B. The nature of the connecting hydrocarbon chain is the basis for classifying drugs that produce conduction blockade of nerve impulses as ester local anesthetics or amide local anesthetics (Fig. 10-2). The important differences between ester and amide local anesthetics relate to the site of metabolism and the potential to produce allergic reactions.
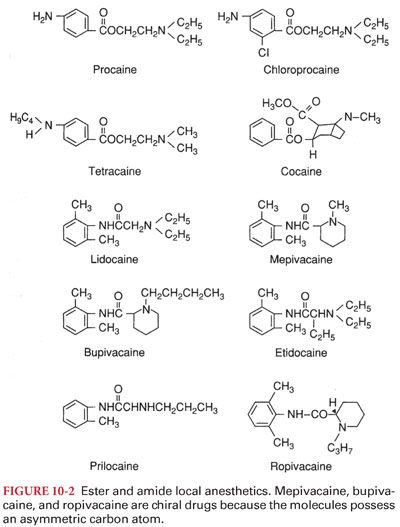
III. Structure-Activity Relationships
A. Modifying the chemical structure of a local anesthetic alters its pharmacologic effects (Table 10-1).
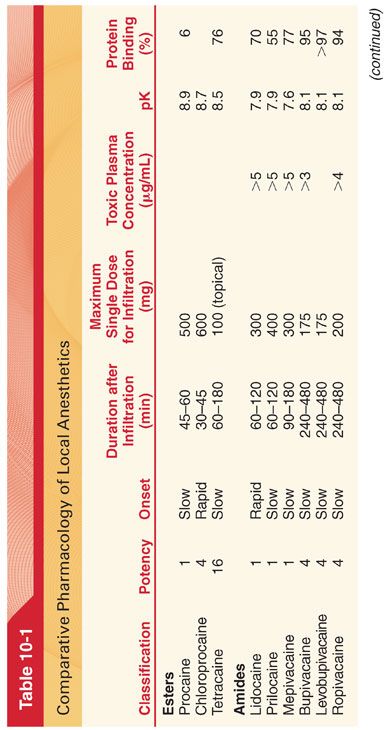
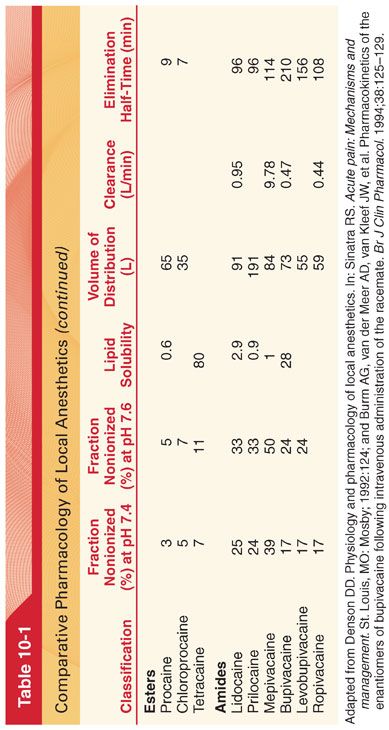
1. Substituting a butyl group for the amine group on the benzene ring of procaine results in tetracaine. Compared with procaine, tetracaine is more lipid soluble, is 10 times more potent, and has a longer duration of action corresponding to a 4- to 5-fold decrease in the rate of metabolism.
2. Halogenation of procaine to chloroprocaine results in a 3- to 4-fold increase in the hydrolysis rate of chloroprocaine by plasma cholinesterase. This rapid hydrolysis rate of chloroprocaine limits the duration of action and systemic toxicity of this local anesthetic.
B. Mepivacaine, bupivacaine, and ropivacaine are characterized as pipecoloxylidides (see Fig. 10-2).
1. Addition of a butyl group to the piperidine nitrogen of mepivacaine results in bupivacaine, which is 35 times more lipid soluble and has a potency and duration of action three to four times that of mepivacaine.
2. Ropivacaine structurally resembles bupivacaine and mepivacaine, with a propyl group on the piperidine nitrogen atom of the molecule.
IV. Racemic Mixtures or Pure Isomers. The pipecoloxylidide local anesthetics (mepivacaine, bupivacaine, ropivacaine, levobupivacaine) are chiral drugs because their molecules possess an asymmetric carbon atom (see Fig. 10-2). As such, these drugs may have a left- (S) or right- (R) handed configuration.
A. The S enantiomers of bupivacaine and mepivacaine appear to be less toxic than the commercially available racemic mixtures of these local anesthetics.
