Metabolic Consequences of Calorie Restriction1
Edward P. Weiss
Luigi Fontana
1Abbreviations: ATP, adenosine triphosphate; CR, calorie restriction; GH, growth hormone; IGF, insulinlike growth factor; IL, interleukin; T3, triiodothyronine; TNF-α, tumor necrosis factor-α; TOR, target of rapamycin; UCP, uncoupling protein.
In animal studies, “calorie restriction” (CR) normally refers to a state in which the energy intake in one group of animals is restricted by 30% to 50% less than the levels consumed by a control group of animals that has free access to food. In some studies, food intake in the control group is somewhat limited (e.g., 85% to 95% of the calories of animals fed ad libitum) to avoid comparison of the CR group with control animals that gain excessive body weight and adiposity with age (1). CR in animal studies is typically started very early in life (from shortly after weaning to 6 months of age), and it results in growth retardation. Also implicit in the term is that the diet contains adequate quantities of proteins and micronutrients (e.g., vitamins, minerals), such that malnutrition does not occur.
In humans, CR generally refers to a state in which energy intake is sufficiently low to achieve or maintain a low-normal body weight status (i.e., body mass index <21 kg/m2) without causing malnutrition (i.e., adequate intake of proteins and micronutrients). Research on CR in humans has generally enrolled fully developed, weightstable adults as subjects. In intervention studies, CR inevitably results in a negative energy balance and weight loss, thus making it difficult to differentiate between the effects of CR itself and weight loss. In clinical settings and in the scientific literature, the term “calorie restriction” is often loosely used to describe any reduction in energy intake, even if the basal energy intake is excessive (as is often the case in obesity) and it is being reduced to more normal levels. For the purposes of discussing CR in this chapter, however, the focus is on the effects of CR when applied to normal-weight or slightly overweight individuals and not on the role of CR in treating the pathologic state of obesity.
CALORIE INTAKE, LONGEVITY, AND DISEASE IN LABORATORY ANIMALS
In 1935, investigators demonstrated that the life span of laboratory rats could be increased by approximately 30% by restricting energy intake (i.e., CR) after weaning (2). Since then, hundreds of studies have demonstrated that CR slows aging and increases life span in several model organisms, including rats, mice, dogs, fish, flies, and worms (3, 4). The severity of CR, the age when CR is started, and the strain or genetic background of the animals determine the degree of life span extension.
In many organisms, a monotonic relationship exists between CR and longevity response. For example, in mice and rats, CR of 30% to 50% started after weaning proportionally extends maximal life span (defined as the mean life span of the longest lived 10% of the cohort) by 30% to 50%. CR has also been shown to lengthen maximal life span in mice when it is started in adulthood, but to a lesser extent (5). In rodents, CR increases life span in part by preventing or postponing the occurrence of a wide range of chronic diseases, including cancer (up to 62% reduction in cancer incidence), obesity, type 2 diabetes, and autoimmune, cardiovascular, kidney, and neurodegenerative diseases (6, 7, 8). Data from postmortem pathologic studies demonstrated that 30% of the calorie-restricted rodents died when they were very old without any evidence of lethal disease; this finding suggests that, in mammals, it is biologically possible to live a long life without developing diseases (9). In addition, CR slows the age-dependent deterioration of structure and function in organs and tissues, such that calorie-restricted animals appear to be biologically younger during advancing age.
Ongoing studies at the National Institutes of Health and the University of Wisconsin are evaluating the effect of lifelong CR on aging and life span in nonhuman primates (i.e., rhesus monkeys). At present, the available
data demonstrate that long-term CR results in some of the same metabolic and hormonal adaptations that take place in calorie-restricted rodents, including decreased total body and abdominal fat, increased insulin sensitivity, improved lipid profile, reduced blood pressure, decreased inflammatory and oxidative stress markers, lower serum triiodothyronine (T3) concentration and body temperature, and prevention of the age-associated decline in serum concentrations of dehydroepiandrosterone sulfate and melatonin (8, 10). Moreover, the University of Wisconsin study showed that 20 years of 30% CR started in adult rhesus monkeys reduced cancer and cardiovascular disease incidence and mortality by 50% and completely prevented type 2 diabetes and obesity (11). Additionally, immune senescence, sarcopenia, and brain atrophy in several subcortical regions that control motor and executive function appear to be delayed by CR in monkeys (11, 12, 13). Although investigators do not yet know whether CR extends maximum life span in nonhuman primates, definitive data will likely be available by 2020 or 2025. Nonetheless, these studies show that chronic moderate (30%) CR can be safely maintained in nonhuman primates, and the several age-related phenotypes can be delayed or prevented.
