5.1 Introduction
5.1.1 The Complexities and Challenges of Aerosol Performance
Arguably, aerosol drug-delivery systems (inhalers) represent the most complex of medicinal products. Understanding the scientific principles and mechanisms that determine the performance of the aerosol clouds generated is particularly important in their design. It is also critical if intelligent design is to be used in improving their delivery. This chapter introduces some core concepts in aiding this understanding and in providing metrics concerning inhalers’ potential performance and their design space during development. The intention here is not however to describe the standard “in vitro” pharmacopeia end-product quality-control impactor and unit-dose tests (see Chapter 6), but rather to outline the constituent parts, and their role in product performance. We start by looking at the context of the performance challenge itself.
The Challenge Ahead
There is no question that pharmaceutical companies are scientific pioneers; they are developers and operators of leading-edge technologies. Indeed, the 20th century has seen some remarkable developments in medicinal chemistry (see [1] for several good examples), and the 21st century is predicted to yield a new era in medicines, resulting from new biopharmaceutical innovations. Many of these products of biotechnology have the potential to be used in inhaled form using dry powder or liquid aerosols (see for example [2, 3]).
Our understanding of the basic phenomena underlying aerosolization, powder behavior, and particle–particle interactions is growing, and there are many new and exciting process technologies emerging, offering for example new generations of particle constructs, or new engineered device systems. Yet as particle science and technology have advanced, methods used in bulk formulation (especially in powders) processing and manufacturing methods have in many areas been argued to have lagged behind (for example [4]). There is a famous quotation from September 2003, when the Wall Street Journal published a front-page article claiming that pharmaceutical “manufacturing techniques lag far behind those of potato-chip and laundry-soap makers” [5]. Furthermore, in 1996, leading pharma aerosol scientists Richard Dalby and Anthony Hickey stated, “The forces governing dispersion are well documented and consist mainly of electrostatic, van der Waals, and capillary forces. Knowing these forces exist has not facilitated aerosol generation to any extent” [6].
Looking specifically at powder technology, this plays a key role in the development and production of most medicines (not just inhalers). It is a cornerstone of many pharmaceutical development projects, from tablets and capsules, to injectables, oral suspensions, and topical products. The approach to powder technology which runs through so many aspects of current pharmaceutical manufacturing processes has in many cases been left virtually unchanged for decades. This can be said of many of the standard primary and secondary processes involved, such as comminution, blending, agglomeration, and coating. It is certainly true of many processes used to manufacture inhaled powders. Despite a brief period when inhaled insulin (Exubera; see for example [2, 3, 7]) was on the market as a notable exception, being a spray-dried powder, currently there are few significant particulate inhaler products for sale in which the drug is not milled by a micronizer—a traditional process of mechanical destruction, rather than sophisticated particle engineering.
It is highly valid in this chapter, which explores performance and improvements, to ask why this is so, and what are the prospects for improvement.
First, it could be said that current inhaled medicines for local therapies such as asthma and chronic obstructive pulmonary disease (COPD) generally enjoy such wide therapeutic windows and safety profiles that high efficiency and consistency in performance, while very desirable, have not always been demanded.
Second, this chapter argues that consistent with the Hickey/Dalby view from 1996, there is still generally a poor appreciation of the fundamental processes involved in bulk particle behavior and aerosolization processes. Powder/particle technology is not just complicated, it is a highly complex multifactorial phenomenon. It is seen by many as a skilled art, reflecting the often poor understanding of the fundamental science behind it. A better recognition of this, and a resulting approach to understanding, is key to improving product performance levels. Many product and process aspects have been left unchanged due to the attitude, “if it ain’t broke, don’t fix it.”
One factor which has allowed powder technology to slip behind progress made in other scientific disciplines in the pharma field appears to be the traditional/historical structure of academia. Powder/particle technology is a multidisciplinary area, as well as a most complex and challenging applied discipline. Consequently, it has too often fallen between the traditional disciplines taught and driven by academic centers. Use of the available technical knowledge requires a cross-disciplinary approach, and the ability and willingness to cross boundaries.