B. In contrast to mepivacaine and bupivacaine, ropivacaine and levobupivacaine have been developed as a pure S enantiomers (less neurotoxicity and cardiotoxicity than the R enantiomers).
V. Liposomal Local Anesthetics. Drugs such as lidocaine, tetracaine, and bupivacaine have been incorporated into liposomes to prolong the duration of action (hemorrhoidectomy, bunionectomy) and decrease toxicity.
VI. Mechanism of Action. Local anesthetics prevent transmission of nerve impulses (conduction blockade) by inhibiting passage of sodium ions through ion-selective sodium channels (a specific receptor for local anesthetic molecules) in nerve membranes (Fig. 10-3). Failure of sodium ion channel permeability to increase slows the rate of depolarization such that threshold potential is not reached and thus an action potential is not propagated (see Fig. 10-3).
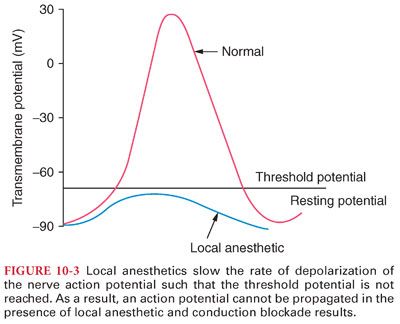
A. Sodium Channels. The sodium channel is a dynamic transmembrane protein consisting of the large sodium-conducting pore (α subunit) and varying numbers of adjacent smaller β subunits.
B. Frequency-Dependent Blockade
1. Sodium ion channels tend to recover from local anesthetic–induced conduction blockade between action potentials and to develop additional conduction blockade each time sodium channels open during an action potential (frequency-dependent blockade).
2. Local anesthetic molecules can gain access to receptors only when sodium channels are in activated-open states and local anesthetic binds more strongly to inactivated state. For this reason, selective conduction blockade of nerve fibers by local anesthetics may be related to the nerve’s characteristic frequencies of activity as well as to its anatomic properties such as diameter.
C. Other Site of Action Targets. In addition to sodium ion channels, local anesthetics block voltage-dependent potassium ion channels.
D. Minimum effective concentration of local anesthetic necessary to produce conduction blockade of nerve impulses is termed the Cm (analogous to the minimum alveolar concentration [MAC] for inhaled anesthetics).
1. Each local anesthetic has a unique Cm, reflecting differing potencies of each drug.
2. The Cm of motor fibers is approximately twice that of sensory fibers; thus, sensory anesthesia may not always be accompanied by skeletal muscle paralysis.
3. Despite an unchanged Cm, less local anesthetic is needed for subarachnoid anesthesia than for epidural anesthesia, reflecting greater access of local anesthetics to unprotected nerves in the subarachnoid space.
E. Differential conduction blockade is illustrated by selective blockade of preganglionic sympathetic nervous system B fibers using low concentrations of local anesthetics. Slightly higher concentrations of local anesthetics interrupt conduction in small C fibers and small- and medium-sized A fibers, with loss of sensation for pain and temperature. Nevertheless, touch, proprioception, and motor function are still present such that the patient will sense pressure but not pain with surgical stimulation (may be misinterpreted as failure of the local anesthetic).
F. Changes during Pregnancy. Increased sensitivity (more rapid onset of conduction blockade) may be present during pregnancy.
VII. Pharmacokinetics (see Table 10-1)
A. Local anesthetics are weak bases that have pK values somewhat above physiologic pH (<50% of the local anesthetic exists in a lipid-soluble nonionized form at physiologic pH). Local anesthetics with pKs nearest to physiologic pH have the most rapid onset of action, reflecting the presence of an optimal ratio of ionized to nonionized drug fraction.
B. Intrinsic vasodilator activity will also influence apparent potency and duration of action (enhanced vasodilator action of lidocaine compared with mepivacaine results in the greater systemic absorption and shorter duration of action of lidocaine).
C. Absorption and Distribution
1. Absorption of a local anesthetic from its site of injection into the systemic circulation is influenced by the site of injection and dosage, use of epinephrine, and pharmacologic characteristics of the drug (Fig. 10-4).