data demonstrate that long-term CR results in some of the same metabolic and hormonal adaptations that take place in calorie-restricted rodents, including decreased total body and abdominal fat, increased insulin sensitivity, improved lipid profile, reduced blood pressure, decreased inflammatory and oxidative stress markers, lower serum triiodothyronine (T3) concentration and body temperature, and prevention of the age-associated decline in serum concentrations of dehydroepiandrosterone sulfate and melatonin (8, 10). Moreover, the University of Wisconsin study showed that 20 years of 30% CR started in adult rhesus monkeys reduced cancer and cardiovascular disease incidence and mortality by 50% and completely prevented type 2 diabetes and obesity (11). Additionally, immune senescence, sarcopenia, and brain atrophy in several subcortical regions that control motor and executive function appear to be delayed by CR in monkeys (11, 12, 13). Although investigators do not yet know whether CR extends maximum life span in nonhuman primates, definitive data will likely be available by 2020 or 2025. Nonetheless, these studies show that chronic moderate (30%) CR can be safely maintained in nonhuman primates, and the several age-related phenotypes can be delayed or prevented.
CALORIE RESTRICTION IN HUMANS
Data from humans who are willing to restrict their energy intake while maintaining adequate micronutrient intakes show that CR provides powerful protective effects against overweight or obesity, type 2 diabetes, oxidative stress, inflammation, and left ventricular diastolic dysfunction, effects similar to those reported in calorie-restricted rodents and monkeys (8, 14, 15, 16, 17, 18). CR in humans markedly reduces several risk factors for cardiovascular disease, including serum concentrations of total and low-density lipoprotein cholesterol, glucose, insulin, inflammatory markers and cytokines, blood pressure, intima media thickness of the carotid arteries, and abdominal obesity, and it causes a large increase in high-density lipoprotein cholesterol concentrations (14). CR also results in some of the same hormonal adaptations related to longevity in calorie-restricted rodents, including lower circulating concentrations of T3, testosterone, and estradiol and increased adiponectin (8, 15, 19, 20). Major differences in the effects of CR exist between rodents and humans, however. In rodents, CR without protein restriction results in a large reduction in the level of insulin-like growth factor-I (IGF-I) (21). In contrast, in humans, CR does not lower IGF-I levels unless protein intake is also reduced (22). Considerable evidence indicates that the reduction in IGF-I plays a key role in mediating both the anticancer and life-extending effects of CR in rodents (3).
Extreme CR in conjunction with malnutrition can lead to numerous unfavorable health effects, such as sarcopenia, osteoporosis, immune dysfunction, anemia, amenorrhea, and infertility (8). Some or all of these adverse effects are possibly related to malnutrition and not to CR itself. Additional clinical studies are needed, however, to evaluate the long-term effects of severe CR with adequate nutrient intake on bone and skeletal muscle metabolism, immune function, and the risk of developing life-threatening infections. Furthermore, more studies are necessary to determine optimums for energy intake (and macronutrient and micronutrient composition) for extending healthy life span, including optimums specific for age, sex, and genetic background.
MECHANISMS FOR THE EFFECTS OF CALORIE RESTRICTION
Substantial progress has been made in evaluating several theories of the way in which CR slows aging and increases life span. It is now becoming clear that many overlapping and mutually dependent metabolic, physiologic, and cellular adaptations to CR itself are responsible for the beneficial effects of CR on health span and life span. The following sections provide an overview of some of the most promising evidence on the mechanisms by which CR affects metabolism, aging, chronic disease, and life span.
