Glycolysis, a series of chemical reactions, converts glucose molecules into pyruvic or lactic acid.
The citric acid cycle removes ionized hydrogen atoms from pyruvic acid and produces carbon dioxide.
Oxidative phosphorylation traps energy from the hydrogen electrons and combines the hydrogen ions and electrons with oxygen to form water and the common form of biological energy, adenosine triphosphate (ATP).
Alpha cells of the islets of Langerhans secrete glucagon, which increases the blood glucose level by stimulating phosphorylase activity to accelerate liver glycogenolysis.
Beta cells of the islets of Langerhans secrete the glucose-regulating hormone insulin, which assists in glucose transport across cell membranes and storage of excess glucose as fat.
The adrenal medulla, as a physiologic response to stress, secretes epinephrine, which stimulates liver and muscle glycogenolysis to increase the blood glucose level.
Corticotropin and glucocorticoids also increase blood glucose levels. Glucocorticoids accelerate gluconeogenesis by promoting the flow of amino acids to the liver, where they’re synthesized into glucose.
Human growth hormone (hGH) limits the fat storage and favors fat catabolism; consequently, it inhibits carbohydrate catabolism and thus raises blood glucose levels.
Thyroid-stimulating hormone and thyroid hormone have mixed effects on carbohydrate metabolism and may raise or lower blood glucose levels.
plasma proteins) and can reproduce, control cell growth, and repair itself. However, when carbohydrates or fats are unavailable as energy sources, or when energy demands are exceedingly high, protein catabolism converts protein into an available energy source. Protein metabolism consists of many processes, including:
Nutrients | Functions |
Carbohydrates | ▪ Energy source |
Fats and essential fatty acids | ▪ Energy source; essential for growth, normal skin, and membranes |
Proteins and amino acids | ▪ Synthesis of all body proteins, growth, and tissue maintenance |
Water-soluble vitamins: | |
▪ Ascorbic acid (C) ▪ Thiamine (B1) ▪ Riboflavin (B2) ▪ Niacin ▪ Vitamin B12 ▪ Folic acid | ▪ Collagen synthesis, wound healing, antioxidation ▪ Coenzyme in carbohydrate metabolism ▪ Coenzyme in energy metabolism ▪ Coenzyme in carbohydrate, fat, energy metabolism, and tissue metabolism ▪ Deoxyribonucleic acid (DNA) and ribonucleic acid synthesis; erythrocyte formation. ▪ Coenzyme in amino acid metabolism; heme and hemoglobin formation; DNA synthesis; lowering homocysteine levels |
Fat-soluble vitamins: | |
▪ Vitamin A ▪ Vitamin D ▪ Vitamin E ▪ Vitamin K | ▪ Vision in dim light, mucosal epithelium integrity, tooth development, endocrine function ▪ Regulation of calcium and phosphate absorption and metabolism; renal phosphate clearance; musculoskeletal function ▪ Antioxidation; essential for muscle, liver, and red blood cell integrity ▪ Blood clotting (catalyzes synthesis of prothrombin by liver); skeletal function |
Deamination—a catabolic and energy-producing process occurring in the liver with the splitting off of the amino acid to form ammonia and a keto acid
Transamination—anabolic conversion of keto acids to amino acids
Urea formation—a catabolic process occurring in the liver, producing urea, the end product of protein catabolism
and other substances needed for cellular function. The many components of body fluids have the important function of preserving osmotic pressure and acid-base and anion-cation balance.

throughout the body. (See Laboratory tests: assessing nutritional status.) Results of such laboratory tests, of course, supplement the information obtained from dietary history and physical examination—which offer gross clinical information about the quality, quantity, and efficiency of metabolic processes. To support clinical information, anthropometry, heightweight ratio, and skin-fold thickness determinations specifically define tissue nutritional status.
Obtain a complete dietary history and nutritional assessment, including weight history and GI symptoms, to determine if carbohydrate, fat, protein, vitamin, mineral, and water intake are adequate for energy production and for tissue repair and growth. Remember that during periods of rapid tissue synthesis (growth, pregnancy, healing), protein needs increase.
Consult a dietitian about any patient who may be malnourished because of malabsorption syndromes, renal or hepatic disease, or clearliquid diets or who may possibly receive nothing by mouth for more than 5 days. Planned meals that provide adequate carbohydrates, fats, and protein are necessary for convalescence. Supplementary carbohydrates are often needed to spare protein and achieve a positive nitrogen balance.
Accurately record intake and output to assess fluid balance (this includes intake of oral liquids or I.V. solutions and urine, gastric, and stool output).
Weigh the patient daily—at the same time, with the same-type clothing, and on the same scale. Remember, a weight loss of 2.2 lb (1 kg) is equivalent to the loss of 1 L of fluid.
Observe the patient closely for insensible water or unmeasured fluid losses (such as through diaphoresis). Remember, fluid loss from the skin and lungs (normally 900 ml/day) can reach as high as 2,000 ml/day from hyperventilation or tachypnea, thus increasing insensible water losses.

