The liver is a very large and complex organ responsible for performing vital tasks that impact all body systems. Its complex functions include metabolism of carbohydrates, lipids, proteins, and bilirubin; detoxification of harmful substances; storage of essential compounds; and excretion of substances to prevent harm. The liver is unique in the sense that it is a relatively resilient organ that can regenerate cells that have been destroyed by some short-term injury or disease or have been removed. However, if the liver is damaged repeatedly over a long period of time, it may undergo irreversible changes that permanently interfere with its essential functions. If the liver becomes completely nonfunctional for any reason, death will occur within approximately 24 hours due to hypoglycemia. This chapter focuses on the normal structure and function of the liver, the pathology associated with it, and the laboratory tests used to aid in the diagnosis of liver disorders.
ANATOMY
Gross Anatomy
Understanding the function and dysfunction of the liver depends on understanding its gross and microscopic structure. The liver is a large and complex organ weighing approximately 1.2 to 1.5 kg in the healthy adult. It is located beneath and attached to the diaphragm, is protected by the lower rib cage, and is held in place by ligamentous attachments. Despite the functional complexity of the liver, it is relatively simple in structure. It is divided unequally into two lobes by the falciform ligament. The right lobe is approximately six times larger than the left lobe. The lobes are functionally insignificant; however, communication flows freely between all areas of the liver (Fig. 25.1).
FIGURE 25.1 Gross anatomy of the liver.
Unlike most organs, which have a single blood supply, the liver is an extremely vascular organ that receives its blood supply from two major sources: the hepatic artery and the portal vein. The hepatic artery, a branch of the aorta, supplies oxygen-rich blood from the heart to the liver and is responsible for providing approximately 25% of the total blood supply to the liver. The portal vein supplies nutrient-rich blood (collected as food is digested) from the digestive tract, and it is responsible for providing approximately 75% of the total blood supply to the liver. The two blood supplies eventually merge into the hepatic sinusoid, which is lined with hepatocytes capable of removing potentially toxic substances from the blood. From the sinusoid, blood flows to the central canal (central vein) of each lobule. It is through the central canal that blood leaves the liver. Approximately 1,500 mL of blood passes through the liver per minute. The liver is drained by a collecting system of veins that empties into the hepatic veins and ultimately into the inferior vena cava (Fig. 25.2).
FIGURE 25.2 Blood supply to the liver.
The excretory system of the liver begins at the bile canaliculi. The bile canaliculi are small spaces between the hepatocytes that form intrahepatic ducts, where excretory products of the cells can drain. The intrahepatic ducts join to form the right and left hepatic ducts, which drain the secretions from the liver. The right and left hepatic ducts merge to form the common hepatic duct, which is eventually joined with the cystic duct of the gallbladder to form the common bile duct. Combined digestive secretions are then expelled into the duodenum (Fig. 25.3).
FIGURE 25.3 Excretory system of the liver.
Microscopic Anatomy
The liver is divided into microscopic units called lobules. The lobules are the functional units of the liver, responsible for all metabolic and excretory functions performed by the liver. Each lobule is roughly a six-sided structure with a centrally located vein (called the central vein) with portal triads at each of the corners. Each portal triad contains a hepatic artery, a portal vein, and a bile duct surrounded by connective tissue. The liver contains two major cell types: hepatocytes and Kupffer cells. The hepatocytes, making up approximately 80% of the volume of the organ, are large cells that radiate outward in plates from the central vein to the periphery of the lobule. These cells perform the major functions associated with the liver and are responsible for the regenerative properties of the liver. Kupffer cells are macrophages that line the sinusoids of the liver and act as active phagocytes capable of engulfing bacteria, debris, toxins, and other substances flowing through the sinusoids (Fig. 25.4).
FIGURE 25.4 Microscopic anatomy of the liver.
BIOCHEMICAL FUNCTIONS
The liver performs four major functions: excretion/secretion, metabolism, detoxification, and storage. The liver is so important that if the liver becomes nonfunctional, death will occur within 24 hours due to hypoglycemia. Although the liver is responsible for a number of functions, this chapter focuses on the four major functions mentioned previously.
Excretory and Secretory
One of the most important functions of the liver is the processing and excretion of endogenous and exogenous substances into the bile or urine such as the major heme waste product, bilirubin. The liver is the only organ that has the capacity to rid the body of heme waste products. Bile is made up of bile acids or salts, bile pigments, cholesterol, and other substances extracted from the blood. The body produces approximately 3 L of bile per day and excretes 1 L of what is produced. Bilirubin is the principal pigment in bile, and it is derived from the breakdown of red blood cells. Approximately 126 days after the emergence from the reticuloendothelial tissue, red blood cells are phagocytized and hemoglobin is released. Hemoglobin is degraded to heme, globin, and iron. The iron is bound by transferrin and is returned to iron stores in the liver or bone marrow for reuse. The globin is degraded to its constituent amino acids, which are reused by the body. The heme portion of hemoglobin is converted to bilirubin in 2 to 3 hours. Bilirubin is bound by albumin and transported to the liver. This form of bilirubin is referred to as unconjugated or indirect bilirubin. Unconjugated bilirubin is insoluble in water and cannot be removed from the body until it has been conjugated by the liver. Once at the liver cell, unconjugated bilirubin flows into the sinusoidal spaces and is released from albumin so it can be picked up by a carrier protein called ligandin. Ligandin, which is located in the hepatocyte, is responsible for transporting unconjugated bilirubin to the endoplasmic reticulum, where it may be rapidly conjugated. The conjugation (esterification) of bilirubin occurs in the presence of the enzyme uridine diphosphate glucuronosyltransferase (UDPGT), which transfers a glucuronic acid molecule to each of the two propionic acid side chains of bilirubin to form bilirubin diglucuronide, also known as conjugated bilirubin. Conjugated bilirubin is water soluble and is able to be secreted from the hepatocyte into the bile canaliculi. Once in the hepatic duct, it combines with secretions from the gallbladder through the cystic duct and is expelled through the common bile duct to the intestines. Intestinal bacteria (especially the bacteria in the lower portion of the intestinal tract) work on conjugated bilirubin to produce mesobilirubin, which is reduced to form mesobilirubinogen and then urobilinogen (a colorless product). Most of the urobilinogen formed (roughly 80%) is oxidized to an orange-colored product called urobilin (stercobilin) and is excreted in the feces. The urobilin or stercobilin is what gives stool its brown color. There are two things that can happen to the remaining 20% of urobilinogen formed. The majority will be absorbed by extrahepatic circulation to be recycled through the liver and re-excreted. The other very small quantity left will enter systemic circulation and will subsequently be filtered by the kidney and excreted in the urine (Fig. 25.5).1
FIGURE 25.5 Metabolism of bilirubin. (Reprinted by permission of Waveland Press, Inc. from Anderson SC, Cockayne S. Clinical Chemistry/Concepts and Applications/2003. Long Grove, IL: Waveland Press, Inc.; 2007. All rights reserved.)