Where specialist subjects have existed more strongly—and colloid science is perhaps the best example of this in our context—a number of problems exist. Many colloid experts will correctly argue that particle–particle interfaces, interactions, and forces are now well understood and can be readily modeled and explained. However, this understanding has generally been developed using idealized model systems, including near-perfect hard spheres of idealized materials such as silica. Real inhalers contain real drug and excipient particles, which often have an apparently infinite range of surface variations (morphologies, chemical groups, structural order, etc.). Recognizing this is critical in appreciating the challenge ahead for the design and prediction of aerosol inhaler performance.
Third, there is a resistance to change in manufacturing. The regulatory environment has historically led to a largely empirical demonstration of conformity to specification and consistency for a series of production batches. While this has generally been effective, it is not especially technically demanding. One can also argue that in consequence of these effects, the refinement and uptake of new particle or device designs and new processing methods has been slow. Perhaps this will change in the near future with current initiatives such as Process Analytical Technology (PAT) and Quality by Design (QbD) [8].
5.1.2 Understanding Powder/Particle Characteristics: Implications for Aerosol Product Performance
So, we know that formulating a drug for practical, efficient, and reproducible pulmonary delivery as particles from a device into a patient is a major challenge. At the core of the problem is the inherent cohesion of drug particles that are physically small enough (aerodynamic diameter of about 1–6 μm or even smaller for systemic delivery) to be efficiently transported as an aerosol into the lung with an inspiratory airflow, especially when they are administrated as a powder form from dry powder inhalers (DPIs). Added to this challenge is the “real-world” variation of each and every new material (as noted in Section 5.1.1).
Considering the entire aerosolization process of a powder from an inhaler device during inhalation, it is logical to view it as two critical events. First, the powder should be resuspended and carried from an inhaler device by airflow, termed “entrainment.” Second, the powder should be deagglomerated into fine particle form during the aerosolization, often termed “dispersion” or “deagglomeration.” The external factors, such as airflow rate and inhaler design (see for example discussions [9–11] and references therein), have been show to be major influences. However, it is important to recognize that not only is our fundamental understanding of aerosolization and real particle interactions limited, but studies on the effect of intrinsic fine-particle cohesion on aerosol performance are not common and are rarely even close to being able to explain observed variation, let alone predict it. Such cohesion confers poor flowability, poor fluidization, and poor dispersion characteristics on powders, which are unable to provide efficient and consistent drug-delivery performance.
Consider the most commonly adopted approach to this issue for DPIs: creation of a homogeneous interactive mixture of fine drug particles and coarse carrier particles for powder inhalers. This interactive-mixture approach has arisen largely through experience, as an empirical solution. The coarse carrier is used to enable the formulation to flow, and powder flowability is achieved in order to meet manufacturing demands, ensuring dose accuracy and dose uniformity, and to enable the formulation to fluidize and be entrained from the inhaler device. However, the drug-delivery efficiency of these mixtures is generally low, since most drug particles are unable to detach from carrier surfaces during the aerosolization phase, due to the strong adhesion between drug and carrier particles [12, 13].
Further, a ternary DPI-system approach has been empirically evolved by adding fine additives (in most cases, finely milled lactose powders) into drug–coarse carrier mixtures, leading to an improved drug-delivery performance [14]. Several theories have been proposed which try to explain the mechanism of this ternary DPI system. One is that fine additives occupy the active binding sites on the carrier surfaces and thus reduce the adhesion between drug and carrier particles; another is that the formation of agglomerates of drug and fine additive particles can reduce the effective adhesion between drug and carrier particles, as well as reduce the agglomerate strength. However, the true mechanism is far from clear and is undoubtedly non-trivial (see for example [14, 15]).
5.1.3 Liquid Systems
One can argue that the basic processes of liquid atomization are much better understood, especially in terms of control, than those of powder dispersion. This understanding of spray processes results from the significant effort invested in developing and predicting liquid-spray behavior in fields varying from fuel injection to instrument design, from printers to spray dryers [16]. The fruit of this knowledge is a growing number of new, standalone, liquid-atomizing inhaler devices.
The aerosol science of droplet atomization and transport still provides highly complex challenges. With different atomization mechanisms, parameters such as surface tension and viscosity vary in influence, but perhaps of greater concern are the differences between the behaviors of solutions and suspensions. As with powders, the interparticle interactions in colloidal suspensions are a prime source of uncertainty in performance. The recent emergence of nanosuspensions during atomization—and their variations—also has important implications for behavior.
