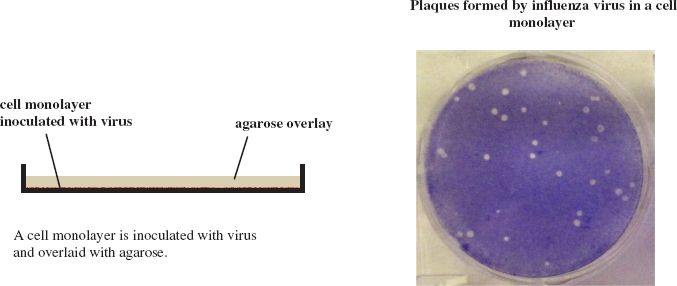
Figure 2.6 Plaques formed by a phage in a bacterial lawn. The control plate on the left was inoculated with only the bacterial host. The plate on the right was inoculated with phage and bacterial host.
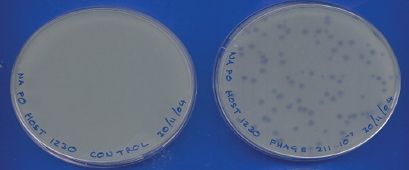
It is generally assumed that a plaque is the result of the infection of a cell by a single virion. If this is the case then all virus produced from virus in the plaque should be a clone, in other words it should be genetically identical. This clone can be referred to as an isolate, and if it is distinct from all other isolates it can be referred to as a strain. This is analogous to the derivation of a bacterial strain from a colony on an agar plate.
There is a possibility that a plaque might be derived from two or more virions so, to increase the probability that a genetically pure strain of virus has been obtained, material from a plaque can be inoculated onto further monolayers and virus can be derived from an individual plaque. The virus is said to have been plaque purified.
When a virus is first isolated it may replicate poorly in cells in the laboratory, but after it has gone through a number of replication cycles it may replicate more efficiently. Each time the virus is “subcultured” (to borrow a term from bacteriology) it is said to have been passaged. After a number of passages the virus may be genetically different to the original wild strain, in which case it is now a laboratory strain.
2.4 CENTRIFUGATION
After a virus has been propagated it is usually necessary to remove host cell debris and other contaminants before the virus particles can be used for laboratory studies, for incorporation into a vaccine, or for some other purpose. Many virus purification procedures involve centrifugation; partial purification can be achieved by differential centrifugation and a higher degree of purity can be achieved by some form of density gradient centrifugation.
2.4.1 Differential centrifugation
Differential centrifugation involves alternating low-speed centrifugation, after which most of the virus is still in the supernatant, with high-speed centrifugation, after which the virus is in the pellet (Figure 2.7).
Figure 2.7 Partial purification of virions by differential centrifugation. A crude preparation of virus containing host debris is subjected to low-speed/short-time centrifugation (e.g. 10 000 g/20 minutes) followed by high-speed/long-time centrifugation (e.g. 100 000 g/2 hours). This cycle can be repeated to obtain a higher degree of purity. The final pellet containing partly purified virus is resuspended in a small volume of fluid.
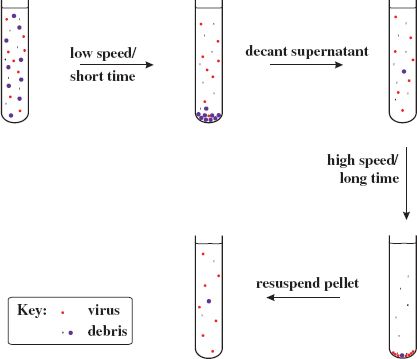
2.4.2 Density gradient centrifugation
Density gradient centrifugation involves centrifuging particles (such as virions) or molecules (such as nucleic acids) in a solution of increasing concentration, and therefore density. The solutes used have high solubility; sucrose is commonly used. There are two major categories of density gradient centrifugation: rate zonal and equilibrium (isopycnic) centrifugation (Figure 2.8).
Figure 2.8 Purification of virions by density gradient centrifugation. A partly purified preparation of virus is further purified in a density gradient. Rate zonal centrifugation involves layering the preparation on top of a pre-formed gradient. Equilibrium centrifugation can often be done starting with a suspension of the impure virus in a solution of the gradient material; the gradient is formed during centrifugation.
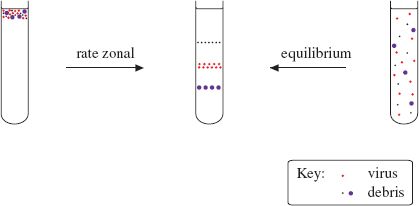
In rate zonal centrifugation a particle moves through the gradient at a rate determined by its sedimentation coefficient, a value that depends principally on its size. Homogeneous particles, such as identical virions, should move as a sharp band that can be harvested after the band has moved part-way through the gradient.