Approximately 200 to 300 mg of bilirubin is produced per day, and it takes a normally functioning liver to process the bilirubin and eliminate it from the body. This, as stated earlier, requires that bilirubin be conjugated. Almost all the bilirubin formed is eliminated in the feces, and a small amount of the colorless product, urobilinogen, is excreted in the urine. The healthy adult has very low levels of total bilirubin (0.2 to 1.0 mg/dL) in the serum, and of this amount, the majority is in the unconjugated form.2
Metabolism
The liver has extensive metabolic capacity; it is responsible for metabolizing many biological compounds including carbohydrates, lipids, and proteins.
The metabolism of carbohydrates is one of the most important functions of the liver. When carbohydrates are ingested and absorbed, the liver can do three things: (1) use the glucose for its own cellular energy requirements, (2) circulate the glucose for use at the peripheral tissues, or (3) store glucose as glycogen (principal storage form of glucose) within the liver itself or within other tissues. The liver is the major player in maintaining stable glucose concentrations due to its ability to store glucose as glycogen (glycogenesis) and degrade glycogen (glycogenolysis) depending on the body’s needs. Under conditions of stress or in a fasting state when there is an increased requirement for glucose, the liver will break down stored glycogen (glycogenolysis), and when the supply of glycogen becomes depleted, the liver will create glucose from nonsugar carbon substrates like pyruvate, lactate, and amino acids (gluconeogenesis).
Lipids are metabolized in the liver under normal circumstances when nutrition is adequate and the demand for glucose is being met. The liver is responsible for metabolizing both lipids and the lipoproteins and is responsible for gathering free fatty acids from the diet, and those produced by the liver itself, and breaking them down to produce acetyl-CoA. Acetyl-CoA can then enter several pathways to form triglycerides, phospholipids, or cholesterol. Despite popular belief, the greatest source of cholesterol in the body comes from what is produced by the liver, not from dietary sources. In fact, approximately 70% of the daily production of cholesterol (roughly 1.5 to 2.0 g) is produced by the liver.3 A more thorough discussion of lipid metabolism may be found in Chapter 15.
Almost all proteins are synthesized by the liver except for the immunoglobulins and adult hemoglobin. The liver plays an essential role in the development of hemoglobin in infants. One of the most important proteins synthesized by the liver is albumin, which carries with it a wide range of important functions. The liver is also responsible for synthesizing the positive and negative acute-phase reactants and coagulation proteins, and it also serves to store a pool of amino acids through protein degradation. The most critical aspect of protein metabolism includes transamination and deamination of amino acids. Transamination (via a transaminase) results in the exchange of an amino group on one acid with a ketone group on another acid. After transamination, deamination degrades them to produce ammonium ions that are consumed in the synthesis of urea and urea is excreted by the kidneys.
Although it would seem logical that any damage to the liver would result in a loss of synthetic and metabolic functions of the liver, that is not the case. The liver must be extensively impaired before it loses its ability to perform these essential functions.
CASE STUDY 25.1
The following laboratory test results were obtained in a patient with severe jaundice, right upper quadrant abdominal pain, fever, and chills (Case Study Table 25.1.1).
CASE STUDY TABLE 25.1.1 Laboratory Results
Questions
1. What is the most likely cause of jaundice in the patient?
Detoxification and Drug Metabolism
The liver serves as a gatekeeper between substances absorbed by the gastrointestinal tract and those released into systemic circulation. Every substance that is absorbed in the gastrointestinal tract must first pass through the liver; this is referred to as first pass. This is an important function of the liver because it can allow important substances to reach the systemic circulation and can serve as a barrier to prevent toxic or harmful substances from reaching systemic circulation. The body has two mechanisms for detoxification of foreign materials (drugs and poisons) and metabolic products (bilirubin and ammonia). Either it may bind the material reversibly to inactivate the compound or it may chemically modify the compound so it can be excreted in its chemically modified form. One of the most important functions of the liver is the drug-metabolizing system of the liver. This system is responsible for the detoxification of many drugs through oxidation, reduction, hydrolysis, hydroxylation, carboxylation, and demethylation. Many of these take place in the liver microsomes via the cytochrome P-450 isoenzymes.
LIVER FUNCTION ALTERATIONS DURING DISEASE
Jaundice
The word jaundice comes from the French word jaune, which means “yellow,” and it is one of the oldest known pathologic conditions reported, having been described by Hippocratic physicians.4 Jaundice, or icterus, is used to describe the yellow discoloration of the skin, eyes, and mucous membranes most often resulting from the retention of bilirubin; however, it may also occur due to the retention of other substances. Although the upper limit of normal for total bilirubin is 1.0 to 1.5 mg/dL, jaundice is usually not noticeable to the naked eye (known as overt jaundice) until bilirubin levels reach 3.0 to 5.0 mg/dL. Although the terms jaundice and icterus are used interchangeably, the term icterus is most commonly used in the clinical laboratory to refer to a serum or plasma sample with a yellow discoloration due to an elevated bilirubin level. Jaundice is most commonly classified based on the site of the disorder: prehepatic jaundice, hepatic jaundice, and posthepatic jaundice. This classification is important because knowing the classification of jaundice will aid health care providers in formulating an appropriate treatment or management plan. Prehepatic and posthepatic jaundice, as the names imply, are caused by abnormalities outside the liver, either before, as in “prehepatic,” or after, as in “posthepatic.” In these conditions, liver function is normal or it may be functioning at a maximum to compensate for abnormalities occurring elsewhere. This is not the case with hepatic jaundice, where the jaundice is due to a problem with the liver itself—an intrinsic liver defect or disease.
Prehepatic jaundice occurs when the problem causing the jaundice occurs prior to liver metabolism. It is most commonly caused by an increased amount of bilirubin being presented to the liver such as that seen in acute and chronic hemolytic anemias. Hemolytic anemia causes an increased amount of red blood cell destruction and the subsequent release of increased amounts of bilirubin presented to the liver for processing. The liver responds by functioning at maximum capacity; therefore, people with prehepatic jaundice rarely have bilirubin levels that exceed 5.0 mg/dL because the liver is capable of handling the overload. This type of jaundice may also be referred to as unconjugated hyperbilirubinemia because the fraction of bilirubin increased in people with prehepatic jaundice is the unconjugated fraction. This fraction of bilirubin (unconjugated bilirubin) is not water soluble, is bound to albumin, is not filtered by the kidneys, and is not seen in the urine.
Hepatic jaundice occurs when the primary problem causing the jaundice resides in the liver (intrinsic liver defect or disease). This intrinsic liver defect or disease can be due to disorders of bilirubin metabolism and transport defects (Crigler-Najjar syndrome, Dubin-Johnson syndrome, Gilbert’s disease, and neonatal physiologic jaundice of the newborn) or due to diseases resulting in hepatocellular injury or destruction. Gilbert’s disease, Crigler-Najjar syndrome, and physiologic jaundice of the newborn are hepatic causes of jaundice that result in elevations in unconjugated bilirubin. Conditions such as Dubin-Johnson and Rotor’s syndrome are hepatic causes of jaundice that result in elevations in conjugated bilirubin.