Once produced, an aqueous liquid aerosol is an inherently unstable beast, in which phenomena such as evaporation and coalescence feature. Such phenomena should be considered in designing any inhaler.
Despite this better understanding and control of liquid aerosolization, liquid-based inhalers are generally on the decline. The reasons for this are many and complex, but include company marketing strategies, the outcome of Montreal Protocol-driven changes to propellant use, the limit on dose ranges possible in liquids, and the inherently lower chemical stability of many molecules in solution rather than in dry powder forms.
5.1.4 Summary
The purpose of this introductory section has been to identify the many challenges facing the product development of an inhaled form: a challenge often underestimated by new entrants into the field, usually at their peril. It can be argued from the examples in this section that advances are not obviously led by a sophisticated understanding of fundamentals. However, the limitations of our knowledge should be qualified by the fact that many highly effective, well-produced, and commercially very successful products clearly exist. Consequently, we have found ways in which these issues can be addressed. Many robust techniques and approaches are available, and these are evolving to characterize specific physical properties of the formulation components, the process methods, the devices and their surfaces, the geometry and hydraulic fluid properties, and of course the nature and behavior of the finished product.
In the rest of this chapter, we will briefly introduce a number of the current main approaches used to characterize specific physical and chemical properties of a formulation. With new analytical technologies appearing regularly, this area is continually evolving. Properties of interest include particle and aerosol size distributions, particle forms, amorphous/crystalline content, surface characteristics, and interparticulate cohesion forces. In addition, we shall introduce some material and process strategies for performance improvement.
5.2 Particle Sizing
The key parameter for efficient delivery is aerodynamic particle size. The background to particle size distributions, moments, and representations, and their implications, is detailed elsewhere, but the reader should keep at the forefront of their mind the fundamental importance of Stokes’ law, and especially the influence of aerosol aerodynamic diameter [17].
No single particle-sizing technique is suitable for all tasks. The particle-sizing approach that is to be used at any stage needs to be selected with care and attention if we are to understand the desired outcome and the nature of the material. The basic techniques will be introduced here in outline only, as a good number of excellent and comprehensive texts exist in this area [18–21].
5.2.1 Sieve Analysis
For larger particles, notably carrier particles used in DPI formulations, sieve analysis is often used. The powder is introduced on to a stack of analytical sieves, which are arranged vertically in order, with the largest aperture sieve at the top, then the next largest, and so on. The stack is then mechanically vibrated, in order to encourage the powders to be exposed to each aperture: particles that can geometrically fall through the mesh will continue to descend until they are trapped on the stage below the smallest aperture they can pass through. The powders are vibrated for a validated time, beyond which further changes are minimal. The powder masses retained on each stage are then determined.
In practice, particle sizing with a sieve is limited by the ability to deagglomerate particles, so sieving below approximately 50 μm is often impractical because the cohesive forces are greater than the gravimetric detachment forces. Wet-sieving or air-jet-sieving can in some cases allow smaller particles to be effectively sieved.
5.2.2 Image Analysis
Particle size (and form) may be assessed visually under a microscope. The microscope method involves preparing suitable particle dispersion for inspection, where preferably all particles are separated and can be independently distinguished, and then comparing the projected 2D areas of a large number of irregularly shaped particles to a standard graticule or series of circles of known diameter.
Determination requires considerable operator skill and is tedious. However, it is important to note that this is the best approach discussed here for attaining information about particle shape. While only two dimensions of the particle can be seen at any one time, the shape information allows detection of particle habit and general morphological issues, and of aggregation.
Optical-microscope methods are limited by the microscope’s resolving power, and hence are practical only for particles in the order of tens of microns. For micron- to submicron-sized particles, an electron microscope can be used.
Computerized image analysis can be used to automate and overcome some of the operator issues. However, this approach requires software that is intelligently defined and can decide, for example, where the edges of each particle are and make assumptions about the particle thickness.
Computerized microscope optical image analysis can provide a host of shape-related data, as indicated here for lactose samples, where for example circularity, elongation, convexity, and so on can be measured (Figure 5.1).