Recognize I.V. solutions that are hypoosmolar, such as 0.45% NaCl (half-normal saline solution). Iso-osmolar solutions include normal saline solution (0.9% NaCl), 5% dextrose in 0.2% NaCl, Ringer’s solutions, and 5% dextrose in water. (The latter acts like a hypotonic solution because dextrose is quickly metabolized, leaving only free water.) Hyperosmolar solutions include 5% dextrose in normal saline solution, 10% dextrose in water, and 5% dextrose in Ringer’s lactate solution.
When continuously administering hypoosmolar solutions, watch for signs of water intoxication: headaches, behavior changes (confusion or disorientation), nausea, vomiting, rising blood pressure, and falling pulse rate.
When continuously administering hyperosmolar solutions, be alert for signs of hypovolemia: thirst, dry mucous membranes, slightly falling blood pressure, rising pulse rate and respirations, low-grade fever (99° F [37.2° C]), and elevated hematocrit, hemoglobin, and BUN levels.
Administer fluid cautiously, especially to the patient with cardiopulmonary or renal disease, and watch for signs of overhydration: constant and irritating cough, dyspnea, moist crackles, rising central venous pressure, and pitting edema (late sign). When the patient is in an upright position, neck and hand vein engorgement is a sign of fluid overload.

malabsorption due to celiac disease, sprue, cirrhosis, obstructive jaundice, cystic fibrosis, giardiasis, or habitual use of mineral oil as a laxative
massive urinary excretion caused by cancer, tuberculosis, pneumonia, nephritis, or urinary tract infection
decreased storage and transport of vitamin A due to hepatic disease.
Corneal damage
failure to thrive and apathy, along with dry skin and corneal changes, which can lead to ulceration and rapid destruction of the cornea.

Administer oral vitamin A supplements with or after meals or parenterally, as indicated. Watch for signs of hypercarotenemia (orange coloration of the skin and eyes) and hypervitaminosis A (rash, hair loss, anorexia, transient hydrocephalus, and vomiting in children; bone pain, hepatosplenomegaly, diplopia, and irritability in adults). If these signs occur, discontinue supplements and notify the physician immediately. (Hypercarotenemia is relatively harmless; hypervitaminosis A may be toxic.)
Recommended daily allowance of B-complex vitamins | |||||||||||||||||||||||||||||||||||
---|---|---|---|---|---|---|---|---|---|---|---|---|---|---|---|---|---|---|---|---|---|---|---|---|---|---|---|---|---|---|---|---|---|---|---|
|