Gilbert’s syndrome, first described in the early twentieth century, is a benign autosomal recessive hereditary disorder that affects approximately 5% of the US population.5 Gilbert’s syndrome results from a genetic mutation in the UGT1A1 gene that produces the enzyme uridine diphosphate glucuronosyltransferase, one of the enzymes important for bilirubin metabolism. The UGT1A1 gene is located on chromosome 2, and other mutations of this same gene produce Crigler-Najjar syndrome, a more severe and dangerous form of hyperbilirubinemia.6
Of the many causes of jaundice, Gilbert’s syndrome is the most common cause, and interestingly, it carries no morbidity or mortality in the majority of those affected and carries generally no clinical consequences. It is characterized by intermittent unconjugated hyperbilirubinemia, underlying liver disease due to a defective conjugation system in the absence of hemolysis. The hyperbilirubinemia usually manifests during adolescence or early adulthood. Total serum bilirubin usually fluctuates between 1.5 and 3.0 mg/dL, and it rarely exceeds 4.5 mg/dL. The molecular basis of Gilbert’s syndrome (in whites) is related to the UDPGT superfamily, which is responsible for encoding enzymes that catalyze the conjugation of bilirubin. The UGT1A1 (the hepatic 1A1 isoform of UDPGT) contributes substantially to the process of conjugating bilirubin. The UGT1A1 promoter contains the sequence (TA)6TAA. The insertion of an extra TA in the sequence, as seen in Gilbert’s syndrome, reduces the expression of the UGT1A1 gene to 20% to 30% of normal values. That is, the liver’s conjugation system in Gilbert’s syndrome is working at approximately 30% of normal.7,8
Crigler-Najjar syndrome was first described by Crigler and Najjar in 1952 as a syndrome of chronic nonhemolytic unconjugated hyperbilirubinemia.9 Crigler-Najjar syndrome, like Gilbert’s syndrome, is an inherited disorder of bilirubin metabolism resulting from a molecular defect within the gene involved with bilirubin conjugation. Crigler-Najjar syndrome may be divided into two types: type 1, where there is a complete absence of enzymatic bilirubin conjugation, and type II, where there is a mutation causing a severe deficiency of the enzyme responsible for bilirubin conjugation. Unlike Gilbert’s syndrome, Crigler-Najjar syndrome is rare and is a more serious disorder that may result in death.10
While Gilbert’s disease and Crigler-Najjar syndrome are characterized as primarily unconjugated hyperbilirubinemias, Dubin-Johnson syndrome and Rotor’s syndrome are characterized as conjugated hyperbilirubinemias. Dubin-Johnson syndrome is a rare autosomal recessive inherited disorder caused by a deficiency of the canalicular multidrug resistance/multispecific organic anionic transporter protein (MDR2/cMOAT). In other words, the liver’s ability to uptake and conjugate bilirubin is functional; however, the removal of conjugated bilirubin from the liver cell and the excretion into the bile are defective. This results in accumulation of conjugated and, to some extent, unconjugated bilirubin in the blood, leading to hyperbilirubinemia and bilirubinuria. Dubin-Johnson is a condition that is obstructive in nature, so much of the conjugated bilirubin circulates bound to albumin. This type of bilirubin (conjugated bilirubin bound to albumin) is referred to as delta bilirubin. An increase in delta bilirubin poses a problem in laboratory evaluation because the delta bilirubin fraction reacts as conjugated bilirubin in the laboratory method to measure conjugated or direct bilirubin. A distinguishing feature of Dubin-Johnson syndrome is the appearance of dark-stained granules (thought to be pigmented lysosomes) on a liver biopsy sample. Usually, the total bilirubin concentration remains between 2 and 5 mg/dL, with more than 50% due to the conjugated fraction. This syndrome is relatively mild in nature with an excellent prognosis. People with Dubin-Johnson syndrome have a normal life expectancy, so no treatment is necessary.11,12
Rotor’s syndrome is clinically similar to Dubin-Johnson syndrome, but the defect causing Rotor’s syndrome is not known.13 It is hypothesized to be due to a reduction in the concentration or activity of intracellular binding proteins such as ligandin. Unlike in Dubin-Johnson syndrome, a liver biopsy does not show dark pigmented granules. Rotor’s syndrome is seen less commonly than Dubin-Johnson syndrome; it is a relatively benign condition and carries an excellent prognosis, and therefore, treatment is not warranted. However, an accurate diagnosis is required to aid in distinguishing it from more serious liver diseases that require treatment.
Physiologic jaundice of the newborn is a result of a deficiency in the enzyme UDPGT, one of the last liver functions to be activated in prenatal life since bilirubin processing is handled by the mother of the fetus. Premature infants may be born without UDPGT, the enzyme responsible for bilirubin conjugation. This deficiency results in the rapid buildup of unconjugated bilirubin, which can be life threatening. When unconjugated bilirubin builds up in the neonate, it cannot be processed and it is deposited in the nuclei of brain and degenerate nerve cells, causing kernicterus. Kernicterus often results in cell damage and death in the newborn, and this condition will continue until UDPGT is produced. Infants with this type of jaundice are usually treated with phototherapy to destroy the bilirubin as it passes through the capillaries of the skin. Since it was first described in 1958, phototherapy has been effectively used as a relatively inexpensive and noninvasive method of treating neonatal hyperbilirubinemia through photo oxidation. Conventional phototherapy lowers serum bilirubin levels by using halogen or fluorescent lights to transform bilirubin into water-soluble isomers that can be eliminated without conjugation in the liver. During phototherapy, the baby is undressed so that as much of the skin as possible is exposed to the light, his/her eyes are covered to protect the nerve layer at the back of the eye (retina) from the bright light, and the bilirubin levels are measured at least once a day. Alternatively, fiberoptic phototherapy is also available via a blanket, called a bili-blanket, that consists of a pad of woven fibers used to transport light from a light source to the baby’s back. The light generated through the bili-blanket breaks down the bilirubin through photo oxidation. The dose of phototherapy is a key factor in how quickly it works and the dose is determined by the wavelength of the light, the intensity of the light (irradiance), the distance between the light and the baby, and the body surface area exposed to the light. Commercially available phototherapy systems include those that deliver light via fluorescent bulbs, halogen quartz lamps, light-emitting diodes, and fiberoptic mattresses. In extreme cases of hyperbilirubinemia, an exchange transfusion may be used as the second-line treatment when phototherapy fails. An exchange transfusion involves removing aliquots of blood and replacing it with donor blood in order to remove abnormal blood components and circulating toxins while maintaining adequate circulating blood volume. Because hyperbilirubinemia is so serious in newborns, bilirubin levels are carefully and frequently monitored so the dangerously high levels of unconjugated bilirubin (approximately 20 mg/dL) can be detected and treated appropriately.14
Posthepatic jaundice results from biliary obstructive disease, usually from physical obstructions (gallstones or tumors) that prevent the flow of conjugated bilirubin into the bile canaliculi. Since the liver cell itself is functioning, bilirubin is effectively conjugated; however, it is unable to be properly excreted from the liver. Since bile is not being brought to the intestines, stool loses its source of normal pigmentation and becomes clay-colored. The laboratory findings for bilirubin and its metabolites in the abovementioned types of jaundice are summarized in Table 25.1. Mechanisms of hyperbilirubinemia may be found in Figure 25.6.