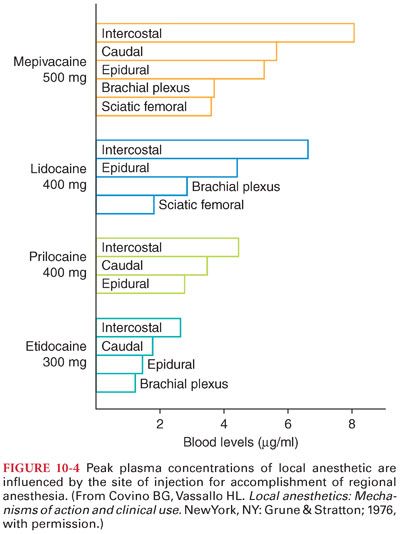
2. The ultimate plasma concentration of a local anesthetic is determined by the rate of tissue distribution and the rate of clearance of the drug.
D. Lung Extraction
1. The lungs are capable of extracting local anesthetics (lidocaine, bupivacaine, prilocaine) from the circulation.
2. This pulmonary extraction will limit the concentration of drug that reaches the systemic circulation for distribution to the coronary and cerebral circulations.
E. Placental Transfer
1. There may be clinically significant transplacental transfer of local anesthetics between the mother and fetus.
2. Bupivacaine, which is highly protein bound (approximately 95%), has an umbilical vein–maternal arterial concentration ratio of about 0.32 compared with a ratio of 0.73 for lidocaine (approximately 70% protein bound).
3. Acidosis in the fetus, which may occur during prolonged labor, can result in accumulation of local anesthetic molecules in the fetus (ion trapping) (Fig. 10-5).
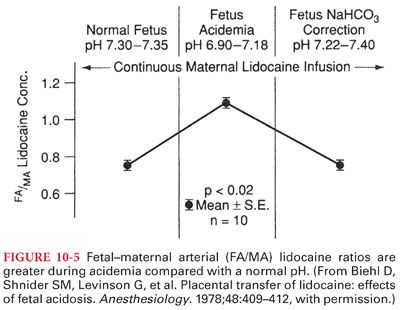
F. Renal Elimination and Clearance
1. The poor water solubility of local anesthetics usually limits renal excretion of unchanged drug to less than 5% (exception is cocaine, of which 10% to 12% of unchanged drug can be recovered in urine).
2. Water-soluble metabolites of local anesthetics, such as para-aminobenzoic acid resulting from metabolism of ester local anesthetics, are readily excreted in urine.
VIII. Metabolism of Amide Local Anesthetics. Amide local anesthetics undergo varying rates of metabolism by microsomal enzymes located primarily in the liver. Prilocaine undergoes the most rapid metabolism; lidocaine and mepivacaine are intermediate; and etidocaine, bupivacaine, and ropivacaine undergo the slowest metabolism among the amide local anesthetics. Compared with that of ester local anesthetics, the metabolism of amide local anesthetics is more complex and slower. This slower metabolism means that sustained increases of the plasma concentrations of amide local anesthetics, and thus systemic toxicity, are more likely than with ester local anesthetics. Furthermore, cumulative drug effects of amide local anesthetics are more likely than with ester local anesthetics.
A. Lidocaine
1. The principal metabolic pathway of lidocaine is oxidative dealkylation in the liver to monoethylglycinexylidide (approximately 80% of the activity of lidocaine for protecting against cardiac dysrhythmias) followed by hydrolysis of this metabolite to xylidide.
2. Xylidide has only approximately 10% of the cardiac antidysrhythmic activity of lidocaine.
B. Prilocaine is an amide local anesthetic that is metabolized to orthotoluidine (an oxidizing compound capable of converting hemoglobin to its oxidized form, methemoglobin, resulting in a potentially life-threatening complication, methemoglobinemia).
1. Methemoglobinemia is readily reversed by the administration of methylene blue, 1 to 2 mg/kg intravenously (IV), over 5 minutes (total dose should not exceed 7 to 8 mg/kg).
2. Prilocaine causes less vasodilation than other local anesthetics and thus can be utilized without epinephrine added to the local anesthetic solution.
C. Mepivacaine
1. Mepivacaine has pharmacologic properties similar to those of lidocaine, although the duration of action of mepivacaine is somewhat longer.
2. In contrast to lidocaine, mepivacaine lacks vasodilator activity (an alternate selection when addition of epinephrine to the local anesthetic solution is not recommended).
D. Bupivacaine
1. Possible pathways for metabolism of bupivacaine include aromatic hydroxylation, N-dealkylation, amide hydrolysis, and conjugation.
2. α1-Acid glycoprotein is the most important plasma protein binding site of bupivacaine, and its concentration is increased in many clinical situations, including postoperative trauma.
E. Ropivacaine is metabolized to 2,6-pipecoloxylidide and 3-hydroxyropivacaine by hepatic cytochrome P-450 enzymes (both metabolites have significantly less local anesthetic potency than ropivacaine).