In equilibrium centrifugation a concentration of solute is selected to ensure that the density at the bottom of the gradient is greater than that of the particles/molecules to be purified. A particle/molecule suspended in the gradient moves to a point where the gradient density is the same as its own density. This technique enables the determination of the buoyant densities of nucleic acids and of virions. Buoyant densities of virions determined in gradients of cesium chloride are used as criteria in the characterization of viruses.
2.5 STRUCTURAL INVESTIGATIONS OF CELLS AND VIRIONS
2.5.1 Light microscopy
The sizes of most virions are beyond the limits of resolution of light microscopes, but light microscopy has useful applications in detecting and studying virus-induced changes in cells, for example observing cytopathic effects (Section 2.7. 2). Fluorescence microscopy is used to detect virus products labeled with fluorophores (molecules that absorb light at certain wavelengths then emit light at longer wavelengths; Section 2.7. 3).
Confocal microscopy is proving to be valuable in virology. The principle of this technique is the use of a pinhole to exclude light from out-of-focus regions of the specimen. Most confocal microscopes scan the specimen with a laser, producing exceptionally clear images of thick specimens and of fluorescing specimens. Furthermore, “optical slices” of a specimen can be collected and used to create a three-dimensional representation. The techniques can be used with live cells and can be applied to investigations of protein trafficking, with the virus or cell protein under investigation carrying a suitable label, e.g. green fluorescent protein (a jellyfish protein).
2.5.2 Electron microscopy
Many investigations of the structure of virions or of virus-infected cells involve observations at large magnifications using transmission electron microscopes. The specimen may be a preparation of virions, a virion component, virus-infected cells or an ultra-thin section of a virus-infected cell. In order to observe detail in the specimen it is either negatively stained, or cooled to a very low temperature, or both.
Negative staining techniques generate contrast by using heavy metal-containing compounds, such as potassium phosphotungstate and ammonium molybdate. In electron micrographs of virions the stains appear as dark areas around the virions, allowing the overall virion shape and size to be determined. Further structural detail may be apparent if the stains penetrate any crevices on the virion surface or any hollows within the virion. Negative staining techniques have generated many high quality electron micrographs, but the traditional techniques have limitations, including structural distortions resulting from drying.
Cryo-electron microscopy techniques are more recent. For these techniques wet specimens are rapidly cooled to a temperature below –160 °C, freezing the water as a glass-like material. The images are recorded while the specimen is frozen. They require computer processing in order to extract maximum detail, and data from multiple images are processed to reconstruct three-dimensional images of virus particles. This may involve averaging many identical copies or combining images into three-dimensional density maps (tomograms).
2.5.3 X-ray crystallography
X-ray crystallography is another technique that is revealing detailed information about the three-dimensional structures of virions (and DNA, proteins, and DNA–protein complexes). This technique requires the production of a crystal of the virions or molecules under study. The crystal is placed in a beam of X-rays, which are diffracted by repeating arrangements of molecules/atoms in the crystal. Analysis of the diffraction pattern allows the relative positions of these molecules/atoms to be determined.
Other techniques that are providing useful information about the structure of viruses are nuclear magnetic resonance and atomic force microscopy.
2.6 ELECTROPHORETIC TECHNIQUES
Mixtures of proteins or nucleic acids can be separated by electrophoresis in a gel composed of agarose or polyacrylamide. In most electrophoretic techniques, each protein or nucleic acid forms a band in the gel. Electrophoresis can be performed under conditions where the rate of movement through the gel depends on molecular weight. The molecular weights of the protein or nucleic acid molecules can be estimated by comparing the positions of the bands with positions of bands formed by molecules of known molecular weight electrophoresed in the same gel. The technique for estimating molecular weights of proteins is polyacrylamide gel electrophoresis in the presence of the detergent sodium dodecyl sulfate (SDS-PAGE; Figure 2.9).
Figure 2.9 Diagram illustrating separation of proteins and estimation of their molecular weights using SDS-PAGE. Lanes 1 and 3 contain standard proteins of known molecular weight. Lane 2 contains the four capsid proteins of a picornavirus.