TABLE 25.1 Changes in Concentration of Bilirubin in Those with Jaundice
Adapted from Table 27.3 of Kaplan LA, Pesce AJ, Kazmierczak S. Clinical Chemistry: Theory, Analysis, Correlation. 4th ed. St. Louis, MO: Mosby; 2003.
FIGURE 25.6 Mechanisms of hyperbilirubinemia. (A) Normal bilirubin metabolism, (B) hemolytic jaundice, (C) Gilbert’s disease, (D) physiologic jaundice, (E) Dubin-Johnson syndrome, and (F) intrahepatic or extrahepatic obstruction. (Adapted from Kaplan LA, Pesce AJ, Kazmierczak S. Clinical Chemistry: Theory, Analysis, Correlation. 4th ed. St. Louis, MO: Mosby; 2003:449.)
Cirrhosis
Cirrhosis is a clinical condition in which scar tissue replaces normal, healthy liver tissue. As the scar tissue replaces the normal liver tissue, it blocks the flow of blood through the organ and prevents the liver from functioning properly. Cirrhosis rarely causes signs and symptoms in its early stages, but as liver function deteriorates, the signs and symptoms appear, including fatigue, nausea, unintended weight loss, jaundice, bleeding from the gastrointestinal tract, intense itching, and swelling in the legs and abdomen. Although some patients with cirrhosis may have prolonged survival, they generally have a poor prognosis. Cirrhosis was the twelfth leading cause of death by disease in 2013, killing just over 36,400 people.15 In the United States, the most common cause of cirrhosis is chronic alcoholism. Other causes of cirrhosis include chronic hepatitis B (HBV), C (HCV), and D virus (HDV) infection, autoimmune hepatitis, inherited disorders (e.g., α1-antitrypsin deficiency, Wilson’s disease, hemochromatosis, and galactosemia), nonalcoholic steatohepatitis, blocked bile ducts, drugs, toxins, and infections.
Liver damage from cirrhosis cannot easily be reversed, but treatment can stop or delay further progression of the disorder. Treatment depends on the cause of cirrhosis and any complications a person is experiencing. For example, cirrhosis caused by alcohol abuse is treated by abstaining from alcohol. Treatment for hepatitis-related cirrhosis involves medications used to treat the different types of hepatitis, such as interferon for viral hepatitis and corticosteroids for autoimmune hepatitis.
Tumors
Cancers of the liver are classified as primary or metastatic. Primary liver cancer is cancer that begins in the liver cells while metastatic cancer occurs when tumors from other parts of the body spread (metastasize) to the liver. Metastatic liver cancer is much more common than primary liver cancer; 90% to 95% of all hepatic malignancies are classified as metastatic. Cancers that commonly spread to the liver include colon, lung, and breast cancer. Tumors of the liver may also be classified as benign or malignant. The common benign tumors of the liver include hepatocellular adenoma (a condition occurring almost exclusively in females of childbearing age) and hemangiomas (masses of blood vessels with no known etiology). Malignant tumors of the liver include hepatocellular carcinoma (HCC) (also known as hepatocarcinoma, and hepatoma) and bile duct carcinoma. Of those, HCC is the most common malignant tumor of the liver. Hepatoblastoma is an uncommon hepatic malignancy of children.
HCC has become increasingly important in the United States. Approximately 85% of the new cases of this liver cancer occur in developing countries, with the highest incidence of HCC reported in regions where HBV is endemic such as Southeast Asia and sub-Saharan Africa. Approximately 500,000 people are diagnosed annually with HCC worldwide, of which 28,000 new cases and 20,000 deaths were reported in the United States in 2012.16,17 It is estimated that in the United States, chronic HBV and HCV infections account for approximately 30% and 40% of cases of HCC.18
Approximately 80% of cases worldwide are attributable to HBV and HCV; however, the mechanism by which the infection leads to HCC is not well established. While surgical resection of HCC is sometimes possible, currently, orthotopic liver transplantation in people with HCC with underlying cirrhosis who meet the Milan criteria (single tumor ≤5 cm in size or ≤3 tumors each ≤3 cm in size, and no macrovascular invasion) is also available for HCC. The estimated 4-year survival rate is 85% and the recurrence-free survival rate is 92%.19
Whether primary or metastatic, any malignant tumor in the liver is a serious finding and carries a poor prognosis, with survival times measured in months.
Reye’s Syndrome
Reye’s syndrome is a term used to describe a group of disorders caused by infectious, metabolic, toxic, or drug-induced disease found almost exclusively in children, although adult cases of Reye’s syndrome have been reported.20 Although the precise cause of Reye’s syndrome is unknown, it is often preceded by a viral syndrome such as varicella, gastroenteritis, or an upper respiratory tract infection such as influenza.21, 22, 23 Although not described as the precise cause of Reye’s syndrome, studies have demonstrated a strong epidemiologic association between the ingestion of aspirin during a viral syndrome and the subsequent development of Reye’s syndrome.24,25 As a result, the Centers for Disease Control and Prevention (CDC) cautioned physicians and parents to avoid salicylate use in children with a viral syndrome, and the U.S. Surgeon General mandated that a warning label be added to all aspirin-containing medications, beginning in 1986.26,27 Reye’s syndrome is an acute illness characterized by noninflammatory encephalopathy and fatty degeneration of the liver, with a clinical presentation of profuse vomiting accompanied with varying degrees of neurologic impairment such as fluctuating personality changes and deterioration in consciousness. The encephalopathy is characterized by a progression from mild confusion (stage 1) through progressive loss of neurologic function to loss of brain stem reflexes (stage 5). The degeneration of the liver is characterized by a mild hyperbilirubinemia and threefold increases in ammonia and the aminotransferases (aspartate aminotransferase [AST] and alanine aminotransferase [ALT]). Without treatment, rapid clinical deterioration leading to death may occur.28,29
Drug- and Alcohol-Related Disorders
Drug-induced liver disease is a major problem in the United States, accounting for one-third to one-half of all reported cases of acute liver failure. The liver is a primary target organ for adverse drug reactions because it plays a central role in drug metabolism. Many drugs are known to cause liver damage, ranging from very mild transient forms to fulminant liver failure. Drugs can cause liver injury by a variety of mechanisms, but the most common mechanism of toxicity is via an immune-mediated injury to the hepatocytes.30 In this type of mechanism, the drug induces an adverse immune response directed against the liver itself and results in hepatic and/or cholestatic disease.31
Of all the drugs associated with hepatic toxicity, the most important is ethanol. In very small amounts, ethanol causes very mild, transient, and unnoticed injury to the liver; however, with heavier and prolonged consumption, it can lead to alcoholic cirrhosis. While the exact amount of alcohol needed to cause cirrhosis is unknown, a small minority of people with alcoholism develop this condition.32 Approximately 90% of the alcohol absorbed from the stomach and small intestines is transported to the liver for metabolism. Within the liver, the elimination of alcohol requires the enzymes alcohol dehydrogenase and acetaldehyde dehydrogenase to convert alcohol to acetaldehyde and subsequently to acetate. The acetate can then be oxidized to water and carbon dioxide, or it may enter the citric acid cycle.