Figure 5.1 Lactose samples imaged using the Malvern Instruments Morphology G3 system. Image courtesy of Monash University-using Morphology G3 instrument
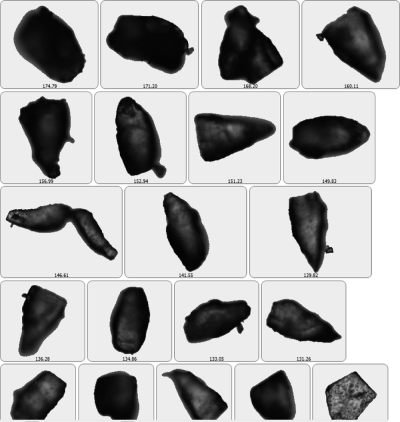
5.2.3 Light Scatter
Light-scattering techniques are currently the most popular systems in use for particle sizing of micronized drug particles, fine or larger excipients, and composite formulated systems. These systems function by measuring the quantity of light scattered by individual particles as they pass through a beam of intense light (often a laser). Instruments may use principles of Fraunhofer diffraction and Mie theory (or Doppler velocimetry) in order to derive particle size information from the scattered light. In each case, the manner in which light is scattered is a function of particle size, and detectors are used to monitor this scatter and convert these signals, using mathematical models, into size distributions.
However, experienced operators warn of many false or erroneous results that can occur if the system is treated as a “black box.” Sample preparation in such particle-sizing methods is perhaps the main cause of false results. These systems will not provide a true particle size distribution unless great care has been taken to validate sample preparation and ensure all primary particles have been deagglomerated (but without milling and size reduction) and formed into a stable, unchanging suspension of a suitable concentration for the period of measurement. Measurement samples may be in liquids or in air/gas.
Particle sizes are then derived using complex mathematical processes. While it is not essential to understand the theories and algorithms, data fit, and modeling used, it is important to appreciate that these data-processing steps include significant levels of assumption and model-fitting activities. Poor signal or material data, such as refractive indices, can yield false data. For example, the suspension medium can provide problems if the refractive indices of sample and medium are not considered in the context of the specific algorithms used to determine size distributions. Considering particle size distributions, it is also important to remember that spherical equivalent diameters are normally quoted; particle shape can differ widely from this in reality. Similarly, these techniques often provide volume equivalent diameter rather than aerodynamic diameter—all of which must be appreciated by the user in data interpretation.
5.2.4 Time-of-Flight
Aerodynamic size can be measured in real time by a time-of-flight aerosol sizer, where particles are separated and accelerated through a well-defined nozzle and their flight between two portions of a split laser beam is timed. The flight time can be directly used to calculate an aerodynamic equivalent diameter. Again, key limitations of this light scatter-based real-time instrument include the sample preparation, interpretation/algorithm issues, and presentation/representation of the data.
5.2.5 Other Methods
Many other methods exist in the arena of particle sizing. Sedimentation has arguably become less popular in recent years, probably due to the advent of modern computer-controlled light-scatter instruments. Similarly, the Coulter-counter method and related electrical sensing methods have become less popular. Despite this apparent fall in popularity, all these methods are useful and may find niche value in specific requirements.
Apart from traditional impactors and impingers familiar to pharmaceutical aerosol science, various other inertial separation methods are available, such as the electrical low-pressure impactor (ELPI), the Stöber centrifuge, and cyclone trains. Again, while less common, these methods can certainly offer some unique benefits.
For submicron particles—a size range not often of interest to inhaler systems—a number of specialist methods are available, from condensation nuclei counters to photocorrelation spectroscopy (e.g. the Zetasizer from Malvern Instruments, Malvern, UK), and including recent alternatives such as NanoSight technology (NanoSight Ltd, Wiltshire, UK).
Shape measurement has been an area of continuing interest, and instruments have used both image analysis (such as the Morphologi G3; Malvern Instruments, Malvern, UK) and changes in light scatter pattern (e.g. ASPECT from Biral Ltd, Bristol, UK).
In practice, particle sizing conducted by pharmaceutical scientists developing inhaled products tends to be limited to a small number of the most common techniques, using direct visual measurement or indirect derivation measurement. Preferred methods are listed in Table 5.1.
Table 5.1 Preferred methods of particle sizing.