Neuroendocrine Systems Adaptations
From an evolutionary perspective, animals and humans have evolved to sense the availability of nutrients and adjust their metabolism as needed to maximize survival. For example, during times of high energy intake, substantial amounts of energy are directed toward anabolic processes, including cell, tissue, and organ growth, as well as growth of the animal as a whole. The larger size of the animal provides a survival advantage by allowing the animal to compete more successfully for food, water, and shelter, to defend itself against predators, and to maximize reproduction. In contrast, during periods of low nutrient availability, cell division and reproduction are arrested or minimized to allow energy to be available to maintenance processes.
Research on simple model organisms and rodents has consistently demonstrated that CR may increase average and maximal life span by decreasing the activity of nutrient-sensing pathways including the IGF, insulin, and target of rapamycin (TOR) pathways (3). For example, animal studies demonstrated that CR results in lower circulating concentrations of IGF-I (21, 23). IGF-I is primarily secreted from the liver into systemic circulation in response to growth hormone (GH) exposure (24). It has potent effects on numerous tissues to stimulate cell growth and proliferation and inhibit programmed cell death (apoptosis) (25). Reductions in IGF-I appear to be important in the life-extending and disease protective effects of CR in laboratory animals (21, 23). This importance is evidenced by the CR-like increase in life span and decrease in cancer seen in rodents with genetic mutations that result in GH deficiency or an absence of IGF-I or
IGF-I receptors (26, 27, 28, 29). In contrast, mice that overexpress the GH receptor have very high concentrations of IGF-I, larger body size, shorter life span, and an increased incidence of cancer and kidney and neurodegenerative disease (30).
IGF-I receptors (26, 27, 28, 29). In contrast, mice that overexpress the GH receptor have very high concentrations of IGF-I, larger body size, shorter life span, and an increased incidence of cancer and kidney and neurodegenerative disease (30).
In addition to alteration in IGF-I signaling, other neuroendocrine alterations appear to contribute to the effect of CR on longevity. The CR-mediated reduction in the activity of the insulin signaling pathway contributes to increases in longevity, because loss-of-function mutations in the insulin receptor in adipose tissue and in insulin receptor substrates 1 and 2 in the brain all promote longevity in mice (31, 32, 33). In both humans and animals, CR reduces circulating levels of hormones that regulate energy homeostasis, cell respiration, and tissue growth, such as the thyroid hormones (34). In particular, CR causes a selective reduction in T3. This change is likely related to CR itself rather than to changes in body composition caused by CR, because a comparable decrease in fat mass achieved by exercising does not reduce T3 (19, 35). Given that T3 stimulates metabolism and thermogenesis (34), T3 reductions may lead to slower cellular metabolism, less free radical production, and lower body temperature, all of which could contribute to increases in longevity (19, 34, 36). Although reductions in T3 concentrations, cellular metabolism, and body temperature are attractive from the viewpoint of slowing aging, these effects of CR may predispose men and women to subsequent weight gain (37). Other important hormonal adaptations that may play a role in mediating the antiaging effects of CR are reduced levels of anabolic hormones, such as testosterone and leptin, and increased levels of hormones that suppress inflammation such as cortisol, adiponectin, and ghrelin (6, 7, 8, 10, 15, 20).