Long-term excessive consumption of alcohol can result in a spectrum of liver abnormalities that may range from alcoholic fatty liver with inflammation (steatohepatitis) to scar tissue formation, as in hepatic fibrosis, to the destruction of normal liver structure seen in hepatic cirrhosis. Alcohol-induced liver injury may be categorized into three stages: alcoholic fatty liver, alcoholic hepatitis, and alcoholic cirrhosis. The risk for the development of cirrhosis increases proportionally with the consumption of more than 30 g (the equivalent of 3 to 4 drinks) of alcohol per day, with the highest degree of risk seen with the consumption of greater than 120 g (the equivalent of 12 to 16 drinks) per day.33
Alcoholic fatty liver represents the mildest category where very few changes in liver function are measurable. This stage is characterized by slight elevations in AST, ALT, and γ-glutamyltransferase (GGT), and on biopsy, fatty infiltrates are noted in the vacuoles of the liver. This stage tends to affect young to middle-aged people with a history of moderate alcohol consumption. A complete recovery within 1 month is seen when the drug is removed. Alcoholic hepatitis presents with common signs and symptoms including fever, ascites, proximal muscle loss, and far more laboratory evidence of liver damage such as moderately elevated AST, ALT, GGT, and alkaline phosphatase (ALP) and elevations in total bilirubin greater than 5 mg/dL. The elevations in AST are more than twice the upper reference of normal but rarely exceed 300 IU/mL. The elevations in ALT are comparatively lower than AST, resulting in an AST/ALT ratio (De Ritis ratio) greater than 2. Serum proteins, especially albumin, are decreased and the international normalized ratio (INR, which is a ratio of coagulation time in the patient compared with a normal coagulation time) is elevated. Prognosis is dependent on the type and severity of damage to the liver, and when serum creatinine levels begin to increase, it is a threatening sign, which may precede the onset of hepatorenal syndrome and death.34 There are a variety of scoring systems that have been used to assess the severity of alcoholic hepatitis and to guide treatment including the Maddrey’s discriminant function,35 the Glasgow score,36 and the Model for End-Stage Liver Disease (MELD) score.37 All three scoring systems use bilirubin, INR, creatinine, age, white cell counts, blood urea nitrogen, and albumin levels to stage and guide treatment. The last and most severe stage is alcoholic cirrhosis. The prognosis associated with alcoholic cirrhosis is dependent on the nature and severity of associated conditions such as a gastrointestinal bleeding or ascites; however, the 5-year survival rate is 60% in those who abstain from alcohol and 30% in those who continue to drink. This condition appears to be more common in males than in females, and the symptoms tend to be nonspecific and include weight loss, weakness, hepatomegaly, splenomegaly, jaundice, ascites, fever, malnutrition, and edema. Laboratory abnormalities include increased liver function tests (AST, ALT, GGT, ALP, and total bilirubin), decreased albumin, and a prolonged prothrombin time. A liver biopsy is the only method by which a definitive diagnosis may be made.38
Other drugs, including tranquilizers, some antibiotics, antineoplastic agents, lipid-lowering medication, and anti-inflammatory drugs, may cause liver injury ranging from mild damage to massive hepatic failure and cirrhosis. One of the most common drugs associated with serious hepatic injury is acetaminophen. When acetaminophen is taken in massive doses, it is virtually certain to produce fatal hepatic necrosis unless rapid treatment is initiated.
CASE STUDY 25.2
The following laboratory test results were found in a patient with mild weight loss and nausea and vomiting, who later developed jaundice and an enlarged liver (Case Study Table 25.2.1)
CASE STUDY TABLE 25.2.1 Laboratory Results
Questions
1. What disease process is most likely in this patient?
ASSESSMENT OF LIVER FUNCTION/LIVER FUNCTION TESTS
Bilirubin
Analysis of Bilirubin: A Brief Review
The reaction of bilirubin with a diazotized sulfanilic acid solution to form a colored product was first described by Ehrlich in 1883 using urine samples. Since then, this type of reaction (bilirubin with a diazotized sulfanilic acid solution) has been referred to as the classic diazo reaction, a reaction on which all commonly used methods today are based. In 1913, van den Bergh found that the diazo reaction may be applied to serum samples but only in the presence of an accelerator (solubilizer). However, this methodology had errors associated with it. It was not until 1937 that Malloy and Evelyn developed the first clinically useful methodology for the quantitation of bilirubin in serum samples using the classic diazo reaction with a 50% methanol solution as an accelerator. In 1938, Jendrassik and Grof described a method using the diazo reaction with caffeine–benzoate–acetate as an accelerator. Today, all commonly used methods for measuring bilirubin and its fractions are modifications of the technique described by Malloy and Evelyn. Total bilirubin and conjugated bilirubin (direct bilirubin) are measured and unconjugated bilirubin (indirect bilirubin) is determined by subtracting conjugated bilirubin from total bilirubin (see Fig. 25.7).
FIGURE 25.7 Methods to measure different fractions of bilirubin.
Bilirubin has also been quantified using bilirubinometry in the neonatal population. This methodology is only useful in the neonatal population because of the presence of carotenoid compounds in adult serum that causes strong positive interference in the adult population. Bilirubinometry involves the measurement of reflected light from the skin using two wavelengths that provide a numerical index based on spectral reflectance. Newer-generation bilirubinometers use microspectrophotometers that determine the optical densities of bilirubin, hemoglobin, and melanin in the subcutaneous layers of the infant’s skin. Mathematical isolation of hemoglobin and melanin allows measurement of the optical density created by bilirubin.39
When using the several methods described earlier, two of the three fractions of bilirubin were identified: conjugated (direct) and unconjugated (indirect) bilirubin. Unconjugated (indirect) bilirubin is a nonpolar and water-insoluble substance that is found in plasma bound to albumin. Because of these characteristics, unconjugated bilirubin will only react with the diazotized sulfanilic acid solution (diazo reagent) in the presence of an accelerator (solubilizer). Conjugated (direct) bilirubin is a polar and water-soluble compound that is found in plasma in the free state (not bound to any protein). This type of bilirubin will react with the diazotized sulfanilic acid solution directly (without an accelerator). Thus, conjugated and unconjugated bilirubin fractions have historically been differentiated by solubility of the fractions. Conjugated bilirubin reacts in the absence of an accelerator, whereas unconjugated bilirubin requires an accelerator. While for many years bilirubin results were reported as direct and indirect, this terminology is now outdated. Direct and indirect bilirubin results should be reported as conjugated and unconjugated, respectively.40
The third fraction of bilirubin is referred to as “delta” bilirubin. Delta bilirubin is conjugated bilirubin that is covalently bound to albumin. This fraction of bilirubin is seen only when there is significant hepatic obstruction. Because the molecule is attached to albumin, it is too large to be filtered by the glomerulus and excreted in the urine. This fraction of bilirubin, when present, will react in most laboratory methods as conjugated bilirubin. Thus, total bilirubin is made up of three fractions: conjugated, unconjugated, and delta bilirubin. The three fractions together are known as total bilirubin.