Size range | Direct measurement | Indirect measurement |
Carrier particles above ~50 μm | Optical microscopy | Laser diffraction Time of flight Sieve analysis |
Carrier particle fines below ~50 μm | Electron or optical microscopy | Laser diffraction Time of flight |
Drug agglomerates/pellets above ~100 μm | Optical microscopy | Laser diffraction |
Micronized drug ~0.1–10 μm | Electron microscopy | Laser diffraction Time of flight |
Nanomilled drug ~0.01–1 μm | Electron microscopy | Photocorrelation spectroscopy |
5.3 Powder and Particulate Characterization Systems
5.3.1 Introduction
The physicochemical issues influencing powder product performance have been outlined in other chapters and illustrated in the introductory section of this chapter, including (but not limited to):
- How bulk powder and individual particles behave during primary and secondary processes in manufacture.
- How practical it is to meter the device: including flow and segregation.
- Behavior in storage and transport, including chemical and physical stability.
- Performance in the device: including flow and deagglomeration.
Other physicochemical factors also have significance for inhalation, such as the taste of a deposited powder and other sensations stimulated, such as cough. Related to this, the dissolution rate is also an issue, at specific points in the respiratory tract for example, and despite arguably receiving little attention, has implications for drug bioavailability.
Consequently, intrinsic powder physicochemical properties provide the basis for product performance. These properties include: size distribution, surface area, crystallinity, purity, moisture, electrostatic charge, shape factors, surface morphology and chemistry, and density. Size distribution has been treated in its own right in Section 5.2. This section looks primarily at interfacial issues.
5.3.2 Powder Cohesion and Adhesion
The primary issue under consideration here is powder cohesion. Most inhaler powder formulations contain both drug and excipient particles, and hence we are concerned with both “cohesive” (drug–drug) interactions and “adhesive” (drug–excipient) interactions. While excipient–excipient “cohesive” interactions may be important too, focus tends to be on interactions directly concerning drug. The bulk behavior of powder mixtures is a complex function of the adhesive and cohesive forces present.
It is important to consider the nature of such forces. These include the ubiquitous van der Waals forces, as well as possible influences from electrostatic and capillary forces. Several textbooks provide a good fundamental background to this area [12, 13].
The forces of adhesion and cohesion experienced by the drug particles should not be too strong, as the powder may flow too poorly to be mixed, in order to be transported and filled during manufacture, and may not be released from the device, or else drug particles may not detach during inhalation. If such forces are too weak, powders may segregate at critical stages, resulting in unacceptable variation in dosing. In addition, it is possible that too-weak forces may also be detrimental to aerosol quality in some poorly matched systems [22].
So, we need to be aware of how the powder physicochemical properties may influence powder cohesion/adhesion, and how we can assess these properties.
Particle size is very important: as particle size decreases, so surface area and surface energy increase. In simple terms, the greater the area of contact between two surfaces, the stronger the interactions. Particle size analysis was discussed in Section 5.2. However, it is important to note that a mean particle size value alone may not tell the complete story, as particle size distribution and shape factors can also play a major part in powder cohesion/adhesion. For example, two powders with similar measured mean sizes may change in cohesion with spread of the size distribution. Particle shape is often ignored when size distributions are measured and, for example, tabular or plate-shaped particles or needles may flow less well than more anisotropic particles, due to differences in the alignment of adjacent particles, packing efficiency, and the area of contact points (Figure 5.2).