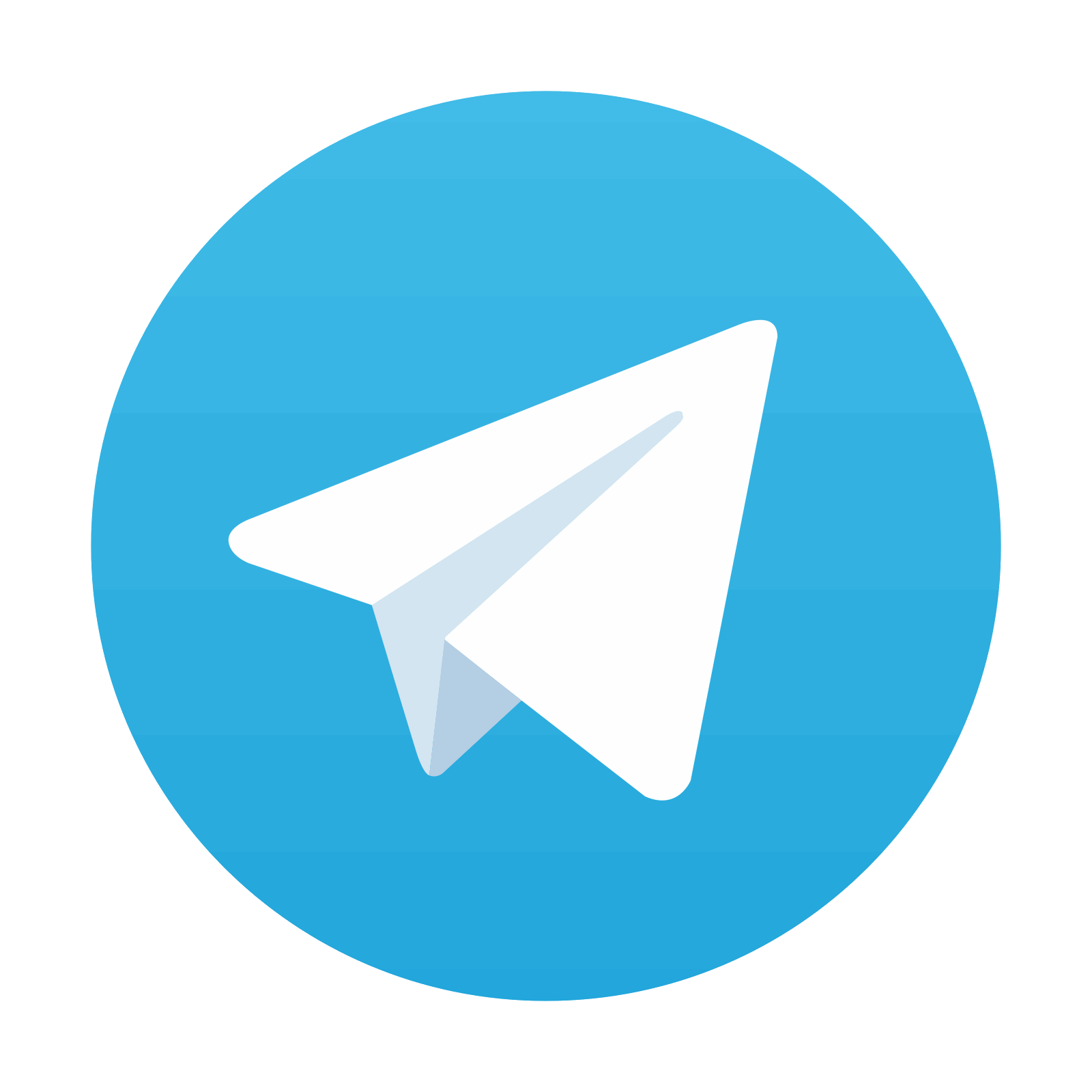
Stay updated, free articles. Join our Telegram channel

Full access? Get Clinical Tree
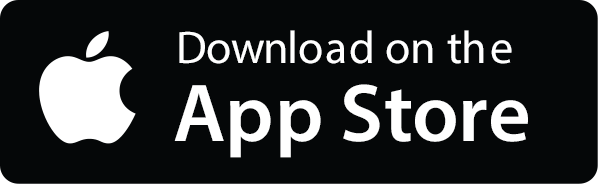
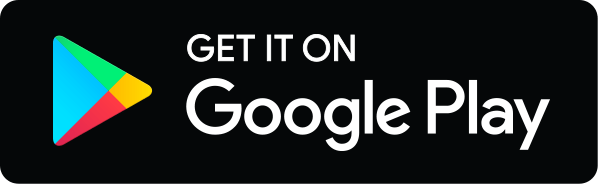