Specimen Collection and Storage
Total bilirubin methods using a diazotized sulfanilic acid solution may be performed on either serum or plasma. Serum, however, is preferred for the Malloy-Evelyn procedure because the addition of the alcohol in the analysis can precipitate proteins and cause interference with the method. A fasting sample is preferred as the presence of lipemia will increase measured bilirubin concentrations. Hemolyzed samples should be avoided as they may decrease the reaction of bilirubin with the diazo reagent. Bilirubin is very sensitive to and is destroyed by light; therefore, specimens should be protected from light. If left unprotected from light, bilirubin values may reduce by 30% to 50% per hour. If serum or plasma is separated from the cells and stored in the dark, it is stable for 2 days at room temperature, 1 week at 4°C, and indefinitely at −20°C.41
METHODS
There is no preferred reference method or standardization of bilirubin analysis; however, the American Association for Clinical Chemistry and the National Bureau of Standards have published a candidate reference method for total bilirubin, a modified Jendrassik-Grof procedure using caffeine–benzoate as a solubilizer.42 Because they both have acceptable precision and are adapted to many automated instruments, the Jendrassik-Grof or Malloy-Evelyn procedure is the most frequently used method to measure bilirubin. The Jendrassik-Grof method is slightly more complex, but it has the following advantages over the Malloy-Evelyn method:
- Not affected by pH changes
- Insensitive to a 50-fold variation in protein concentration of the sample
- Maintains optical sensitivity even at low bilirubin concentrations
- Has minimal turbidity and a relatively constant serum blank
- Is not affected by hemoglobin up to 750 mg/dL
Because this chapter does not allow for a detailed description of all previously mentioned bilirubin test methodologies, only the most widely used principles for measuring bilirubin in the adult and pediatric populations are covered.43, 44, 45
Malloy-Evelyn Procedure
Bilirubin pigments in serum or plasma are reacted with a diazo reagent. The diazotized sulfanilic acid reacts at the central methylene carbon of bilirubin to split the molecule forming two molecules of azobilirubin. This method is typically performed at pH 1.2 where the azobilirubin produced is red-purple in color with a maximal absorption of 560 nm. The most commonly used accelerator to solubilize unconjugated bilirubin is methanol, although other chemicals have been used.46
Jendrassik-Grof Method for Total and Conjugated Bilirubin Determination
Principle
Bilirubin pigments in serum or plasma are reacted with a diazo reagent (sulfanilic acid in hydrochloric acid and sodium nitrite), resulting in the production of the purple product azobilirubin. The product azobilirubin may be measured spectrophotometrically. The individual fractions of bilirubin are determined by taking two aliquots of sample and reacting one aliquot with the diazo reagent only and the other aliquot with the diazo reagent and an accelerator (caffeine–benzoate). The addition of caffeine–benzoate will solubilize the water-insoluble fraction of bilirubin and will yield a total bilirubin value (all fractions). The reaction without the accelerator will yield conjugated bilirubin only. After a short period of time, the reaction of the aliquots with the diazo reagent is terminated by the addition of ascorbic acid. The ascorbic acid destroys the excess diazo reagent. The solution is then alkalinized using an alkaline tartrate solution, which shifts the absorbance spectrum of the azobilirubin to a more intense blue color that is less subject to interfering substances in the sample. The final blue product is measured at 600 nm, with the intensity of color produced directly proportional to bilirubin concentration. Indirect (unconjugated) bilirubin may be calculated by subtracting the conjugated bilirubin concentration from the total bilirubin concentration.
Comments and Sources of Error
Instruments should be frequently standardized to maintain reliable bilirubin results, and careful preparation of bilirubin standards is critical as these are subject to deterioration from exposure to light. Hemolysis and lipemia should be avoided as they will alter bilirubin concentrations. Serious loss of bilirubin occurs after exposure to fluorescent and indirect and direct sunlight; therefore, it is imperative that exposure of samples and standards to light be kept to a minimum. Specimens and standards should be refrigerated in the dark until testing can be performed.
Reference Range
See Table 25.2.
TABLE 25.2 Reference Ranges for Bilirubin in Adults and Infants
Urobilinogen in Urine and Feces
Urobilinogen is a colorless end product of bilirubin metabolism that is oxidized by intestinal bacteria to the brown pigment urobilin. In the normal individual, part of the urobilinogen is excreted in feces, and the remainder is reabsorbed into the portal blood and returned to the liver. A small portion that is not taken up by the hepatocytes is excreted by the kidney as urobilinogen. Increased levels of urinary urobilinogen are found in hemolytic disease and in defective liver cell function, such as that seen in hepatitis. Absence of urobilinogen from the urine and stool is most often seen with complete biliary obstruction. Fecal urobilinogen is also decreased in biliary obstruction, as well as in HCC.47
Most quantitative methods for urobilinogen are based on a reaction first described by Ehrlich in 1901: the reaction of urobilinogen with p-dimethylaminobenzaldehyde (Ehrlich’s reagent) to form a red color. Many modifications of this procedure have been made over the years to improve specificity. However, because the modifications did not completely recover urobilinogen from the urine, most laboratories use the less laborious, more rapid, semiquantitative method described next.
Determination of Urine Urobilinogen (Semiquantitative)
Principle
Urobilinogen reacts with p-dimethylaminobenzaldehyde (Ehrlich’s reagent) to form a red color, which is then measured spectrophotometrically. Ascorbic acid is added as a reducing agent to maintain urobilinogen in the reduced state. The use of saturated sodium acetate stops the reaction and minimizes the combination of other chromogens with the Ehrlich’s reagent.48
Specimen
A fresh 2-hour urine specimen is collected. This specimen should be kept cool and protected from light.
Comments and Sources of Error
1. The results of this test are reported in Ehrlich units rather than in milligrams of urobilinogen because substances other than urobilinogen account for some of the final color development.
2. Compounds, other than urobilinogen, that may be present in the urine and react with Ehrlich’s reagent include porphobilinogen, sulfonamides, procaine, and 5-hydroxyindoleacetic acid. Bilirubin will form a green color and, therefore, must be removed, as previously described.
3. Fresh urine is necessary, and the test must be performed without delay to prevent oxidation of urobilinogen to urobilin. Similarly, the spectrophotometric readings should be made within 5 minutes after color production because the urobilinogen–aldehyde color slowly decreases in intensity.
Reference Range
Urine urobilinogen, 0.1 to 1.0 Ehrlich units every 2 hours or 0.5 to 4.0 Ehrlich units per day (0.86 to 8 mmol/d); 1 Ehrlich unit is equivalent to approximately 1 mg of urobilinogen.