1. Because only a very small fraction of ropivacaine is excreted unchanged in the urine (about 1%) when the liver is functioning normally, dosage adjustments based on renal function do not seem necessary.
2. Overall, clearance of ropivacaine is higher than that determined for bupivacaine (may offer an advantage over bupivacaine in terms of systemic toxicity).
3. Ropivacaine is highly bound to α1-acid glycoprotein.
F. Dibucaine is an amide local anesthetic known for its ability to inhibit the activity of normal butyrylcholinesterase (plasma cholinesterase) by more than 70%, compared with only approximately 20% inhibition of the activity of atypical enzyme. Laboratory evaluation of patients suspected of having atypical pseudocholinesterase is facilitated by measurement of the degree of enzyme suppression by dibucaine, a test termed the dibucaine number.
IX. Metabolism of Ester Local Anesthetics. Ester local anesthetics undergo hydrolysis by cholinesterase enzyme (exception is cocaine), principally in the plasma and to a lesser extent in the liver. The rate of hydrolysis varies, with chloroprocaine being most rapid, procaine being intermediate, and tetracaine being the slowest. The resulting metabolites are pharmacologically inactive, although para-aminobenzoic acid may be an antigen responsible for subsequent allergic reactions. Systemic toxicity is inversely proportional to the rate of hydrolysis; thus, tetracaine is more likely than chloroprocaine to result in excessive plasma concentrations. Because cerebrospinal fluid contains little to no cholinesterase enzyme, anesthesia produced by subarachnoid placement of tetracaine will persist until the drug has been absorbed into the systemic circulation. Patients with atypical plasma cholinesterase may be at increased risk for developing excess systemic concentrations of an ester local anesthetic due to absent or limited plasma hydrolysis.
A. Procaine is hydrolyzed to para-aminobenzoic acid, which is excreted unchanged in urine.
B. Chloroprocaine
1. Addition of a chlorine atom to the benzene ring of procaine to form chloroprocaine increases by 3.5 times the rate of hydrolysis of the local anesthetic by plasma cholinesterase.
2. Resulting pharmacologically inactive metabolites of chloroprocaine are 2-chloro-aminobenzoic acid and 2-diethylaminoethanol.
C. Tetracaine undergoes hydrolysis by plasma cholinesterase, but the rate is slower than for procaine.
D. Benzocaine is unique among clinically useful local anesthetics because it is a weak acid (pKa 3.5), so that it exists predominantly in the nonionized form at physiologic pH.
1. Benzocaine is ideally suited for topical anesthesia of mucous membranes prior to tracheal intubation, endoscopy, transesophageal echocardiography, and bronchoscopy.
2. Methemoglobinemia is a rare but potentially life-threatening complication following topical application of benzocaine, especially when the dose exceeds 200 to 300 mg.
E. Cocaine is metabolized by plasma and liver cholinesterases to water-soluble metabolites that are excreted in urine. Plasma cholinesterase activity is decreased in parturients, neonates, the elderly, and patients with severe underlying hepatic disease.
1. Cocaine may be present in urine for 24 to 36 hours, depending on the route of administration and cholinesterase activity.
2. Assays for the metabolites of cocaine in urine are useful markers of cocaine use or absorption.
X. Alkalinization of local anesthetic solutions shortens the onset of neural blockade, enhances the depth of sensory and motor blockade, and increases the spread of epidural blockade (adding sodium bicarbonate will speed the onset of peripheral nerve block and epidural block by 3 to 5 minutes).
XI. Use of Vasoconstrictors
A. The duration of action of a local anesthetic is proportional to the time the drug is in contact with nerve fibers (epinephrine [1:200,000 or 5 μg/mL] added to local anesthetic solutions produces vasoconstriction, which limits systemic absorption and maintains the drug concentration in the vicinity of the nerve fibers to be anesthetized) (Fig. 10-6).