Figure 5.2 Tabular and needle particles of milled salbutamol sulfate (left) pack together differently to spray-dried particles containing salbutamol sulfate of a similar size (right). Image courtesy of Monash University-using Morphology G3 instrument
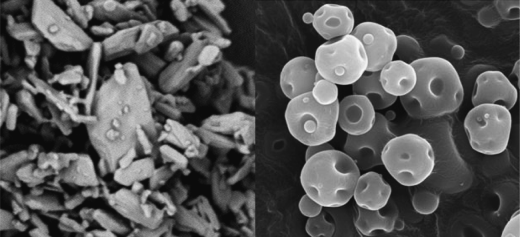
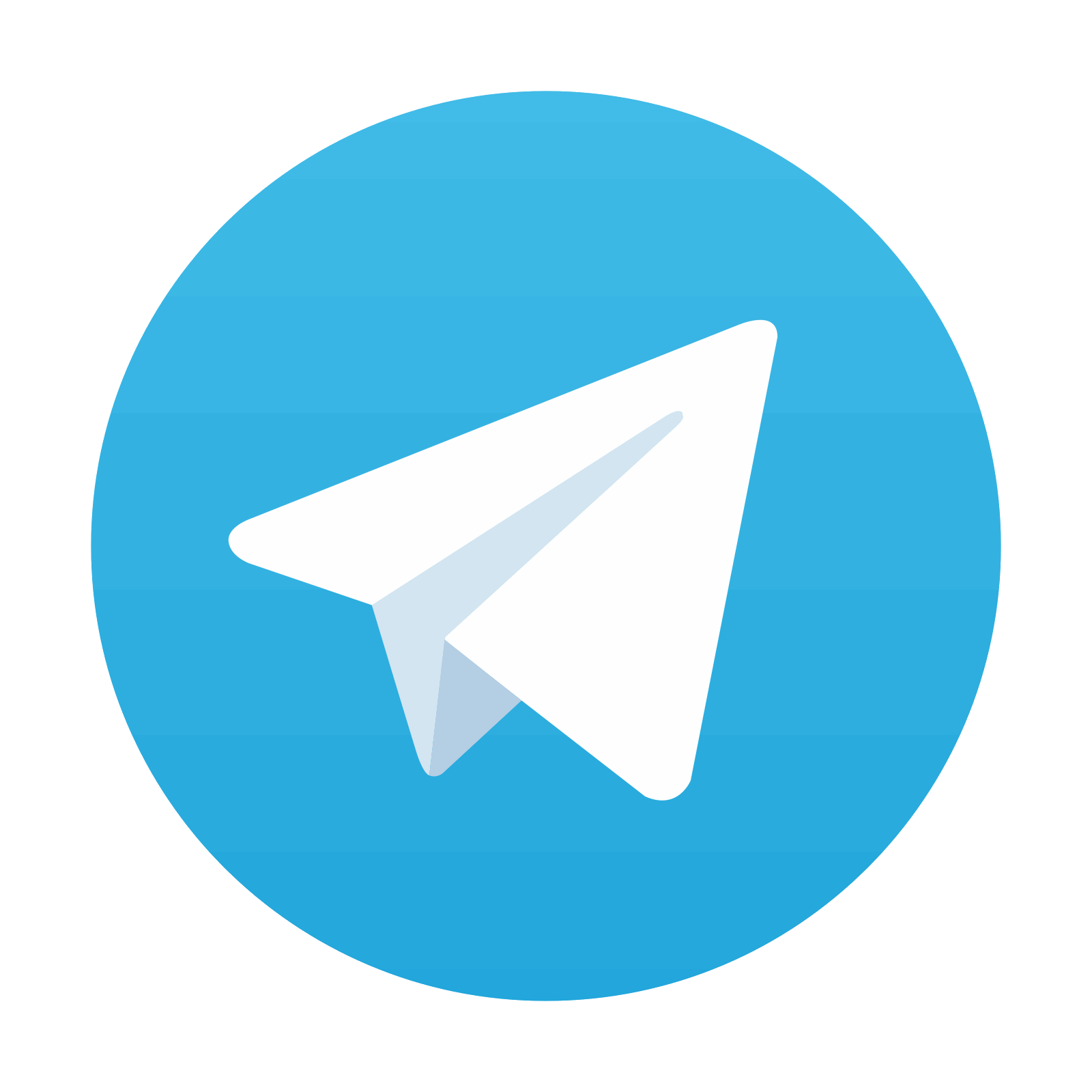
Stay updated, free articles. Join our Telegram channel

Full access? Get Clinical Tree
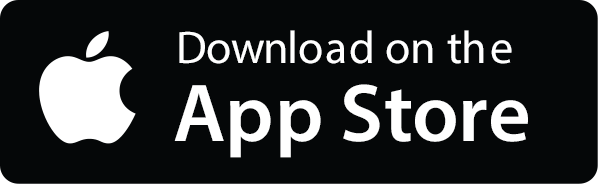
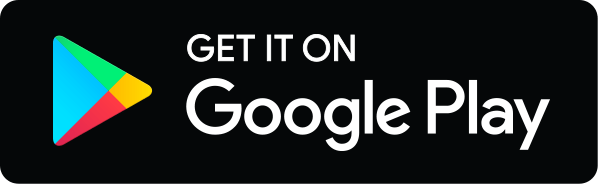