on diets of unenriched rice and wheat. Although this disease is uncommon in the United States, alcoholics may develop cardiac (wet) beriberi with high-output heart failure, neuropathy, and cerebral disturbances. In times of stress (e.g., pregnancy), malnourished young adults may develop beriberi; infantile beriberi may appear in infants on low-protein diets or in those breastfed by thiamine-deficient mothers.
a “3-D” syndrome—dementia, dermatitis, and diarrhea. If not reversed by therapeutic doses of niacin, pellagra can be fatal.
Thiamine deficiency—commonly measured as micrograms per deciliter in a 24-hour urine collection. Deficiency levels are age-related: 1 to 3 years, less than 120; 4 to 6 years, less than 85; 7 to 9 years, less than 70; 10 to 12 years, less than 60; 13 to 15 years, less than 50; adults, less than 27; pregnant women, less than 23 (second trimester), less than 21 (third trimester).
Riboflavin deficiency—measured as micrograms per gram of creatinine in a 24-hour urine collection. Deficiency levels are age-related: 1 to 3 years, less than 150; 4 to 6 years, less than 100; 7 to 9 years, less than 85; 10 to 15 years, less than 70; adults, less than 27; pregnant women, less than 39 (second trimester), less than 30 (third trimester).
Niacin deficiency—measured by N-methyl nicotinamide in a 24-hour urine collection as micrograms per gram of creatinine. Deficiency levels in adults are less than 0.5; in pregnant women, less than 0.5 (first trimester), less than 0.6 (second trimester), and less than 0.8 (third trimester).
Pyridoxine deficiency—xanthurenic acid more than 50 mg/day in 24-hour urine collection after administration of 10 g of L-tryptophan; decreased levels of serum and red blood cell transaminases; reduced excretion of pyridoxic acid in urine.
Cobalamin deficiency—cobalamin serum levels less than 170 pg/ml. Tests to discover the deficiency’s cause include gastric analysis and hemoglobin studies. In addition, the Schilling test measures absorption of radioactive cobalamin with and without intrinsic factor; however, it is rarely used.
Thiamine deficiency—a high-protein diet, with adequate calorie intake, possibly supplemented by B-complex vitamins for early symptoms. Thiamine-rich foods include pork, peas, wheat bran, oatmeal, and liver. Alcoholic beriberi may require thiamine supplements or thiamine hydrochloride as part of a B-complex concentrate.
Riboflavin deficiency—supplemental riboflavin in patients with intractable diarrhea or increased need for riboflavin related to growth, pregnancy, lactation, or wound healing. Good sources of riboflavin are meats, enriched flour, milk and dairy products eggs, cereal, and green, leafy vegetables. Acute riboflavin deficiency requires daily oral doses of riboflavin alone or with other B-complex vitamins. Riboflavin phosphate can also be administered I.V. or I.M.
Niacin deficiency— supplemental B-complex vitamins and dietary enrichment in patients at risk because of marginal diets or alcoholism. Meats, fish, peanuts, brewer’s yeast, enriched breads, and cereals are rich in niacin; milk and eggs, in tryptophan. Confirmed niacin deficiency requires daily doses of niacinamide orally or I.V.
Pyridoxine deficiency—prophylactic pyridoxine therapy in infants and in children with a seizure disorder; supplemental B-complex vitamins in patients with anorexia, malabsorption, or those taking isoniazid or penicillamine. Some women who take hormonal contraceptives may have to supplement their diets with pyridoxine. Confirmed pyridoxine deficiencies require oral or parenteral pyridoxine. Children with seizures stemming from metabolic dysfunction may require daily doses of 200 to 600 mg pyridoxine.
Cobalamin deficiency—parenteral cobalamin in patients with reduced gastric secretion of hydrochloric acid, lack of intrinsic factor, some malabsorption syndromes, or ileum resections. Strict vegetarians may have to supplement their diets with oral vitamin B12. Depending on the deficiency’s severity, supplementary cyanocobalamin or methylcobalamin is usually given parenterally for 5 to 10 days, followed by monthly or daily vitamin B12 supplements.
Identify and observe patients who are at risk for vitamin B deficiencies—alcoholics, the elderly, pregnant women, oral hormonal contraceptive users (vitamins B6 and B12), and people on limited diets.
Administer prescribed supplements. Make sure patients understand how important it is that they adhere strictly to their prescribed treatment for the rest of their lives. Watch
for adverse effects from large doses of niacinamide, such as a flushed sensation or hot flashes, in patients with niacin deficiency. Remember, prolonged intake of niacin can cause hepatic dysfunction. Caution patients with Parkinson’s disease receiving pyridoxine that this drug can impair response to levodopa therapy.
Explain all tests and procedures. Reassure patients that, with treatment, the prognosis is good. Refer patients to appropriate assistance agencies if their diets are inadequate due to adverse socioeconomic conditions.

Encourage the patient to follow a wellbalanced diet.
Vitamin B12 injections can prevent anemia after surgeries known to cause vitamin B12 deficiency.
destruction of vitamin C in foods by overexposure to air or by overcooking
excessive ingestion of vitamin C during pregnancy, which causes the neonate to require large amounts of the vitamin after birth
marginal intake of vitamin C during periods of physiologic stress—caused by infectious disease, for example—which can deplete tissue saturation of vitamin C
Scurvy

Administer ascorbic acid orally or by slow I.V. infusion, as indicated. Avoid moving the patient unnecessarily to avoid irritating painful joints and muscles. Encourage him to drink orange juice.
Explain the importance of supplemental ascorbic acid. Counsel the patient and his family about good dietary sources of vitamin C.
Advise against taking too much vitamin C. Explain that excessive doses of ascorbic acid may cause nausea, diarrhea, and renal calculi formation and may also interfere with anticoagulant therapy.

vitamin D-resistant rickets (refractory rickets, familial hypophosphatemia) from an inherited impairment of renal tubular reabsorption of phosphate (from vitamin D insensitivity)
conditions that lower absorption of fatsoluble vitamin D, such as chronic pancreatitis, celiac disease, Crohn’s disease, cystic fibrosis, gastric or small-bowel resections, fistulas, colitis, and biliary obstruction
hepatic or renal disease, which interferes with the formation of hydroxylated calciferol, necessary to initiate the formation of a calciumbinding protein in intestinal absorption sites
malfunctioning parathyroid gland (decreased secretion of parathyroid hormone), which contributes to calcium deficiency (normally, vitamin D controls calcium and phosphorus absorption through the intestine) and interferes with activation of vitamin D in the kidneys
Chronic skeletal pain
Skeletal deformities
Skeletal fractures