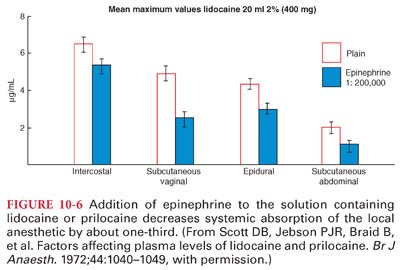
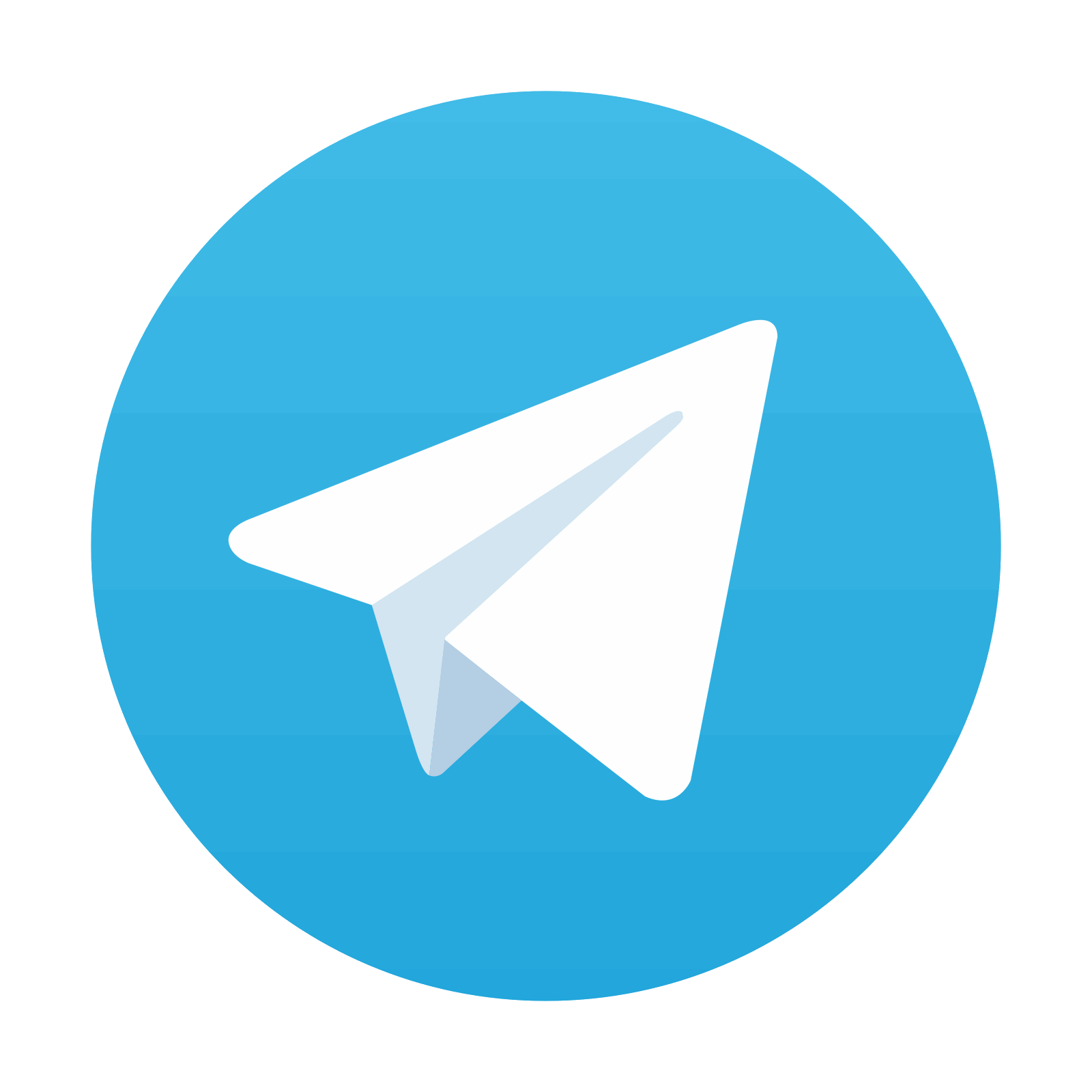
Stay updated, free articles. Join our Telegram channel

Full access? Get Clinical Tree
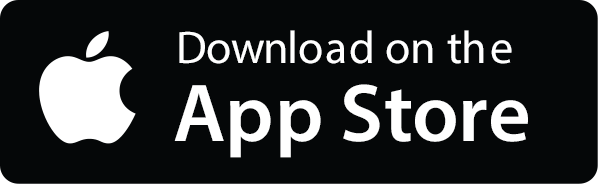
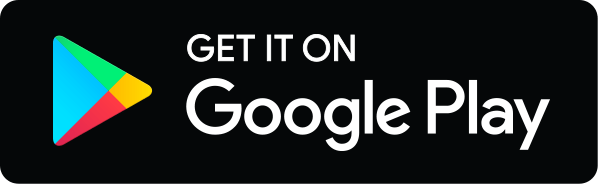