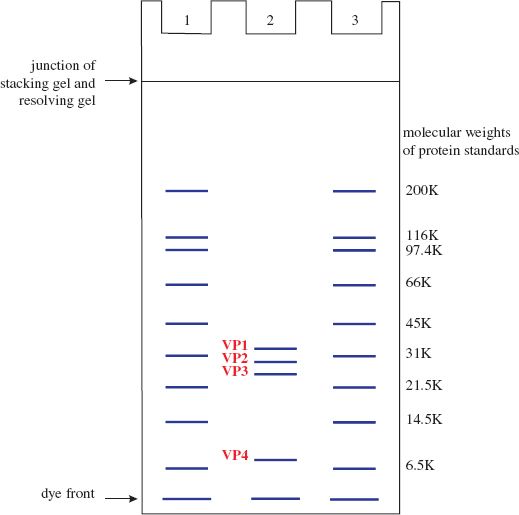
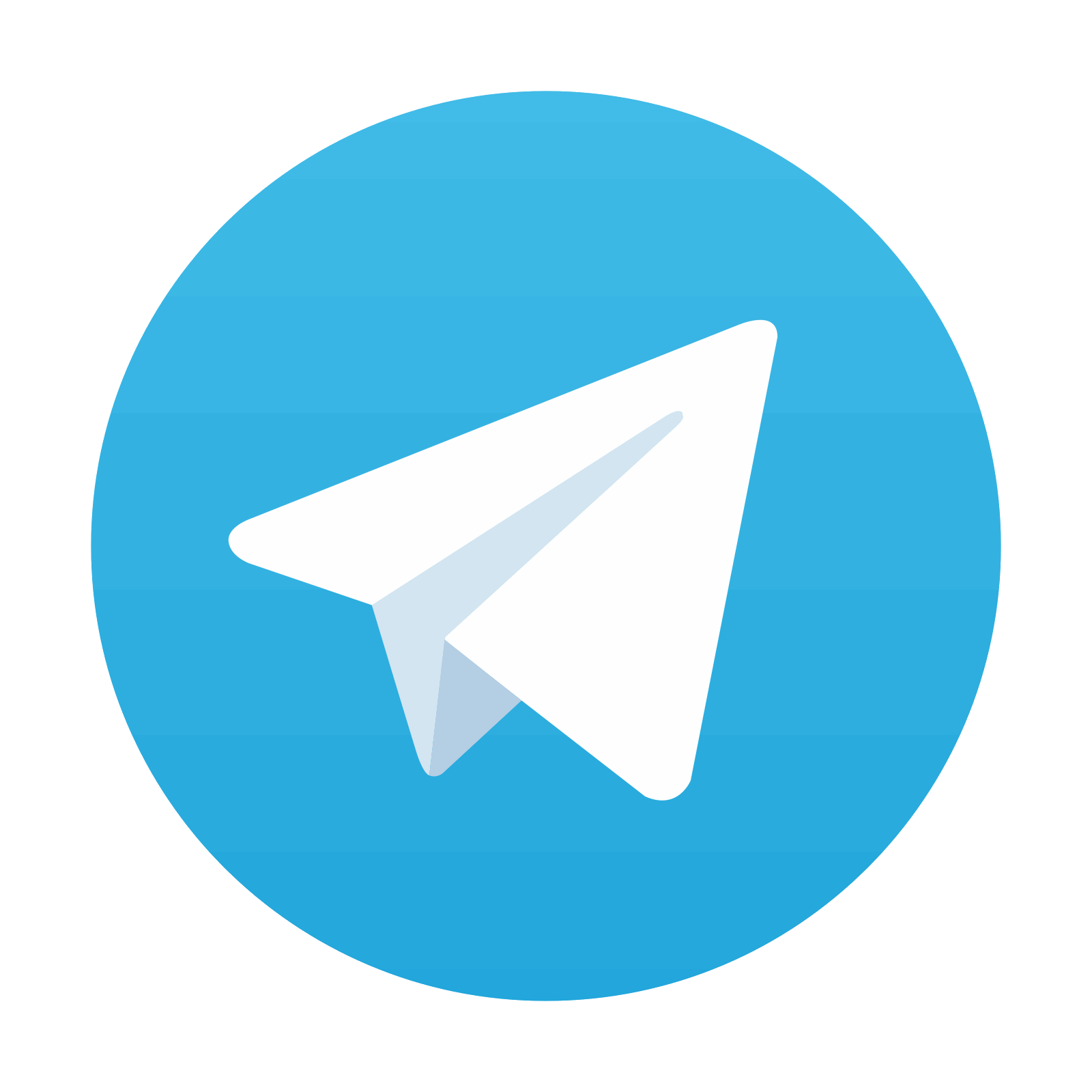
Stay updated, free articles. Join our Telegram channel

Full access? Get Clinical Tree
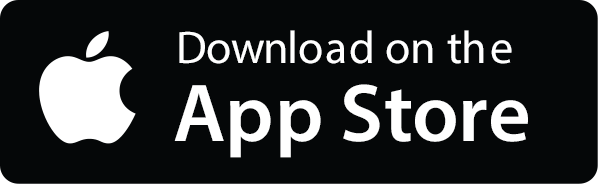
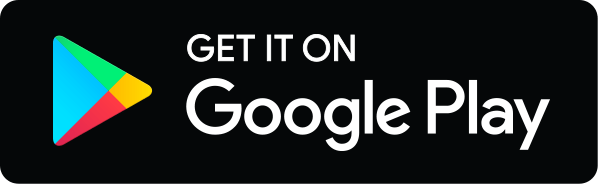