Fecal Urobilinogen
Visual inspection of the feces is usually sufficient to detect decreased urobilinogen. However, the semiquantitative determination of fecal urobilinogen is available and involves the same principle described earlier for the urine. It is carried out in an aqueous extract of fresh feces, and any urobilin present is reduced to urobilinogen by treatment with alkaline ferrous hydroxide before Ehrlich’s reagent is added. A range of 75 to 275 Ehrlich units per 100 g of fresh feces or 75 to 400 Ehrlich units per 24-hour specimen is considered a normal reference range.48
Serum Bile Acids
Serum bile acid analysis is rarely performed because the methods required are very complex. These involve extraction with organic solvents, partition chromatography, gas chromatography–mass spectrometry, spectrophotometry, ultraviolet light absorption, fluorescence, radioimmunoassay, and enzyme immunoassay (EIA) methods. Although serum bile acid levels are elevated in liver disease, the total concentration is extremely variable and adds no diagnostic value to other tests of liver function. The variability of the type of bile acids present in serum, together with their existence in different conjugated forms, suggests that more relevant information of liver dysfunction may be gained by examining patterns of individual bile acids and their state of conjugation. For example, it has been suggested that the ratio of the trihydroxy to dihydroxy bile acids in serum will differentiate patients with obstructive jaundice from those with hepatocellular injury and that the diagnosis of primary biliary cirrhosis and extrahepatic cholestasis can be made on the basis of the ratio of the cholic to chenodeoxycholic acids. However, the high cost of these tests, the time required to do them, and the current controversy concerning their clinical usefulness render this approach unsatisfactory for routine use.49,50
Enzymes
Liver enzymes play an important role in the assessment of liver function because injury to the liver resulting in cytolysis or necrosis will cause the release of enzymes into circulation. Enzymes also play an important role in differentiating hepatocellular (functional) from obstructive (mechanical) liver disease, which is an important clinical distinction because failure to identify an obstruction will result in liver failure if the obstruction is not rapidly treated. Although many enzymes have been identified as useful in the assessment of liver function, the most clinically useful include the aminotransferases (ALT and AST), the phosphatases (ALP and 5′-neucleotidase), GGT, and lactate dehydrogenase (LD).
The methods used to measure these enzymes, the normal reference ranges, and other general aspects of enzymology are discussed in Chapter 13. Discussion in this chapter focuses on the characteristic changes in serum enzyme levels seen in various hepatic disorders. It is important to note that the diagnosis of disease depends on a combination of patient history, physical examination, laboratory testing, and sometimes radiologic studies and biopsy, and therefore, abnormalities in liver enzymes alone are not diagnostic in and of themselves.51,52
Aminotransferases
The two most common aminotransferases measured in the clinical laboratory are AST (formerly referred to as serum glutamic oxaloacetic transaminase [SGOT]) and ALT (formerly referred to as serum glutamic pyruvic transaminase [SGPT]). The aminotransferases are responsible for catalyzing the conversion of aspartate and alanine to oxaloacetate and pyruvate, respectively. In the absence of acute necrosis or ischemia of other organs, these enzymes are most useful in the detection of hepatocellular (functional) damage to the liver. ALT is found mainly in the liver (lesser amounts in skeletal muscle and kidney), whereas AST is widely distributed in equal amounts in the heart, skeletal muscle, and liver, making ALT a more “liver-specific” marker than AST. Regardless, the serum activity of both transaminases rises rapidly in almost all diseases of the liver and may remain elevated for up to 2 to 6 weeks. The highest levels of AST and ALT are found in acute conditions such as viral hepatitis, drug- and toxin-induced liver necrosis, and hepatic ischemia. The increase in ALT activity is usually greater than that for AST. Only moderate increases are found in less severe conditions. AST and ALT are found to be normal or only mildly elevated in cases of obstructive liver damage. Because AST and ALT are present in other tissues besides the liver, elevations in these enzymes may be a result of other organ dysfunction or failure such as acute myocardial infarction, renal infarction, progressive muscular dystrophy, and those conditions that result in secondary liver disease such as infectious mononucleosis, diabetic ketoacidosis, and hyperthyroidism. It is often helpful to conduct serial determinations of aminotransferases when following the course of a patient with acute or chronic hepatitis, and caution should be used in interpreting abnormal levels because serum transaminases may actually decrease in some patients with severe acute hepatitis, owing to the exhaustive release of hepatocellular enzymes.51,52
Phosphatases
Alkaline Phosphatase
The ALP family of enzymes are zinc metalloenzymes that are widely distributed in all tissues; however, highest activity is seen in the liver, bone, intestine, kidney, and placenta. The clinical utility of ALP lies in its ability to differentiate hepatobiliary disease from osteogenic bone disease. In the liver, the enzyme is localized to the microvilli of the bile canaliculi, and therefore, it serves as a marker of extrahepatic biliary obstruction, such as a stone in the common bile duct, or in intrahepatic cholestasis, such as drug cholestasis or primary biliary cirrhosis. ALP is found in very high concentrations in cases of extrahepatic obstruction with only slight to moderate increases seen in those with hepatocellular disorders such as hepatitis and cirrhosis. Because bone is also a source of ALP, it may be elevated in bone-related disorders such as Paget’s disease, bony metastases, diseases associated with an increase in osteoblastic activity, and rapid bone growth during puberty. ALP is also found elevated in pregnancy due to its release from the placenta, where it may remain elevated up to several weeks postdelivery. As a result, interpretation of ALP concentrations is difficult because enzyme activity of ALP can increase in the absence of liver damage.51,52
5′-Nucleotidase
5′-Nucleotidase (5NT) is a phosphatase that is responsible for catalyzing the hydrolysis of nucleoside-5′-phosphate esters. Although 5NT is found in a wide variety of cells, serum levels become significantly elevated in hepatobiliary disease. There is no bone source of 5NT, so it is useful in differentiating ALP elevations due to the liver from other conditions where ALP may be seen in increased concentrations (bone diseases, pregnancy, and childhood growth). Levels of both 5NT and ALP are elevated in liver disease, whereas in primary bone disease, ALP level is elevated, but the 5NT level is usually normal or only slightly elevated. This enzyme is much more sensitive to metastatic liver disease than is ALP because, unlike ALP, its level is not significantly elevated in other conditions, such as in pregnancy or during childhood. In addition, some increase in enzyme activity may be noted after abdominal surgery.51, 52, 53, 54
γ-Glutamyltransferase
GGT is a membrane-localized enzyme found in high concentrations in the kidney, liver, pancreas, intestine, and prostate but not in bone. Similar to the clinical utility of 5NT (see earlier), GGT plays a role in differentiating the cause of elevated levels of ALP as the highest levels of GGT are seen in biliary obstruction. GGT is a hepatic microsomal enzyme; therefore, ingestion of alcohol or certain drugs (barbiturates, tricyclic antidepressants, and anticonvulsants) elevates GGT. It is a sensitive test for cholestasis caused by chronic alcohol or drug ingestion. Measurement of this enzyme is also useful if jaundice is absent for the confirmation of hepatic neoplasms.51, 52, 53, 54, 55
Lactate Dehydrogenase
LD is an enzyme with a very wide distribution throughout the body. It is released into circulation when cells of the body are damaged or destroyed, serving as a general, nonspecific marker of cellular injury. Moderate elevations of total serum LD levels are common in acute viral hepatitis and in cirrhosis, whereas biliary tract disease may produce only slight elevations. High serum levels may be found in metastatic carcinoma of the liver. As a result of its wide distribution, LD measurements provide no additional clinical information above that which is provided by the previously mentioned enzymes. However, fractionation of LD into its five tissue-specific isoenzymes may give useful information about the site of origin of the LD elevation.