Obtain a dietary history to assess the patient’s current vitamin D intake. Encourage him to eat foods high in vitamin D—fortified milk, fish liver oils, herring, liver, and egg yolks—and get sufficient sun exposure. If deficiency is due to socioeconomic conditions, refer the patient to appropriate community agencies.
If the patient must take vitamin D for a prolonged period, tell him to watch for signs of vitamin D toxicity (headache, nausea, constipation and, after prolonged use, renal calculi).

Administer supplementary aqueous preparations of vitamin D for chronic fat malabsorption, hydroxylated cholecalciferol for refractory rickets, and supplemental vitamin D for breast-fed infants.
Consider genetic counseling for a patient with a family history of inherited disorders that can cause rickets.
oxidation of polyunsaturated fatty acids and other lipids. It protects body tissue from damage caused by unstable substances called free radicals, which can harm cells, tissues, and organs and are believed to be one of the causes of aging’s degenerative process. Vitamin E is also important in the formation of red blood cells (RBCs) and helps the body to use vitamin K. Vitamin E deficiency usually manifests as hemolytic anemia in low-birth-weight or premature neonates. With treatment, prognosis is good.
Hemolytic anemia

As indicated, prevent deficiency by providing vitamin E supplements for low-birth-weight neonates receiving formulas not fortified with vitamin E and for adults with vitamin E malabsorption. Many commercial multivitamin supplements are easily absorbed by patients with vitamin E malabsorption.
If vitamin E deficiency is related to socioeconomic conditions, refer the patient to appropriate community agencies.
Inform new mothers who plan to breastfeed that human milk provides adequate vitamin E.
Encourage adult patients to eat foods high in vitamin E; good sources include vegetable oils (corn, safflower, soybean, cottonseed); whole grains; dark green, leafy vegetables; nuts; and legumes. Tell them that heavy consumption of polyunsaturated fatty acids increases the need for vitamin E.

Administer vitamin K to neonates and patients with fat malabsorption or with prolonged diarrhea from colitis, ileitis, or long-term antibiotic drug therapy.
If the deficiency has a dietary cause, help the patient and his family plan a diet that includes important sources of vitamin K, such as cauliflower, tomatoes, cheese, egg yolks, liver, and green, leafy vegetables.
Warn against self-medication with or overuse of antibiotics, because these drugs destroy the intestinal bacteria needed to generate significant amounts of vitamin K.
Vitamin | Sources | Recommended dietary allowance | Action |
Vitamin A | ▪ Carrots; sweet potatoes; dark green, leafy vegetables; butter; margarine; liver; egg yolk | ▪ Ages 1-13: 1,000 to 2,000 international units ▪ Ages ≥ 14: 2,300 to 3,000 international units ▪ Lactating women: 4,000 to 4,300 international units | ▪ Produces retinal pigment and maintains epithelial tissue |
Vitamin D | ▪ Ultraviolet light; fortified foods (especially milk) | ▪ 600 international units daily | ▪ Promotes absorption and regulates metabolism of calcium and phosphorus |




Keep the patient comfortable, and reassure him that symptoms will subside after he stops taking the vitamin.
Make sure the patient or the parents of a child with these conditions understand that vitamins aren’t innocuous. Explain the hazards associated with excessive vitamin intake. Point out that vitamin A and D requirements can easily be met with a diet containing dark green, leafy vegetables; fruits; and fortified milk or milk products.

Hypothyroidism
Goiter

Administer SSKI preparation in milk or juice to reduce gastric irritation and mask its metallic taste. To prevent tooth discoloration, tell the patient to drink the solution through a straw. Store the solution in a light-resistant container.

Advise pregnant women that severe iodine deficiency may produce cretinism in neonates, and instruct them to watch for early signs of iodine deficiency, such as fatigue, lassitude, weakness, and decreased mental function.

Advise the patient to take zinc supplements with milk or meals to prevent gastric distress and vomiting.