Tests Measuring Hepatic Synthetic Ability
A healthy functioning liver is required for the synthesis of serum proteins (except the immunoglobulins). The measurement of serum proteins, therefore, can be used to assess the synthetic ability of the liver. Although these tests are not sensitive to minimal liver damage, they may be useful in quantitating the severity of hepatic dysfunction.
A decreased serum albumin may be a result of decreased liver protein synthesis, and the albumin level correlates well with the severity of functional impairment and is found more often in chronic rather than in acute liver disease. The serum α-globulins also tend to decrease with chronic liver disease. However, a low or absent α-globulin suggests α-antitrypsin deficiency as the cause of the chronic liver disease. Serum γ-globulin levels are transiently increased in acute liver disease and remain elevated in chronic liver disease. The highest elevations are found in chronic active hepatitis and postnecrotic cirrhosis. In particular, immunoglobulin G (IgG) and IgM levels are more consistently elevated in chronic active hepatitis; IgM, in primary biliary cirrhosis; and IgA, in alcoholic cirrhosis.
Prothrombin time is commonly increased in liver disease because the liver is unable to manufacture adequate amounts of clotting factor or because the disruption of bile flow results in inadequate absorption of vitamin K from the intestine. However, a prothrombin time is not routinely used to aid in the diagnosis of liver disease. Rather, serial measurements of prothrombin times may be useful in following the progression of disease and the assessment of the risk of bleeding. A marked prolongation of the prothrombin time indicates severe diffuse liver disease and a poor prognosis.
Tests Measuring Nitrogen Metabolism
The liver plays a major role in removing ammonia from the bloodstream and converting it to urea so that it can be removed by the kidneys. A plasma ammonia level, therefore, is a reflection of the liver’s ability to perform this conversion. In liver failure, ammonia and other toxins increase in the bloodstream and may ultimately cause hepatic coma. In this condition, the patient becomes increasingly disoriented and gradually lapses into unconsciousness. The cause of hepatic coma is not fully known, although ammonia is presumed to play a major role. However, the correlation between blood ammonia levels and the severity of the hepatic coma is poor. Therefore, ammonia levels are most useful when multiple measurements are made over time.
The most common laboratory determination of ammonia concentrations is based on the following reaction:

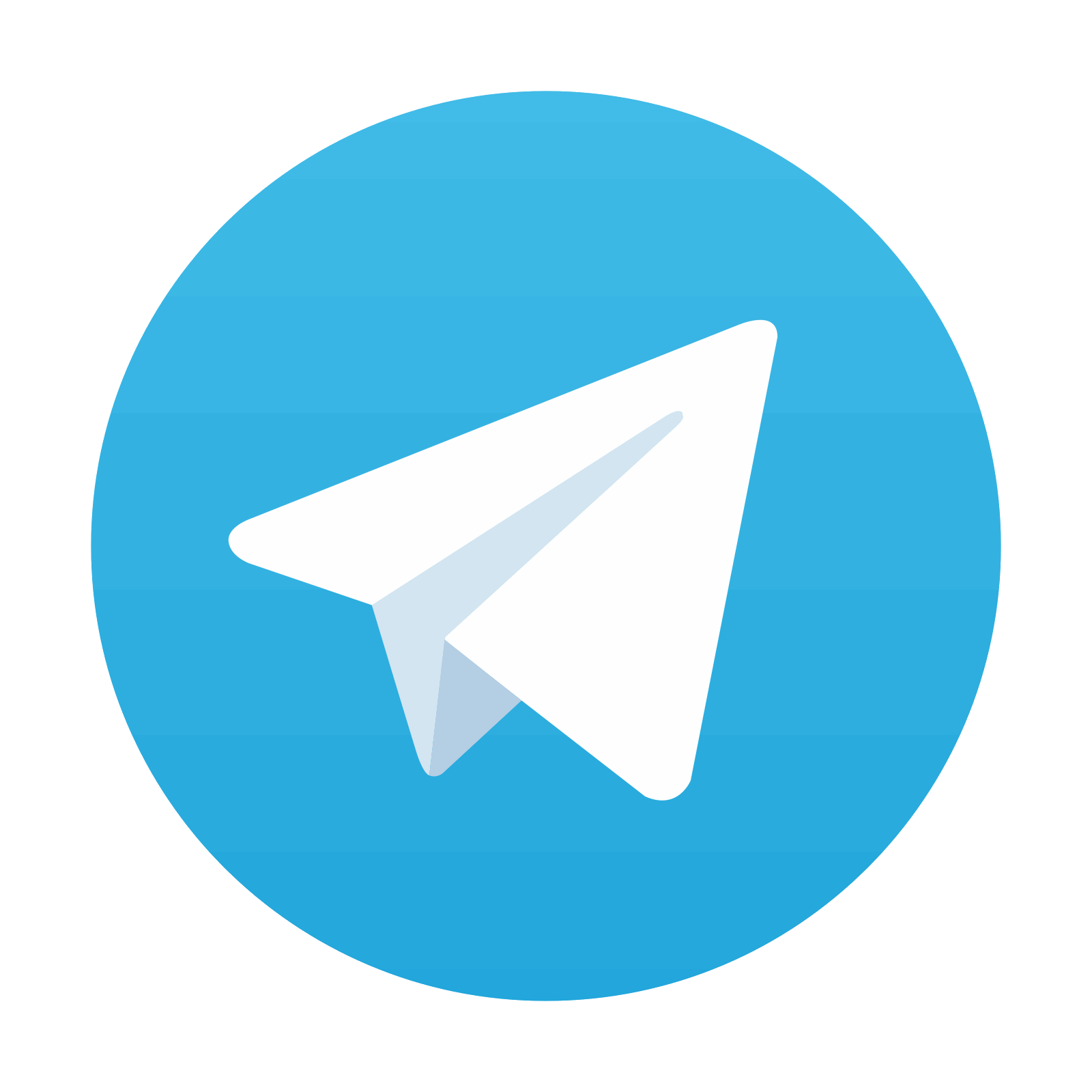
Stay updated, free articles. Join our Telegram channel

Full access? Get Clinical Tree
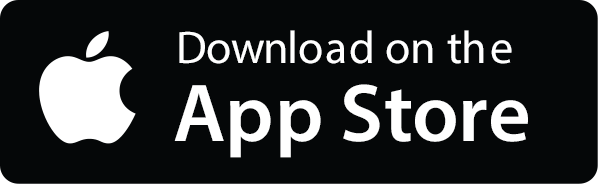
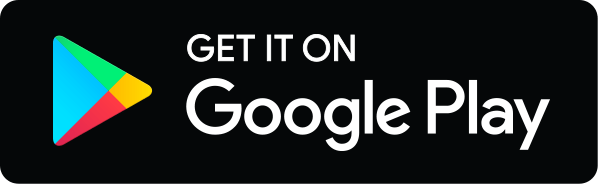