Respiratory difficulties
Hypertension
Cardiovascular disease
Diabetes mellitus
Renal disease
Gallbladder disease
Psychosocial difficulties
Premature death
small intestine. Nutrition counseling before and after these procedures is recommended to enhance safe weight loss, educate the patient about proper diet advancement, and monitor for nutritional deficiencies. Psychological and social support are also beneficial before and after surgery.
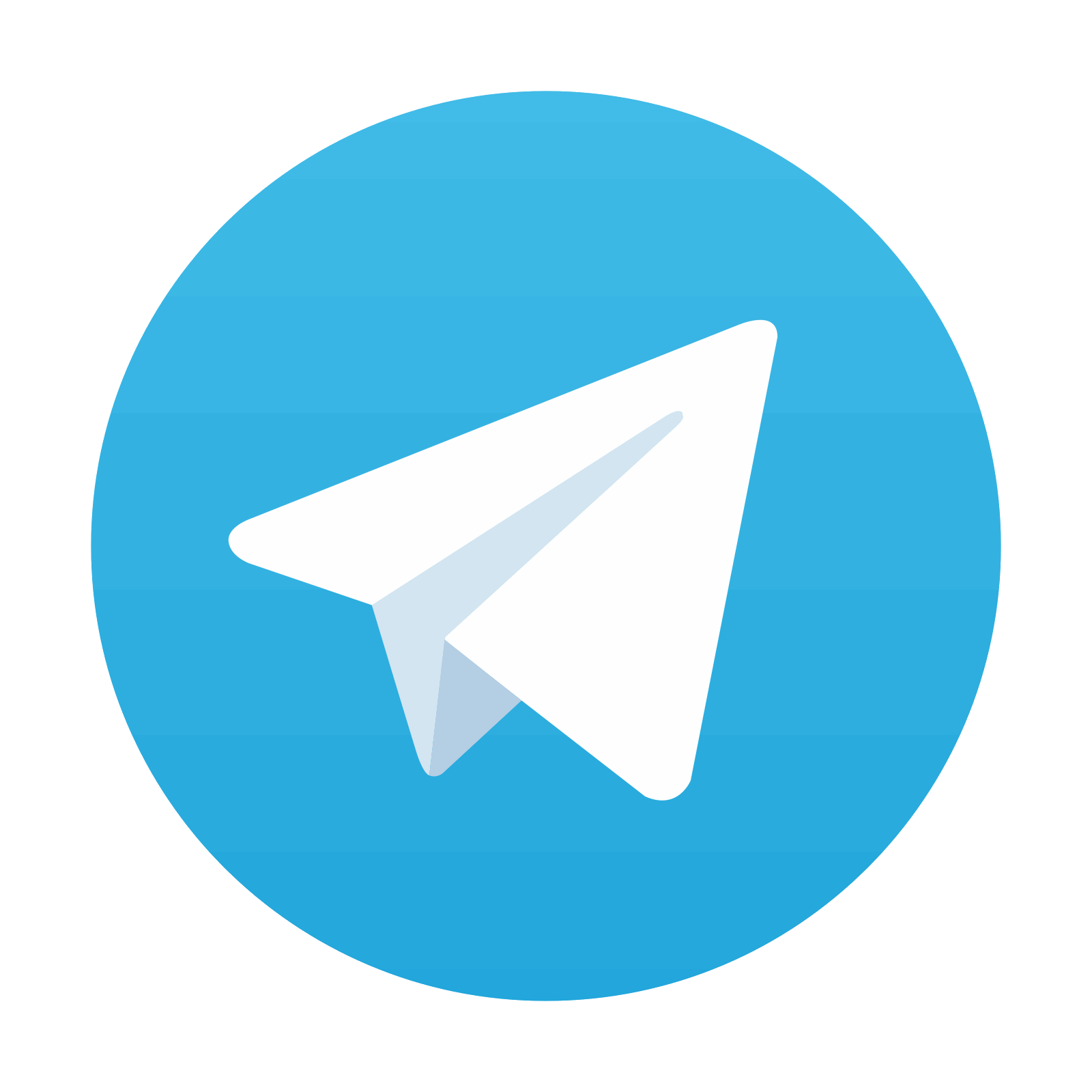
Stay updated, free articles. Join our Telegram channel

Full access? Get Clinical Tree
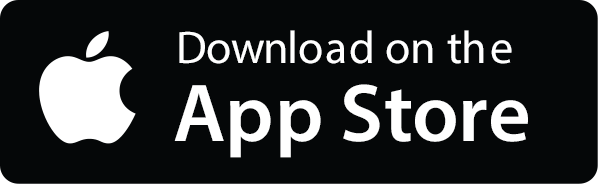
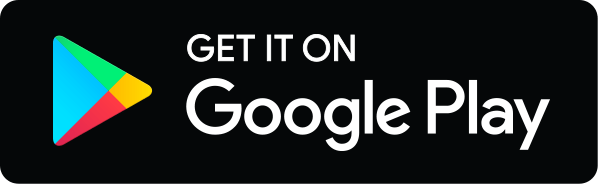
